ABSTRACT
Although DNA vaccines have shown a good antitumor effect in animal models, they are often not effective in clinical trials. This requires researchers to find an effective way to significantly improve the immunogenicity of vaccines. Here, we developed a new DNA vaccine based on MAGE-A3, which has been suggested as a potential target for lung cancer therapy. We enhanced the potency of this DNA vaccine by overcoming tumour-induced immunosuppressive environment through blockade of the PD-1/PD-L pathway using a soluble PD-1 (sPD1). A series of DNA plasmids encoding MAGE-A3, the extracellular domain of murine PD-1 (sPD1) and their conjugates were constructed and injected into female mice intramuscularly (i.m.) followed by an electric pulse. The humoral and cellular immune responses after immunization were evaluated by enzyme-linked immunosorbent assay (ELISA) and enzyme-linked immunospot assay (ELISPOT), respectively. To evaluate the therapeutic efficacy of the plasmids, a mouse model with a MAGE-A3-expressing tumour was designed. Mice vaccinated with the fusion expression plasmid generated the strongest MAGE-A3-specific immune responses. Furthermore, these vaccinations inhibited the growth of MAGE-A3-expressing tumours and prolonged mouse survival. These findings suggest that the combination of DNA vaccines with PD-1 pathway inhibitors may be a promising approach in clinical trials. This approach may be viable for vaccines targeting cancer, as anti-tumour vaccines have demonstrated clinical benefit but PD-1 pathway inhibitors alone have demonstrated poor efficacy so far.
KEYWORDS:
Introduction
DNA vaccines are also known as nucleic acid vaccines, which are composed of an expression plasmid harbouring a target antigen. Once a DNA vaccine is injected into the body, the exogenous gene is expressed, which could activate the body's humoral and cellular immune response [Citation1]. Currently, DNA vaccines have become one of the hot topics of research, especially for the treatment of malignant tumours and infectious diseases [Citation2,Citation3].
DNA vaccines have shown certain effectiveness, with a low level of toxicity in animal and human trials [Citation4]. However, the traditional DNA vaccine, which contains only antigen components, can hardly produce efficient CD8+ T cell responses despite the use of electroporation (EP) [Citation5]. This has called for further efforts towards improvement of the immunogenicity of DNA vaccines [Citation6].
The use of immune check-point inhibitors that target PD-1 and CTLA-4 in cancer treatment is emerging as being effective in cancers [Citation7]. PD-L1 is constitutively expressed on T cells, B cells, macrophages and dendritic cells (DCs) [Citation8,Citation9]. The essential role of the PD-1/PD-L1 pathway in modulating immunity against cancer has been well established [Citation10,Citation11]. The upregulation of PD-1 is associated with dysfunction of T and B cells. Therefore, reduction or blockade of the PD-1/PD-L signalling pathway using PD-1 or PD-L antibody or soluble PD-1 protein could prevent T cell dysfunction to a large extent, so as to improve the immune therapeutic effect against tumours or viruses [Citation12–14].
We therefore hypothesize that a soluble PD-1 (sPD1)-based DNA vaccine may improve the adaptive T cell immunity by overcoming tumour-induced immunosuppressive environment through blockade of the PD-1/PD-L pathway in vivo. To test this hypothesis, a DNA vaccine was constructed by cloning MAGE-A3 (Melanoma Associated Antigen 3) gene and murine sPD1 genes linked by Furin-2A (F/2A) [Citation15] into pVAX1. The fusion construct was designed to improve the immunogenicity of a lung cancer DNA vaccine, and this study explores the resulting anti-tumour activity.
Materials and methods
Plasmid DNA constructs
Based on the coding regions of MAGE-A3 (GenBank: NM_005362.3) and murine PD-1 (GenBank: NP_032824.1), we designed a MAGE-A3 (coding gene: 210-1154) and murine sPD1 (extracellular 21-169aa) fusion gene. The MAGE-A3 sequence was connected to sPD1 by Furin-2A. Synthesis of the fusion gene was carried out by Invitrogen (San Diego, USA). The fusion gene was released from the cloning vector by NheI and XhoI and then cloned into the eukaryotic expression vector pVAX1. This resulted in the eukaryotic expression plasmid pVAX1-MAGE-A3-sPD1. The plasmid was transiently transfected into 293T cells, and Western blot was performed to confirm the validity of this construct.
