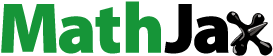
ABSTRACT
Utilization of waste dregs, e.g. Huaimi dregs, is an important issue. Huaimi is a traditional Chinese medicine and 20,000 tons of dregs are annually produced. This study optimized the production of L-lactic acid through solid-state fermentation of Huaimi dregs using uniform and orthogonal experimental designs. Among three tested parameters, fermentation time and substrate ratio (wheat bran:Huaimi dregs) were found to have a significant effect on the production of lactic acid. Using fermentation time of 41.6 h, substrate ratio of 4.26:1, inoculum size of 6.1% and fermentation temperature of 28 °C, 1 kg Huaimi dregs could produce 286.6 mg lactic acid.
KEYWORDS:
Introduction
Lactic acid (molecular formula: C3H6O3, semi-structural formula: CH3CHOHCOOH) is a common, natural, organic acid. Its scientific name is α-hydroxypropanoic acid and it has a relative molecular weight of 90.08. The lactic acid molecule has one asymmetric carbon atom that causes optical isomerism. This results in lactic acid having two configurations, the left-handed D-type and the right-handed L-type. Lactic acid and its derivatives are widely used in the food, pharmaceutical, chemical, leather and textile industry [Citation1,Citation2].
Lactic acid is usually prepared through fermentation, chemical synthesis or the enzymatic method. When the microbial fermentation method is used, the production of L-lactic acid can be implemented by carefully choosing specific microbial strains and culture conditions. According to the microbial type, the fermentation methods can be divided into bacterial fermentation and Rhizopus fermentation [Citation3–6]. Chemical synthesis can also be used to produce lactic acid. There are a variety of chemical synthesis methods; the most feasible one is acetaldehyde cyanohydrin synthesis [Citation7]. Unfortunately, the synthesis involves racemic DL-lactic acid [Citation8]. The enzymatic synthesis method includes enzymatic conversions from 2-chloropropionic acid and pyruvate [Citation9,Citation10]. Although the enzymatic synthesis of lactic acid can produce the desired optical isomers, the process is complicated and needs further exploration regarding its industrial applications [Citation11,Citation12].
The demand for lactic acid in China is increasing, as the fields that utilize it and its derivatives have expanded [Citation13–15]. The beer industry alone uses more than 3000 tons of lactic acid every year, and the demand for lactic acid by the food and pharmaceutical industries is on the increase [Citation16–19]. L-lactic acid is primarily used in the production of polylactic acid by polymerization, which has good compatibility and biodegradability, and has been widely used in the packaging, pharmaceutical and textile industries [Citation20–24]. The low-cost high-efficient production of lactic acid can use lignocellulose as raw materials, as in patent CN102586348A [Citation25]. Under this patent, first, the cellulose in lignocellulose is enzymatically hydrolysed to obtain sugar-containing hydrolysates, and then high temperature fermentation is conducted to produce lactic acid. Under patent CN1843152 [Citation26], cellulase and amylase are added to food waste to produce lactic acid by open fermentation.
Lactic acid could possibly be produced from the dregs that result from commercial production of traditional Chinese medicine products. In the past, Chinese herbal medicines used to be produced through simple processes; however, with the development and progress of science and technology, the processing of Chinese herbal medicine has become more complex. Previously, Chinese herbal medicine was administered through powders, soups and pastes, but currently the medicines can be administered orally or through injections and capsules. The modern production process results in the production of a large amount of dregs [Citation27]. Currently, the dregs are disposed of through accumulation, incineration and landfills, which not only pollute the environment, but also are a waste of resources [Citation28]. Modern society values energy conservation, emission reduction, low-carbon footprints and environmentally friendly processes [Citation29,Citation30].
