ABSTRACT
Most oral rinses contain active ingredients with limited antifungal activity, particularly against hyphae development. This has encouraged us to search for bioactive compounds effective against preventing morphology transition of the dimorphic Candida. The antifungal activity of the selected bioactive compounds against seven species of oral-associated Candida was screened before the evaluation of their potency to inhibit hyphal formation. The identified potent inhibitor was then further investigated on its effectiveness through a brief treatment test on germination, adhesion, cellular morphology and gene expression. Bakuchiol, hydroxychavciol and pseudolaric acid B showed antifungal activity while luteolin and sakuranetin were determined to be inactive against the oral-associated Candida species at a concentration below 1 mg/mL. Hydroxychavicol was determined as a potent inhibitor against hyphal growth of Candida albicans. Hydroxychavicol delayed the germination process of C. albicans by affecting the expression of RAS1, NRG1 and HWP1 genes up to 1 h after the treatment. In addition, the treatment caused minimal changes to the cellular morphological structure. But, hydroxychavicol showed poor anti-adherence activity against the germinated cells. In summary, hydroxychavicol is one of the identified bioactive compounds that possessed anti-candida properties against oral-associated Candida species and appeared potent in inhibition of hyphae of C. albicans by affecting its ultrastructural morphology and gene regulation. This finding could suggest development of hydroxychavicol as a bioactive compound that can potentiate the activity of oral rinse in preventing the colonization of dimorphic Candida in the oral cavity.
Introduction
Candidiasis represents one of the leading cause of nosocomial infections in public health [Citation1]. About 96% of invasive fungal infections including candidiasis are caused by genus Candida [Citation2]. Candida albicans is the most common species isolated from oral cavity while other species are infrequently but consistently isolated [Citation3]. Candida albicans has extensively developed its virulence traits for survival. It is a dimorphic fungus which is able to alternate between yeast and hyphae in certain environmental conditions [Citation4]. Several lines of evidence suggest that hyphae morphology plays a key role in adhesion to epithelial cells to cause oral candidiasis [Citation5] and it also contributes to formation of biofilms [Citation6]. Other than C. albicans, Candida dubliniensis, Candida tropicalis and Candida lusitenae are also able to produce hyphae [Citation7].
The morphology transition in C. albicans is controlled by regulatory genes such as Ras-like Protein 1 (RAS1) and Negative Regulator of Glucose-repressed 1(NRG1) [Citation8,Citation9]. Additionally, the presence of hyphae also facilitates the adhesion activity which is mediated by Adhesin-like Hyphal Cell Wall Protein 1 (HWP1) [Citation10]. This protein functions in generating the adhesion of C. albicans to host tissue surfaces. The existence of adhesin is essential for the Candida pathogenesis particularly in candidiasis infection [Citation11,Citation12]. HWP1 is also crucial to strengthen the biofilm structure [Citation13]. Since HWP1 is hyphae-specific, its expression is highly regulated in hyphae rather than yeast form [Citation14,Citation15].
In the past few years, the use of oral rinse as a hygiene practice has increased dramatically. However, certain active ingredients in oral rinse such as sodium monofluorophosphate and thymol have been discovered to have weak activity against hyphae formation of C. albicans [Citation16]. This may contribute to resistance of Candida biofilm formation in the oral cavity and limits the usage of oral rinses in the treatment of oral candidiasis as well. Many studies have now focused on searching novel bioactive compounds from natural products as an alternative to the current antimicrobial agents [Citation17–19]. Bioactive compounds such as hydroxychavicol, bakuchiol, luteolin, pseudolaric acid B and sakuranetin have been identified to have antimicrobial properties [Citation17,Citation19–22]. However, actual potency of the bioactive compounds as antifungal agents particularly against dimorphic Candida remains scarce and insufficient.
Therefore, the present study was designed to investigate their antifungal activities against the common oral Candida species and its virulence capability involving hyphae growth. Oral rinse that is formulated with a potent antifungal agent developed from bioactive compounds is hoped to be reliable in the prevention of oral candidiasis.