Cell culture
The 293T cells and the Lewis carcinoma cell (LLC) line purchased from the Shanghai Cell Institute (Shanghai, China) were maintained in Dulbecco minimum essential medium (DMEM) supplemented with 10% fetal bovine serum (FBS) and antibiotics.
Mice
The mice were maintained in accordance with the Guide for the Care and Use of Laboratory Animals (National Institutes of Health Publication No. 85-23, Revised 1996). All animal experiments were approved by the Committee on the Use of Live Animals of General Hospital of Chinese People's Armed Police Forces. Six- to eight-week-old female C57BL/6 mice were maintained according to standard operational procedures at the General Hospital of Chinese People's Armed Police Forces Laboratory Animal Unit.
MAGE-A3-expressing tumour cell lines
To generate a cell line stably expressing MAGE-A3, LLC cells were transfected with a plasmid pIRES-neo-MAGE-A3 and then selected with 0.5 mg/mL G418. This cell line is hereafter referred to as LLC-MAGEA3. Single clones were obtained by limiting dilution of neomycin-resistant cells, and the MAGE-A3 expression was measured by Western blot.
Mouse immunization and tumour challenge
Mice received three DNA immunizations by i.m. injection with EP (60 V/mm distance between the electrodes; 50 ms pulse length; 6 pulses) given every 1 week at a dose of 30 μg per mouse. Two weeks after the final immunization, the mice were sacrificed, and sera and splenocytes were collected for immune response analysis. For tumour inoculation, C57BL/6 mice received 1 × 106 LLC-MAGEA3 cells in the right hind flank subcutaneously. Tumour size was measured using calipers [tumour volume was calculated according to the following formula: V (mm3) = 0.5 × long diameter × short diameter2]. Tumours were removed and weighed, and the tumour growth inhibition rate was calculated. The formula for tumour inhibition rate was as follows: (average tumour weight in the control group − average tumour weight in the treatment group)/ average tumour weight of the control group × 100%.
Assaying serum for MAGE-A3 antibodies
Specific antibody responses were assessed by enzyme-linked immunosorbent assay (ELISA). Briefly, high-affinity, protein-binding ELISA plates (BD Biosciences, Franklin Lake, USA) were coated with MAGE-A3 protein (Abcam, Cambridge, Britain). Serial diluted sera were then added and antibodies detected with horseradish peroxidase (HRP)-labelled anti-mouse IgG1 or IgG2a antibody (Sigma-Aldrich, Darmstadt, Germany). Relative antibody titers were expressed as the reciprocal highest dilution of samples producing at least a two-fold greater optical density readout than that of the control serum sample at the same dilution.
ELISPOT assay
Interferon gamma (IFN-γ)–producing T cells were evaluated by an ELISPOT assay (Dakewe Biotech Ltd., Shenzhen, China). Briefly, 10 μg/mL recombinant MAGE-A3 protein were used to stimulate splenocytes in vitro. Cells stimulated with 500 ng/mL phorbol 12-mystrate 13-acetate (PMA; Sigma-Aldrich) plus 1 μg/mL calcium ionocycin or left in media only served as positive and negative controls, respectively. Cells were incubated at 37 °C, 5% CO2 and 100% humidity for 36 hours. Spots were identified by an immunospot reader and image analyzer (Thermo Scientific).
Cytokine production assay
Splenocytes were prepared and cultured with MAGE-A3 (5 µg/mL) (Abcam, ab99958) using the same procedure as that used for ELISPOT assays. Culture supernatants were collected on the third day. The concentrations of IFN-γ and interleukin-4 (IL-4) were measured by ELISA using commercial cytokine assay kits (Abcam, ab100689 and ab100710, respectively), according to the manufacturer's instructions.
Statistical analysis
Differences between groups were analyzed by one-way analysis of variance using the GraphPad Prism 5 software. P < 0.05 was considered to indicate statistically significant differences.
Results and discussion
Molecular cloning and expression of DNA vaccine plasmids in 293T cells
Two DNA vaccines: pVAX1-MAGEA3 and pVAX1-MAGEA3-sPD1, consisting of MAGE-A3 or/and sPD1, were designed and generated to test our working hypothesis ((A)). The expression of fusion proteins encoded by these DNA vaccines was detected by Western blot using either anti-MAGE-A3 (Abcam, ab140678) or anti-murine PD-1 (GeneTex, GTX17271) antibody ((B)). The results showed that the fusion gene was expressed in 293T cells.
sPD1-fusion DNA vaccination enhances MAGE-A3-specific T cell immunity and antibody responses in vivo
The two DNA vaccines were subsequently evaluated in vivo for their immunogenicity, and pVAX1 empty vector was used as the negative control. As intramuscular injection followed by EP has been reported to improve the immunogenicity of DNA vaccines by enhancing antigen expression and recruiting DCs [Citation16,Citation17], for vaccination in mice, we selected an initial dose of 30 μg DNA per shot.