Huaimi is a commonly used traditional Chinese medicine that is derived from the dried buds of Sophora japonicum L. from the Fabaceae family [Citation31]. The richest active ingredient is rutin, which serves as the primary indicator of Huaimi quality. Under the action of an enzyme or acid, rutin is hydrolysed into quercetin [Citation32–33]. The pharmacological effects of Huaimi include blood cooling, stopping bleeding, liver clearing and stomach fire draining. In recent years, Chinese and international scholars have extensively studied its effects, and have found that the active ingredient has anti-cancer, anti-platelet aggregation, analgesic, anti-bacterial, anti-viral, anti-aging, anti-inflammatory, anti-allergic, anti-free-radical formation and antioxidant effects, and that it can improve cardiac circulation, promote detoxification, lower blood lipids content, soften blood vessels and invigorate the kidneys [Citation34–37]. Previous studies that have looked at upcycling Huaimi dregs include patent CN103892045A [Citation38], which reports the production of animal feed from Huaimi solid dregs using Aspergillus niger.
In the current fermentation technology for lactic acid production, biomass sugars and starchy substances such as potato and corn are the main raw material. The main problem is the high cost. Using cheaper raw materials is an important way to reduce costs. Thus, Chinese medicine waste residue as a renewable biomass resource is the ideal raw materials for production of lactic acid. To the best of our knowledge, related research has not been reported so far.
Since the 1990s, plant extracts have been increasingly attracting close attention from the pharmaceutical and food industry. The consumption of plant extracts from the United States’ market amounted to about $ 2.8 billion in 2005. These extracts are mainly from a single plant, for example, extracts from ginkgo, green tea, angelica, ginseng and other herbs. There are about 800 kinds of botanical products registered as medicines in Germany. There is a variety of standardized medicinal plant extracts in Europe, such as: extracts from purple daisies, short palms, elderberry etc., which are widely used in medicinal products, healthy food, cosmetics and other industries [Citation39]. Therefore, the solid residue remaining from these industrial-scale extractions is a common problem.
The aim of this study was to perform step-wise optimization of physico-chemical parameters such as inoculum size (%), fermentation time (h) and substrate ratio for mixed-substrate solid-state fermentation of traditional Chinese medicine residues, Huaimi dregs, by uniform and orthogonal factorial design followed by response surface methodology (RSM) for the production of L-lactic acid.
Materials and methods
Micro-organism and culture conditions
Rhizopus oryzae, accession number 3.819, was obtained from the China General Microbiological Culture Collection Center (CGMCC). The primary culture of R. oryzae was prepared by inoculation of the R. oryzae strain onto potato dextrose agar solid medium (200 g/L potato, 20 g/L dextrose, 15–20 g/L agar, pH 6.8–7.0) and incubation at 28 °C for 5–7 days. The spore suspension was prepared by gentle pipetting under strict sterile conditions and the number of shed spores was determined. The concentration of the R. oryzae spore suspension was adjusted to (0.10–1.00) × 107 spores/mL.
Biomass materials and pretreatment
Wheat bran (WB) (1–3 mm in size) was collected in its original state from local pet markets at Yuquan Road, Beijing. Huaimi dregs were obtained by students from the Biochemical Engineering College of Beijing Union University via the preparation of rutin from Huaimi. Huaimi buds were boiled for 30 min in water that was 2–3 times their weight and then boiled two additional times, each time for 15 min. Then the buds were filtered while still hot and the solids were rinsed twice in hot tap water, filtered until they were dry and finally air-dried at room temperature.
Lactic acid production by solid-state fermentation
Lactic acid was produced through solid-state fermentation in 250 mL Erlenmeyer flasks containing the Huaimi dregs medium, which was prepared by adding 12–14 g of a mixture of WB and the pretreated Huaimi dregs (DH), with a WB:DH ratio of 7:1–1:6, 0.6% corn flour, 0.6% ammonium chloride, 0.4% potassium dihydrogen phosphate and sterile water (in an amount that was equal to the mixture). The dregs of Huaimi medium (pH of 6.8–7.0) was autoclaved at 121 °C for 20 min.