Material and methods
Candida strain
All seven Candida type strains: C. albicans ATCC 14 053, C. dubliniensis ATCCMYA-2975, Candida glabrata ATCC 90 030, Candida krusei ATCC 14 243, C. lusitaniae ATCC 64 125, Candida parapsilosis ATCC 22 019 and C. tropicalis ATCC 13 803 were purchased from American Type Culture Collection (ATCC) and the samples were delivered in lyophilized form in vials. The lyophilized cells were rehydrated in sterile distilled water, followed by inoculation onto Yeast Peptone Dextrose (YPD) agar media and then incubated overnight at 37 °C for 24 h to recover the cell growth as recommended on the product sheet of ATCC. Following incubation, a single colony from the agar was sub-cultured onto fresh YPD agar slants before being kept at 4 °C for short-term storage stock culture. For long-term storage, stock culture was prepared in 20% (v/v) glycerol and kept at −70 °C. During the experiment, working cultures were prepared on YPD agar and maintained for two weeks at 4 °C. Routine sub-culturing was carried out before being used in any experiment to ensure the viability of cells. The growth colonies from the culture were used in cell suspension preparation.
Bioactive compounds
Bakuchiol, hydroxychavicol, luteolin, PAB and sakuranetin were purchased from ChromaDexInc., USA. All bioactive compounds were delivered in lyophilized form in vials. The bioactive compounds were dissolved in 50% dimethyl sulphoxide (DMSO) as stock solution, and kept at −20 °C for long-term storage. During the experiment, working solution was freshly prepared through dilution of stock with sterile distilled water to obtain 4 mg/mL of the respective bioactive compounds in 1% DMSO. A 0.12% chlorhexidine gluconate (CHX) was used as positive control in the study.
Media
YPD agar and broth were used as the growth media for all Candida strains in the study. The media was initially dissolved in distilled water before further sterilized at 121 °C for 15 min in an autoclave. The sterilized YPD agar media was poured into 15 mm agar plates while the sterilized broth media was kept in Schott bottle and stored at 4 °C for later use.
An induction medium was prepared to stimulate the germination of Candida cells. The induction media is a mixture of YPD broth with 10% fetal bovine serum (FBS) and named as YPDS. During preparation, YPD growth media and FBS were sterilized separately. YPD was sterilized through heat sterilization using autoclave while FBS was sterilized through microfiltration using 0.22 µm membrane filter. Following sterilization, YPD was left to cool to about 50–60 °C before being added with 10% FBS to produce YPDS. YPDS was freshly prepared each time prior to the experiment.
Standard cell suspension
Candida cell suspension was adjusted to a 0.5 McFarland standard which contain approximately 1 × 106 yeast cells/mL. Briefly, a loopful of Candida colony from fresh culture agar was inoculated into 5 mL of YPD broth and mixed by vortex. The turbidity of cell suspensions was then adjusted spectrophotometrically to achieve an optical density (OD550 nm) of 0.144 which is equivalent to the 0.5 McFarland standards.
Minimum inhibitory concentrations (MIC) determination
In our study, the MIC was determined using Clinical and Laboratory Standards Institute M38-A2, a reference method for broth dilution antifungal susceptibility testing with some modifications [Citation23]. A 50 μL of YPD broth was dispensed into 96 wells microdilution plate marked as Well 1 (W1) to Well 12 (W12). Following this, 50 μL of the working solution (4 mg/mL) of the bioactive compounds was added into W1 and twofold serial dilution was carried out from W1 to W10. CHX was used as positive control in W11 and W12, which contained only YPD broth, represented the negative control. A 50 μL of standard yeast cell suspension was added into each well (W1–W12). Hence, the final concentrations of the compounds in W1–W10 were 1000, 500, 250, 125, 62.5, 31.25, 15.63, 7.81, 3.91 and 1.95 µg/mL in the respective wells. Triplicate samples were performed for each test concentration. The microdilution plates were incubated overnight at 37 °C in an aerobic incubator.
Following this, the inhibition of Candida growth was determined spectrophotometrically using a microplate reader (PowerWave 200, BioTek Instruments, Winooski, VT, USA) at a wavelength of 550 nm. MIC was determined as the lowest concentration of the compounds that inhibit Candida cell growth. The MIC value reported in the study was the median of three independent tests to ensure reproducibility of research data.