The effects of the sPD1-fusion DNA vaccine on the specific T cell response were evaluated by ELISPOT assay at two weeks after the last immunization ((A)). We found that IFN-γ was increased significantly in the splenocytes of sPD1-fusion DNA vaccinated mice ((B)). As shown in (B), the number of spots observed in this group was higher (about two-fold) than that in the non–sPD1-fusion DNA group (P < 0.0001). These data indicate that sPD1-fusion DNA vaccination significantly enhances the release of the cytokine IFN-γ.
Figure 2. Induction of enhanced MAGE-A3-specific immune responses by sPD1 fusion vaccination. (A) DNA vaccine immunization schedule for C57BL/6 mice. (B) IFN-γ production as a specific response to MAGE-A3 protein determined by antigen ELISPOT assay. (C) IgG1 and IgG2a antibodies specific to MAGE-A3 in sera detected by ELISA. (D) Cytokine profile of proliferating T cells detected by ELISA.
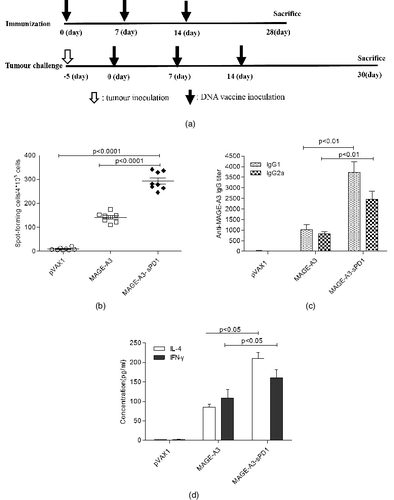
On day 14 after vaccination, the serum from each mouse was collected and the anti-MAGE-A3 level was monitored. As shown in (C), the non–sPD1-fusion DNA group and sPD1-fusion DNA group could elicit anti-MAGE-A3 antibody after vaccination, however, the level of specific anti-MAGE-A3 antibody in the mice vaccinated with sPD1-fusion DNA was approximately 3–4 times higher than in those vaccinated with non–sPD1-fusion DNA (P < 0.01).
Therefore, immunization with the sPD1-fusion DNA not only elicits higher titers of antibody but also induces a specific T cell response, indicating that the fusion gene vaccine might be effective in the control and treatment of lung cancer.
Augmentation of both MAGE-A3-specific Th1 and Th2 responses by the sPD1-fusion DNA vaccine
Since the quality of the immune response is very crucial for the efficacy of any vaccine, we analyzed the polarization of the immune response by determining the generated antibody subclasses. The MAGE-A3-specific IgG was subtyped by ELISA using IgG1 and IgG2a antibodies in mice vaccinated with either sPD1-fusion DNA or non–sPD1-fusion DNA at week 2 after the final immunization. (C) shows that the sPD1-fusion construct mediated both MAGE-A3 specific IgG2a (Th1) and IgG1 (Th2) antibody responses. Compared with non–sPD1-fusion DNA vaccination, the level of IgG2a was increased about three-fold, whereas the level of IgG1 showed about a four-fold enhancement.
To further characterize the immune response polarization, the IFN-γ and IL-4 levels were also measured as indicators of the Th1 and Th2 response, respectively. As shown in (D), in the case of Th1 cytokines, the IFN-γ release from splenocytes of mice immunized with the sPD1-fusion DNA was increased greatly. As for the Th2 cytokines, the IL-4 release was higher in mice vaccinated with the sPD1-fusion DNA than in those vaccinated with non–sPD1-fusion DNA or pVAX1 empty vector. Therefore, vaccination with the sPD1-fusion DNA construct induced both specific Th1 and Th2 responses.
Enhancement of the antitumour effective in vivo
In recent years, targeting the delivery of DNA vaccines to professional antigen-presenting cells, such as dendritic cells, and thus improving the immunogenicity of the vaccine, is one of the research hot topics [Citation18]. In these studies, the antigen of interest is targeted to DC by its surface molecules, such as CD205 [Citation19]. However, there is less research on targeting DC cells through the fusion expression of antigens and PD-1. PD-L1 are mainly expressed in the T, B cells and macrophages and PD-L2, in DCs. In malignant tumours and infectious diseases, the immunity is down-regulated through PD-1/PD-L1 pathway [Citation20,Citation21].