The R. oryzae primary cell culture was added to the Huaimi dregs medium at a volume of 5–30 mL culture/100 g Huaimi dregs, fermented at 20–30 °C for 24–96 h, and shaken uniformly every 12 h. After fermentation, the solid dregs culture was rinsed with distilled water to obtain the filtrate. Milk of lime was added to the filtrate to adjust the pH to 9.5–10, and the clarified supernatant was concentrated under reduced pressure at a temperature of 45–50 °C. The concentrated solution was stored in a refrigerator at 3–5 °C in order to grow calcium lactate crystals. After the liquid was filtered off, the crystals were placed in a drying oven at 100 °C for 2–2.5 h in order to dry into powder. The calcium lactate powder was dissolved by heating and stirring, and then acidified with concentrated sulphuric acid, with the same mole fraction as the calcium in the crystals. Finally, the crude lactic acid was obtained by filtration. The experiments were repeated three times.
Lactic acid determination
An SBA-50B biosensor analyser (Institute of Biology, Shandong Academy of Sciences) was used to perform the analysis. An immobilized biologically active substance was used as the catalyst, which was able to react with a particular substrate. This system is not sensitive to colour and turbidity, and was highly accurate, with a relative error of about 1%. The system was calibrated with an injection of 25 μL standard solution at a concentration of 1.5 mg/mL. Then, a sample of 12 g fermentation was rinsed with 50 mL of distilled water and filtered to collect the supernatant, which was diluted for the test (sample concentration range: 0–100 mg/mL). A sample of 25 μL diluted supernatant was injected into the biosensor analyser, and the lactic acid concentration was displayed; then it was multiplied by the dilution factor to give the actual sample concentration.
Model building
When an experimental design reaches the optimized conditions, a nonlinear model is generally used for regression. In most cases, the following second-order model is appropriate:
Based on our prior experience and preliminary experiments, we found that lactic acid yield was not only closely related to temperature, but also to inoculum size (%), fermentation time (h) and substrate ratio (bran:dregs).
Therefore, a ternary quadratic polynomial regression was proposed to evaluate the impact of each independent variable on the amount of lactic acid. Among the variables, y is the amount of lactic acid; xi (i = 1, 2, 3) represents the independent variables which might affect the amount of lactic acid; m is the number of factors; x1 is inoculum size (%); x2 is fermentation time (h); and x3 is the substrate ratio; β0 is a bias term; βi (i = 1, 2, 3) represents the linear effect of xi; βii (i = 1, 2, 3) represents the secondary effect of xi; and βij (i, j = 1, 2, 3; i < j) represents the interaction between xi and xj [Citation40].
The established mathematical model was
Experimental design
Uniform design
Three factors were considered in the experimental design: inoculum size (%), fermentation time (h) and substrate ratio. First, a mixed level uniform design was conducted for these three factors. This was a uniform design U12 (6 × 4 × 3), with six levels of inoculum size (4, 6, 8, 10, 12 and 14); four levels of fermentation time (24, 36, 48 and 60); and three levels of substrate ratio (WB:DH) (3, 4 and 5). Then, an experimental design that contained 12 runs was used to investigate the amount of lactic acid. The specific design is shown in .
Table 1. Uniform experimental design.
Statistical Program for Social Sciences (SPSS) version 17.0 (SPSS Inc., Chicago, IL, USA) was used to perform the regression analysis. The quality of the polynomial model was represented by the coefficient of determination (R2). The statistical significance was measured by the F-test, and the significance of the regression coefficients was determined by the t-test.
MATLAB version 7.9.00 529 (MathWorks Inc., USA) was used for further optimal screening.
Orthogonal design
An orthogonal experimental design was performed for the inoculum size (%), fermentation time (h) and substrate ratio. The design used the orthogonal table L8(27), with two inoculum size levels (4 and 5); two fermentation time levels (36 and 48 h); and two substrate ratio levels (4 and 5). The specific design is shown in .
Table 2. Orthogonal experimental design.
Response surface methodology (RSM)
RSM was used for evaluation of the main and interaction effects of the three factors found to be significant from the experiments, while keeping the other factors at their central level. When using RSM, we assume that lactic acid is a second-order function of three parameters: inoculum size (%), fermentation time (h) and substrate ratio. In this paper, RSM was used to analyse the main response and corresponding response. SPSS version 17.0 (SPSS Inc., Chicago, IL, USA) returns a regression function given our experimental data, and by using MATLAB it is easy to get the optimal condition to the function. Next, one of the variables was fixed at its medium such that RSM can draw the indifference curve of the other two variables. For instance, the substrate ratio was assigned a medium value, and MATLAB could output a diagram of indifference curve of the function.