Inhibitory concentration (IC50) on germination
At initial stage, all seven Candida were first screened for germination using the induction media, YPDS for 3 h at 37 °C. Following that, only the strain with positive germination was used for the next experiment. A 50 µL of standard yeast cell suspension was added into a 96 well microliter plate containing 50 µL of the induction media that has been twofold serially diluted with the respective compounds and then incubated at 37 °C for 3 h to induce germination. After incubation, the method of crystal violet staining (colorimetric assay) [Citation24] was then used to determine IC50 value. In this context, IC50 was the concentration of compounds required for 50% inhibition of germinated Candida strains. IC50 and 95% confidence interval (CI) of the IC50 were determined using GraphPad Prism version 3.00 for Windows (GraphPad Software, San Diego, CA, USA).Triplicate samples were performed for each test concentration and the IC50 value reported in the study was the median of three independent tests to ensure reproducibility of research data.
Assessment of potent inhibitor against germinated Candida
Since the usage and recommendations for using oral rinse for each gargle are practically between 1 and 2 min, we take into consideration using similar exposure period of the bioactive compounds in this study. This experiment is basically assessing the post effect on germinated Candida cells after being exposed to the potent inhibitor for 2 min with focus on germination inhibition, cellular morphological changes, adherence inhibition and gene expression changes.
In this experiment, sample preparation for cell treatment was prepared by incubating 1 mL of standardized yeast cells suspension in the presence of the compound at IC50 (treated) for 2 min at 37 °C in the incubator. After incubation, the cells were subsequently washed twice with PBS and collected by centrifugation at 3000 g at 37 °C for 10 min. Untreated yeast cells of Candida, which act as control of the experiment, were prepared similarly without the addition of the compound.
Germination inhibition
The washed cells were suspended in 1 mL of induction media and 100 µL of the samples were then added into 96 well plates and incubated at 37 °C. The percentage of germ tube formation was checked every hour for the first 3 h of incubation using the crystal violet staining (colorimetric assay). After the absorbance reading at 590 nm wavelength, fold change of the germination for the treated sample was calculated as follows: (Abstreated − Abscontrol)/Abscontrol.
Cellular morphology
The washed cells were suspended in 1 mL of induction media and incubated at 37 °C. After 3 h of incubation, the cells were washed again with PBS twice prior to immersion in 1 mL mixture of gluteraldehyde 8% and Sorenson phosphate buffer solution (1:1 ratio) for 1 h for fixation. After that, the solution was discarded and the samples were washed with a mixture of Sorenson phosphate buffer solution and distilled water (1:1 ratio). Samples were further immersed in a mixture of osmium tetraoxide 4% and distilled water (1:3 ratio) at 4 °C for 14 h in fridge to coat the cells. After pipetting out the solution, the samples were then immersed for 15 min in the following series of solutions: distilled water, 10%, 20%, 30%, 40%, 50%, 60%, 70%, 80%, 90% and 100% ethanol in the fume hood for dehydration steps. Following that, samples were immersed in several respective ratios of a mixture of ethanol (100%, v/v) and acetone (100%, v/v) with a ratio of 3:1, 1:1, 1:3 for 20 min. Samples were immersed in acetone 100%, v/v for 20 min for four times and dried using critical drying point technique. Once the samples were completely dried, they were mounted on aluminium stubs, with copper tape and coated with gold under low-pressure with an ion sputter coater. Three samples from each treatment were visualized with a scanning electron microscope in high-vacuum mode at 15 kV and the images processed. Any considerable changes on the morphology between the untreated and treated cells were taken into account.
Adherence inhibition
In the experiment, unstimulated saliva was collected by expectoration from a healthy donor between 8 am and 11 am and kept in ice-chilled test tube. A single donor was used throughout the study to minimize variations in saliva composition that may arise between different individuals. The donor was asked to gargle with distilled water for a few seconds to clean and reduce bacterial contamination before the collection begins. The saliva was then clarified by centrifugation (17,000 g) for 30 min and the pelleted debris was discarded. The clarified saliva was then sterilized through microfiltration using 0.22 µm membrane filter. Collection from different batches was then pooled and stored at −20 °C until further use.