Therefore, the antigen targeting DC by using anti-PD-1 antibody or a soluble PD-1 can not only improve the efficiency of antigen presentation, but also block the PD-1/PD-L1 pathway. The exhaustion state of T cell function was relieved, thereby improving the immunogenicity [Citation22–24].
To test this hypothesis, in our study, the effect of immunotherapy was evaluated by measuring the tumour size. Five days after tumour cell injection, mice were vaccinated three times with the DNA vaccine, and the tumour volume was measured every 4 days. The tumour growth in mice treated with sPD1-fusion DNA was significantly inhibited compared with that in the pVAX1 vector group or non–sPD1-fusion DNA group (P < 0.05) ((A)). Additionally, the tumour weight was measured ((B)) and the tumour growth inhibition rate was calculated after vaccine treatment. The results showed that the tumour growth inhibition rates were significantly higher in the mice that received sPD1-fusion DNA than in the mice that received non–sPD1-fusion DNA (P < 0.05) or pVAX1 vector (P < 0.05) ((C)).
Figure 3. sPD1-based DNA vaccination–induced antigen-specific immunity confers protection against tumour challenge. Growth curve of LLC-MAGE-A3 tumour (A); mean tumour weight (B); tumour inhibition rate (C).
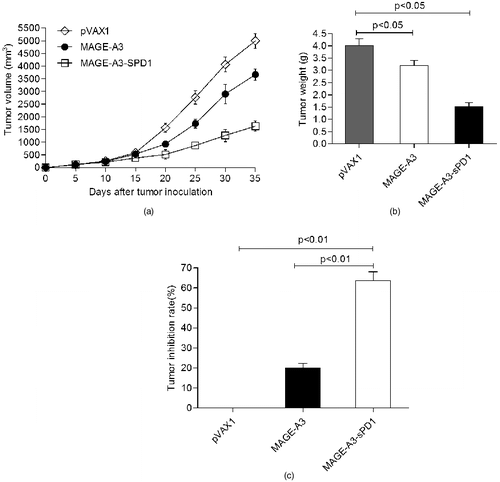
Through the above experiments, it can be seen that the use of a sPD1-fused vaccine is a feasible and effective approach to overcome the immune tolerance and improve the immunogenicity. All in all, sPD1-fusion DNA vaccine can significantly improve the anti-tumour effect, mainly because sPD1 can effectively block PD-L1 in vivo [Citation13], which can eliminate the inhibitory effects of the PD-1/PD-L1 pathway on T cell activation. Therefore, our results support the suggestion [Citation24] that the technique of increasing DNA vaccine immunogenicity by fusion expression of PD-1 and antigen could be considered a promising approach in anti-tumour therapy.
Conclusions
In this study, we demonstrated that vaccination with a sPD1-fusion DNA vaccine encoding MAGE-A3 enhanced both the B and T cell responses through the blockage effect of PD-1 on PD-L1. Moreover, the sPD1-fusion DNA vaccine was demonstrated to be significantly more potent in inhibiting tumour growth than the non–sPD1-fusion DNA. These promising results can serve as a further point of departure. We will carry out a more detailed investigation of the effectiveness and safety, and will fully evaluate the potential of this DNA vaccine as a therapeutic lung cancer vaccine.
Disclosure statement
The authors declare no conflict of interest.
Additional information
Funding
References
- Arce-Fonseca M, Rios-Castro M, Carrillo-Sánchez Sdel C, et al. Prophylactic and therapeutic DNA vaccines against Chagas disease. Parasit Vectors. 2015 [cited 2016 Sep 01];8:121. DOI:10.1186/s13071-015-0738-0
- Marć MA, Domínguez-Álvarez E, Gamazo C. Nucleic acid vaccination strategies against infectious diseases. Expert Opin Drug Deliv. 2015;12(12):1851–1865.
- Yang B, Jeang J, Yang A, et al. DNA vaccine for cancer immunotherapy. Hum Vaccin Immunother. 2014;10(11):3153–3164.
- Grunwald T, Ulbert S. Improvement of DNA vaccination by adjuvants and sophisticated delivery devices: vaccine-platforms for the battle against infectious diseases. Clin Exp Vaccine Res. 2015;4(1):1–10.