Univariate analysis
Univariate analysis was used to study how one particular variable can influence the experiment while other factors are fixed. The above mathematical method and results were computed using MATHLAB.
Results and discussion
Determination of optimal temperature
Lactic acid production through fermentation can utilize starch and cellulose as raw materials, in addition to monosaccharides like glucose and lactose. Lactic acid production through fermentation is very popular because of the wide range of raw materials that can be used and the low production costs. The resulting products have high optical purity and high safety level [Citation4,Citation6]. It is important to conduct research and offer ideas for sustainable handling of Chinese medicine dregs. Studying the use of traditional Chinese medicine dregs as raw materials for lactic acid production could not only offer new sources of biomass materials for lactic acid production, but also could provide a new strategy for industrial upgrading and structural adjustment of Chinese medicine industry.
Temperature is known to affect the lactic acid yield. The R. oryzae strain from CGMCC used in this study has an optimal temperature of approximately 28 °C, as reported by CGMCC based on a large number of experiments.
To ensure the reliability of the experimental results, we conducted a verification experiment using each of the following temperatures: 26, 27, 28, 29 and 30 °C, an inoculation amount of 5%, fermentation time of 54 h and substrate ratio (WB:DH) of 4.
The results shown in confirmed that the maximum amount of lactic acid was obtained at 28 °C, and the yield was significantly higher than at the other tested temperatures. Therefore, the provider's recommendation of 28 °C was adopted for the following experiments.
Table 3. Effect of temperature on lactic acid yield.
Uniform and orthogonal experiments
The results from the uniform experimental design are shown in . The data from the 12 runs of the uniform design were used for regression analysis. However, the results were not favourable – the adjusted R2 was only 0.64. Therefore, it was necessary to perform further experiments including an orthogonal experimental design.
Table 4. Results from the uniform experimental design.
Relatively, good results were obtained from the uniform design. The tested inoculum size of 4% gave better results than 5%; fermentation time of 36 h was better than 48 h; and a substrate ratio (WB:DH) of 5 was observed to be more suitable than a ratio of 4. The results from the orthogonal design based on the uniform design results are shown in . K is the average of one certain level in the certain factor, R is extreme value which is the largest K minus the smallest K.
Table 5. Results from the orthogonal experimental design.
According to the orthogonal analysis, the optimal conditions were: inoculation amount of 5%, fermentation time of 40 h and substrate ratio (WB:DH) of 4, with a corresponding maximum lactic acid yield of 0.2598 mg/g.
Regression analysis
SPSS was used to perform regression analysis based on 20 runs of the experimental data. The resulting regression equation was y = −0.140231 + 0.085833x1 + 0.030413x2 + 0.373069x3 − 0.00318x12 − 0.00026x22 − 0.03491x32 − 0.00065x1x2 − 0.0047x1x3 − 0.00113x2x3.
The adjusted R2 was 0.988, indicating high goodness of fit and a strong explanatory power. The F-value obtained from the regression analysis was 175.14, which is much larger than the critical value of F0.05(9, 10) = 3.03. The p-value (p = 0.000) was small, indicating that the overall equation was statistically significant. All of the regression coefficients were analysed by t-test, and the corresponding p-values (p ≤ 0.014) indicated that the regression coefficients are significant.
Using MATLAB, the predicted optimum parameters were found to be: inoculum amount of 6.1%, fermentation time of 41.6 h and substrate ratio (WB:DH) of 4.26. The results of the regression model were consistent with the results of the orthogonal analysis. The results of the regression model were better than those of the orthogonal analysis; the former could give a predicted maximum lactic acid yield of 0.2866 mg/g using the above conditions.
Optimization of the parameters using response surface design
RSM was used to perform a modelling analysis of the multiple variables that affect the response in order to optimize the response. RSM can draw a continuous function, whereas the orthogonal design only gives discrete points. In this study, the purpose of using RSM was to visually evaluate the main effects and interactions of the three factors. Combined with the economic input, it could intuitively provide the optimal conditions.