A 100 μL of the saliva was added in 96 well plates for 1 h to allow the formation of salivary pellicle in the wells. The saliva was then removed by gentle pipetting and the formed salivary pellicle was then incubated with 100 μL of the compound (treated) at IC50 for 2 min. Untreated salivary pellicle, which acts as the control of the experiment, was prepared similarly without the addition of the compound. The salivary pellicles were subsequently washed twice with PBS by pipetting. A 100 μL of 1 × 106 cells per millilitre of germinated cells, which was prepared prior to the experiment was added into the wells and then incubated at 37 °C. The adherence of germinated cells to the salivary pellicle was checked every hour for the first 3 h of exposure using the crystal violet staining (colorimetric assay). After the absorbance reading at 590 nm wavelength, fold change of the adherence for the treated sample was calculated as follows: (Abstreated − Abscontrol)/Abscontrol.
Gene expression
Total RNA extraction and cDNA synthesis
The washed cells were suspended in 1 mL of induction media and incubated for 3 h at 37 °C. The cells were washed again with PBS twice prior to RNA extraction process every hour of incubation period, from first to third hour. Total RNA was extracted using RiboPure™ Yeast RNA Purification Kit according to the manufacturer's instructions. The concentration of total RNA was standardized to 5 μg by sample dilution before use in cDNA synthesis step using SuperScript® VILO™ cDNA Synthesis Kit.
Development of primers
Candidate genes that are related to hyphae −RAS1, NRG1 and HWP1 were selected for gene expression analysis. DNA sequences of the related genes were obtained from the Candida Genome Database (www.candidagenome.org). Primers () were designed using National Center for Biotechnology Information Primer-Blast online software. The housekeeping gene actin1 (ACT1) was used as a control for normalization in real-time PCR experiments. Universal primer of ACT1 for yeast was obtained from previous study [Citation25]. For optimization purposes, the primers were subjected to standard PCR reaction.
Table 1. Details of forward and reverse primer of ACT1, RAS1, NRG1 and HWP1 used for gene expression analysis.
Real-time PCR
Real-time PCR was performed in MicroAmp® Fast 8-Tube Strip, 0.1 mL and run using the 7500 Fast Real-Time PCR System. The SYBR® green assay was used as a dye for the quantification of PCR product using SYBR® Select Master Mix kit. Real-time PCR reaction was performed at 95 °C for 5 min, followed by 40 cycles of 15 s at 95 °C and 1 min at 60 °C. Following PCR, samples were subjected to incubation of an ascending temperature starting from 60 to 95 °C to obtain melt curves. Control reaction was performed with RNA that had not been reverse transcribed in order to ensure that no genomic DNA amplified during the PCR reactions. Triplicate reactions were performed for each sample. RT-qPCR data analyses were performed using Applied Biosystems 7500 Fast Real-Time PCR System Software v2.3 after data collection from this experiment. Fold changes of gene expression were determined through double delta CT (2−ΔΔCT) value and relative quantification value was plotted in graph with comparison between treated and untreated sample for each incubation time.
Data analysis
Every test was run in triplicate and the data was recorded and analysed using IBM SPSS statistics v. 20 software with significance value acceptance p < 0.05.
Results and discussion
MIC of the selected bioactive compounds
MIC of the selected bioactive compounds against the Candida strains was determined as shown in . Three out of five selected bioactive compounds: bakuchiol, hydroxychavicol and PAB had antifungal activity against all seven Candida strains. Bakuchiol exhibited the most effective antifungal activity with the lowest range of MIC (31.3–125 µg/mL) followed by PAB (3.9–250 µg/mL) and hydroxychavicol (250–500 µg/mL). MIC of both luteolin and sakuranetin was unsuccessfully determined at concentration ≤1 mg/mL. Luteolin and sakuranetin were previously described to be active against dermatophytes and rice blast fungus [Citation19,Citation20]; however, both were found to be inactive against the Candida species tested in our study. This finding suggests that both compounds, luteolin and sakuranetin, are not anti-candida agents. Based on the chemical structure, the selected bioactive compounds are categorized into three different groups which is terpene (bakuchiol and PAB), flavonoid (luteolin and sakuranetin) and phenylpropanoid (hydroxychavicol). In previous reports, most of the terpenes and phenylpropenes are known to be active against isolates of Candida species [Citation26,Citation27] but certain flavonoids need to be coupled with other commercial drugs to be fully active against the species [Citation28].