- Lee SH, Danishmalik SN, Sin JI. DNA vaccines, electroporation and their applications in cancer treatment. Hum Vaccin Immunother. 2015;11(8):1889–1900.
- Rekoske BT, Smith HA, Olson BM, et al. PD-1 or PD-L1 blockade restores antitumor efficacy following SSX2 epitope-modified DNA vaccine immunization. Cancer Immunol Res. 2015;3(8):946–955.
- Wang SD, Li HY, Li BH, et al. The role of CTLA-4 and PD-1 in anti-tumor immune response and their potential efficacy against osteosarcoma. Int Immunopharmacol. 2016;38:81–89.
- Hofman V, Ilie M, Long E, et al. Issues and current limits for immunohistochemical assessment of PD-L1 status in bronchial biopsies. Bull Cancer. 2016;103(4):368–380.
- Merelli B, Massi D, Cattaneo L, et al. Targeting the PD1/PD-L1 axis in melanoma: biological rationale, clinical challenges and opportunities. Crit Rev Oncol Hematol. 2014;89(1):140–165.
- Ma W, Gilligan BM, Yuan J, et al. Current status and perspectives in translational biomarker research for PD-1/PD-L1 immune checkpoint blockade therapy. J Hematol Oncol. 2016 [cited 2016 Sep 01];9(1):47. DOI:10.1186/s13045-016-0277-y
- Festino L, Botti G, Lorigan P, et al. Cancer treatment with anti-PD-1/PD-L1 agents: is PD-L1 expression a biomarker for patient selection? Drugs. 2016;76(9):925–945.
- Kline J, Gajewski TF. Clinical development of mAbs to block the PD1 pathway as an immunotherapy for cancer. Curr Opin Investig Drugs. 2010;11(12):1354–1359.
- Chikuma S. Basics of PD-1 in self-tolerance, infection, and cancer immunity. Int J Clin Oncol. 2016;21(3):448–455.
- Zhou J, Cheung AK, Tan Z, et al. PD1-based DNA vaccine amplifies HIV-1 GAG-specific CD8+ T cells in mice. J Clin Invest. 2013;123(6):2629–2642.
- Ho SC, Bardor M, Li B, et al. Comparison of internal ribosome entry site (IRES) and Furin-2A (F2A) for monoclonal antibody expression level and quality in CHO cells. PLoS One. 2013 [cited 2016 Sep 01];8(5):e63247. DOI:10.1371/journal.pone.0063247
- Lambricht L, Lopes A, Kos S, et al. Clinical potential of electroporation for gene therapy and DNA vaccine delivery. Expert Opin Drug Deliv. 2016;13(2):295–310.
- Apostolopoulos V. Vaccine delivery methods into the future. Vaccines (Basel). 2016 [cited 2016 Sep 01];4(2):9. DOI:10.3390/vaccines4020009
- Grodeland G, Fredriksen AB, Løset GÅ, et al. Antigen targeting to human HLA class II molecules increases efficacy of DNA vaccination. J Immunol. 2016;197(9):3575–3585.
- Njongmeta LM, Bray J, Davies CJ, et al. CD205 antigen targeting combined with dendritic cell recruitment factors and antigen-linked CD40L activation primes and expands significant antigen-specific antibody and CD4 (+) T cell responses following DNA vaccination of outbred animals. Vaccine. 2012;30(9):1624–1635.
- Latchman Y, Wood CR, Chernova T, et al. PD-L2 is a second ligand for PD-1 and inhibits T cell activation. Nat Immunol. 2001;2(3):261–268.
- Freeman GJ, Long AJ, Iwai Y, et al. Engagement of the PD-1 immunoinhibitory receptor by a novel B7 family member leads to negative regulation of lymphocyte activation. J Exp Med. 2000;192(7):1027–1034.
- He L, Zhang G, He Y, et al. Blockade of B7-H1 with sPD-1 improves immunity against murine hepatocarcinoma. Anticancer Res. 2005;25(5):3309–3313.
- Velu V, Titanji K, Zhu B, et al. Enhancing SIV-specific immunity in vivo by PD-1 blockade. Nature. 2009;458(7235):206–210.
- Yang Z, Jiang DD, Zhu Q, et al. The immunogenicity and anti-tumor effects of a lung cancer DNA vaccine harboring a MUC-1 and GM-CSF fusion gene. Braz Arch Biol Technol. 2016 [cited 2017 Jun 12];59:e16150208. DOI:10.1590/1678-4324-2016160208