If the substrate ratio was taken as the centre point, the amount of lactic acid with respect to inoculum amount and fermentation time satisfied the equation y = −0.44661 + 0.065813x1 + 0.0256x2 − 0.00318x12 − 0.00026x22 − 0.00065x1x2, and the corresponding response surface is shown in .
Figure 1. Response surface plot of the lactic acid concentration with respect to inoculum size and fermentation time using the substrate ratio as a centre point.
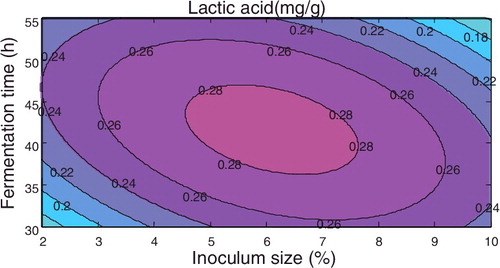
If fermentation time was taken as the centre point, the amount of lactic acid with respect to inoculum amount and substrate ratio satisfied the equation y = −0.58696 + 0.058796x1 + 0.326084x3 − 0.00318x12 − 0.03491x32 − 0.0047x1x3, and the corresponding response surface is shown in .
Figure 2. Response surface plot of the lactic acid concentration with respect to inoculum size and substrate ratio using the fermentation time as a centre point.
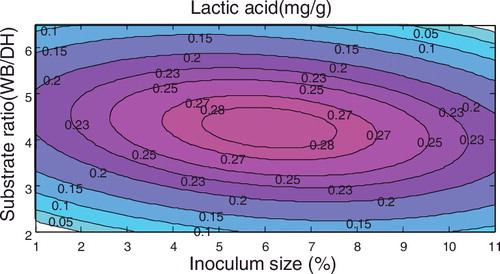
If the inoculum amount was taken as the centre point, the amount of lactic acid with respect to fermentation time and substrate ratio satisfied the equation y = −0.99728 + 0.026451x2 + 0.34421x3 − 0.00026x22 − 0.03491x32 − 0.00113x2x3, and the corresponding response surface is shown in .
Univariate analysis
In the univariate analysis, all other factors were set to the corresponding centre values. According to the obtained results, the equation for the amount of lactic acid with respect to inoculum size is y = 0.16844 + 0.038766x1 − 0.00318x12, and the plot is shown in . Lactic acid amount (mg/g) is per the solid-state fermentation medium (WB and the pretreated DH generate the lactic acid amount).
The equation for the amount of lactic acid with respect to fermentation time is y = −0.163603 + 0.021638x2 − 0.00026x22, and the plot is shown in .
The equation for the amount of lactic acid with respect to the substrate ratio is y = −0.34681 −0.2974x3 − 0.03491x32. The plot is shown in .
The above figures demonstrated that among the three factors, the fermentation time and the substrate ratio both have significant effects on the amount of lactic acid produced, followed by inoculum size.
Verification of the regression model
The principle for production of L-lactic acid by solid-state fermentation of Huaimi dregs by using R. oryzae is as follows: R. oryzae is able to degrade external starch by glucoamylase and external waste fibres by cellulase. The lactic acid fermentation follows the Embden–Meyerhof–Parnas pathway, and pyruvate is produced. Part of the pyruvate enters the three tricarboxylic acid cycle, and another part generates lactic acid, ethanol, malic acid and fumaric acid under the catalytic action of lactate dehydrogenase, pyruvate decarboxylase and pyruvate carboxylase, respectively.
The optimum conditions predicted from the regression model were verified by three experimental repetitions. The resulting amounts of lactic acid were 0.2873, 0.2862 and 0.2869 mg/g. The experimental results demonstrated that the prediction from the model performed well.
Lactic acid is a green platform chemical, and therefore its raw materials should be non-toxic and green. This makes renewable biomass resources the ideal raw materials for lactic acid production. In 2015, there were more than 600 manufacturers of pharmaceutical products containing rutin, which is mainly extracted from Huaimi. In China, the annual emissions of Huaimi dregs are about 20,000 tons [Citation41]. Plant residues containing large amounts of cellulose, such as angelica, elderberry or straw, can be used to produce lactic acid. This study demonstrates a new use of Chinese medicine residues for the production of lactic acid.