Table 2. The MIC of the selected bioactive compounds against seven Candida strains.
Identification of potent inhibitor
Out of the seven Candida strains, only one strain – C. albicans was positive in germination. Candida albicans produced 98% of germinated cells in the induction media within 3 h incubation period. Other strains did not display any morphological changes but showed an increased cell number and grew in budding yeast form. The bioactive compounds that showed antifungal activity in earlier experiment were further subjected to germination inhibition of C. albicans.
IC50 of the antifungal bioactive compounds and CHX on germination were determined as shown in . CHX demonstrated the most effective inhibition activity with the lowest IC50. Among the bioactive compounds, hydroxychavicol showed the lowest IC50.
Table 3. IC50 with 95% CI of the antifungal bioactive compounds (compounds with antifungal activity) and CHX (comparative control) against germination of C. albicans.
Each compound has tended to act as an inhibitor against germination. However, certain compounds such as bakuchiol and PAB have a higher concentration range of IC50 which is greater than the MIC determined. This variance may indicate the capacity of certain compounds in possessing limited and inconsistent inhibitory activity. In order to describe the differences better, a previous study [Citation29] has formulated a calculation based on IC50/MIC ratio. This ratio can be used to determine the potency of each agent in the inhibition of either budding yeast or germination of hyphal growth. It has suggested that an agent preferentially inhibits hyphal growth if the IC50/MIC ratio is less than 1. If the ratio is more than 2, an agent is considered to have low ability to inhibit hyphal growth, but exert their activity by inhibiting the budding yeast growth. In contrast, if the ratio is between 1 and 2 an agent is considered to have approximately equal effects on both activities.
Hydroxychavicol was considered to have higher potency in the inhibition of hyphal growth with ratio below 1 while bakuchiol and PAB were considered to be more potent in inhibiting the growth of yeast bud with ratio more than 2. CHX has an equal inhibition effect on both budding and hyphal growth with ratio between 1 and 2 ().What makes the potency different between the compounds could be possibly derived from their specific action mechanism on the biological target.
Table 4. IC50/MIC ratio and preferential growth inhibition of antifungal bioactive compounds and CHX against C. albicans.
In biological reactions, hydroxychavicol and CHX are capable to alter the cell membrane structure and disrupt the permeability barrier of the membrane [Citation17,Citation30]. Consequently, the injured cells are incapable to perform normal biological activity and indirectly inhibit the germination as well as budding growth. PAB is known to inhibit the synthesis of mitotic spindles, collective spindle fibres formed during mitosis [Citation31]. Mitosis is crucial during cell growth in living yeast, but its process is variable in different growth mode. During budding growth, yeast cells will initially undergo mitosis stage before further buds off daughter from parent cells [Citation32]. In germination, yeast cells will start to germinate before producing elongated tube through mitosis stage [Citation33]. These factors may explain the inefficient activity of PAB against germination. From this observation, it is suggested that distinct mechanisms of the mitosis in yeast growth modes affect the activity of certain compounds.
Hydroxychavicol suppressed germination
Hydroxychavicol exhibited suppression effect on the germination activity of C. albicans. Significant inhibition can be seen within the first hour with 0.4 fold reduction but not in the second and third hour post-treatment with 0.1 fold reduction compared to the untreated sample ().The presence of hydroxychavicol during the brief treatment delayed the process of elongated tube production but then it started to return to its normal biological activity after the first hour post-treatment. Hydroxychavicol may inhibit the growth of cells and cause injury by disrupting the cell membrane [Citation17]. However, the cells are able to recover within a certain period after hydroxychavicol was removed and continue germinating. Since the effect of 2 min exposure was 1 h inhibition, we expected that longer time inhibition could be achieved when the exposure time increased.