Conclusions
In this study, we combined uniform and orthogonal experimental designs, and successfully optimized the parameters for the production of L-lactic acid from Huaimi dregs using solid-state fermentation. One kilogram of Huaimi dregs was able to produce 286.6 mg of lactic acid after statistical optimization of the medium containing a mixture of WB and Huaimi dregs, using R. oryzae strain. The optimal conditions were: substrate ratio of WB to Huaimi dregs of 4.26:1, inoculum size of 6.1%, fermentation temperature of 28 °C and fermentation time of 41.6 h. This process has a number of advantages: the aerobic fermentation using R. oryzae based on Huaimi dregs opens up new sources of raw materials for lactic acid production, which could reduce the use of food products, such as sweet potatoes and corn, for non-food purposes. The proposed fermentation process is simple, cost-effective and stable. This process would not only bring considerable economic benefits for pharmaceutical companies, it could also reduce landfill use, and improve the air, water and soil environments. Using discarded dregs as cheap raw materials is an important way to reduce costs, while reducing food use and alleviating the competition for food between humans and animals.
Acknowledgement
We thank Yang Xu and Xiaoyan Yu, of Functional Food Research Centre, for analysis of the sample.
Disclosure statement
The authors declare no conflict of interest.
Additional information
Funding
References
- Wang B, Jin Q. Fermentation production of organic acids and Application Manual. Beijing: China Light Industry Press; 2000.
- Wang D. Development and use of L-lactic acid. Chem Intermedia. 2004;7:7–14.
- Chen L, Li S, Yan Y, et al. Syntheses and applications of polylactic acid and its derivatives. Hubei Chem. 2001;3:4–5.
- Qiao C, Tang F, Zhu X. Present situation of production and research of L-lactic acid. J Ningxia Agr College. 2001;22(3):75–79.
- Xu Z, Yang P, Wang Q, et al. Advance on the production and application of L-lactic acid. Chem and Adhe. 2004;4:214–217.
- Wang R, Wang Y, Chen G, et al. Advances in fermentation of L(+)-lactic acid by Rhizopus Oryzae. J Chongqing Univ (Nat Sci Ed). 2004;27(12):95–97.
- Tong M, Fang X, Liu S, et al. Progress in research of L -lactic acid and polylactic acid. Petrochem Tech. 2005;3:724–728.
- Martin O, Averous L. Poly {lactic acid}: plasticization and properties of biodegradable multiphase systems. Polymer. 2001;42:6209–6219.
- Zhao P, Huang X. Progress on biofermentative production of lactic acid from renewable resources and wastes. Food Fermentation Ind. 2001;27(4):60–65.
- Liu W. The recent advances in development of lactic acid and polylactic acid. Food Fermentation Ind. 2001;27(3):61–65.
- Cao B, Xu J. Summary of L-lactic acid. Food Fermentation Ind. 1993;3:56–61.
- Qian Z, Lao H, Wang J. Recent progress in industrial lactic acid. Chinese J Bioprocess Eng. 2003;1(1):23–27.
- Wu H. Development and application of lactic acid and its derivatives in China and abroad. J Northwest Univ Nationalities (Nat Sci). 2010;31(2):67–70.
- Qian Z, Hu J, Lei Z. The industrialized production, application and market of lactic acid. Ind Microbiol. 2001;31(2):49–53.
- Cui L, Guo X, Wang Z. Studies on lactic acid yeasts and their functions in feed additives. Food Fermentation Ind. 2009;5:118–120.
- Xu H, Li C. Production and development of lactic acid and its derivatives. Fine Specialty Chem. 1998;1:3–7.
- Zhang X, Wang X, Chen H, et al. Advance of poly (lactic acid) modified chitosan in the field of Biomedica. Polymer Bul. 2013;11:62–69.
- Xie H, Zhou C, Li J, et al. Research and development of lactic acid and its derivatives. Chem World. 2007;7:437–411.
- Shao R, Yu X, Liu S. Study on the production of L-lactic acid from beer grain. Food Sci. 2008;29(8):467–470.
- Zhang N, Zhang J. Recent progress in study of bio macromolecular material poly. J Hebei Polytechnic Univ (Nat Sci Ed). 2010;32(3):116–120.
- Honarbakhsh, S, Pourdeyhimi, B. Scaffolds for drug delivery, part I: electrospun porous poly(lactic acid) and poly(lactic acid)/poly(ethylene oxide) hybrid scaffolds. J Mater Sci. 2011;46(9):2874–2881.
- Weng Y. Review of study of synthesis, production, process and application of PLA. Plast Ind. 2007;35(6):69–73.
- Cao Y, Yin J, Yan S. Recent research advance in biodegradable poly(lactic acid)(PLA): modification and application. Chinese Polymer Bull. 2006;10:90–97.
- Cao D, Wu L, Li B, et al. Progress in nanocomposites of polylactic acid. Chinese Polymer Bull. 2007;10:15–20.
- Bao J, Chu D, Zhao K, et al., inventor; East China University of Science and Technology, assignee. A method for the production of lactic acid by saccharification and fermentation of lignocellulose. China patent CN 102,586,348A. 2012 July 18.
- Wang Q, Wang X, Wang X, inventor; Harbin Institute of Technology, assignee. Method for the production of lactic acid and feed by open fermentation of food waste. China patent CN 1,843,152. 2006 Oct 11.
- Zou Y, Wu J, Yang L, et al. Research progress on comprehensive utilization of Chinese medicine. Jiangsu J Trad Chinese Med. 2008,40(12):1–3.
- Tan X, Wang X, Huang J, et al. Research progress of recycling technology of Chinese medicine. Chinese Trad Patent Med. 2010;32(5):847–849.
- Wang B, Wang X, Qin L. Solid-state fermentation of Chinese herbal medicine production of protein feed. J Food Biotechnol. 2007;26(4):77–82.
- Tang M, Cheng W, Wang X. Study on the effect of quick-ripening raw soil of traditional Chinese medicine residue. Changjiang Vegetable. 2005;8:54–55.
- Li F, Li Q, Li T. Research progress on determination methods of active constituents in Huaimi. Inf Trad Chinese Med. 2008;25(4):19–21.
- Zhang H, Zhang H. Study on extraction technology of rutin from Flos Sophorae. World Chinese Med. 2016;11(4):722–724.
- Jin C, Zhou J, Cui P, et al. Research progresses of aspergillus transforming traditional Chinese medicinal materials. J Hunan Univ CM. 2016;36(6):89–92.
- Zang Z, Cao L, Zhong L. Research progress of pharmacological action and preparation of rutin. Herald Med. 2007;26(7):758–760.
- Meng G, Chen Z, Bong C. Experimental study on the protective effect of rutin on cerebral ischemic injury in mice. Chinese Pharm Bull. 2000;16(3):305–307.
- Zhu J, Huang Y. Effect of rutin on lipid peroxidation in ovariectomized rats. Preventive Med. 2002;9(6):628–629.
- Chen Y, Ma L, Hu L, et al. Inhibition effects and mechanisms of sophora flower bud abstract on tumor in S180 tumor-bearing mice. Pharmacol Clinics Chinese Materia Med. 2014;30(5):100–102.
- Li D, Liu C, inventor; Shanxi Xin Zhongda Biotechnology Co., Ltd., assignee. A kind of livestock and poultry feed produced by using Sophora japonica solid residue and its preparation method. China patent CN 103,892,045A. 2014 July 2.
- Li W. Brief introduction of plant extract in domestic and foreign markets. Phar Chem Eng. 2014;11:23–31.
- He W, Xue W, Tang B. Optimization methods and experimental design and data analysis. Beijing: Chemical Industry Press, 2012. p. 74–80.
- China Food and Drug Administration [ Internet]. Beijing: CFDA. [ cited 2016 May 6]. Available from: http://app2.sfda.gov.cn/datasearchp/gzcxSearch.do?formRender=cx&page=1