Hydroxychavicol caused minimal changes tocellular morphology
The activity of hydroxychavicol was further studied for their effect on the morphological appearance of the germinated cells by using a scanning electron microscope and the result was shown in . For the untreated control sample, it can be concluded that the cells had developed the optimal size, rounded shape and had a smooth surface of the germinated tube. Following 3 h treatment with hydroxychavicol, similar observation was seen in the untreated sample but some of the cells have minimal changes such as constriction at the germ tube neck and had rough surface morphology on the whole surface of its body and tail. Hydroxychavicol may cause slight environmental stress towards cell growth, which would restrict the ions and nutrients uptake mechanisms that normally take place on the cell surfaces. Some hyphal cell wall constituents which are less firmly bound to the rigid glucan-chitin network may be lost during the treatments which then caused uneven cell surface. Previous study has suggested that the loss of a few cell wall components could lead to the loss of structural integrity [Citation34].
Anti-adherence of hydroxyhchavicol
Oral surface comprises of salivary pellicle, a covering protein film that has a selective binding of glycoproteins. Cell membrane of C. albicans has non-polar cell surface proteins and the cells are able to bind to the pellicle through hydrophobic interaction. Rationally, if the cells are in hyphae form, it has more adherence capacity to pellicle layers due to the large surface area of the elongated membrane.
Treatment of the experimental pellicle with hydroxychavicol at IC50 (152 µg/mL) was found to exhibit insignificant reduction in the adhering capacity of the germinated C. albicans strain () with 0.01 fold reduction after the first, the second and the third hour post-treatment. We suggest that hydroxychavicol has poor anti-adherence effects against the germinated C. albicans. It has been described that certain antifungal agents were able to alter the protein receptors on salivary pellicle resulting in failure recognition of Candida cells [Citation35]. Furthermore, physiochemical forces from cell surface hydrophobicity (CSH) of the cells may be implicated in assisting its adherence to treated pellicle. In a previous report, some researchers have suggested that CSH is directly involved in adherence since it eases the binding process of microbes to epithelial cells [Citation36]. In this case, the CSH was increased in germinated cells since the elongated tube has large surface area compared to yeast form. This situation increases the physiochemical forces and strengthens the binding affinity of the cells even to the treated salivary pellicle.
Transcriptional response of RAS1, NRG1 and HWP1 gene to hydroxychavicol
Candida albicans change its morphology from yeast to hyphae through germination process. In untreated condition normal yeast-hyphae transition involved RAS1 gene that works as a signalling transducer for cAMP-PKA pathway [Citation37] being upregulated when the cells being cultured in YPDS [Citation38]. As a result, cAMP signalling pathway is activated. The upregulation of RAS1 has indirectly downregulated the expressions of NRG1, a repressor gene which blocks the transition activity of Candida yeast [Citation39]. Thus, the lowered expression of repressor gene NRG1 has encouraged yeast transition activity and resulted in germination. Changes in the regulatory genes have finally resulted in a higher expression of hyphae specific genes including HWP1. HWP1 is a gene that encodes for a cell wall adhesion protein which helps C. albicans to attach strongly to oral surfaces [Citation40].
In our study, we found that the relative expression of the genes changed with time in hydroxychavicol-treated cells (). During the treatment (0 h), expression of these genes were initially downregulated with 0.68, 0.90 and 0.71 fold changes for RAS1, NRG1 and HWP1, respectively. However, the expression of RAS1 was initially upregulated with 1.40 fold change both within the first and second hour but was then downregulated with 1.0 fold change within the third hour post-treatment; the expression of NRG1 was upregulated within the first hour with 1.40 fold changes but was then downregulated with 1.0 and 0.87 fold changes within the second and third hour post-treatment, respectively. In contrast, expression of HWP1 was downregulated with 0.96 fold change within the first hour and then began to show signs of upregulation with 1.53 and 1.19 fold changes within the second and third hour post-treatment, respectively.
Apart from causing damage to the cell membrane [Citation17], we found that hydroxychavicol-inhibited cell germination by regulating the hyphae-related genes expression (), especially on RAS1 which caused the inhibition to the growth of cells. Since RAS1 plays an important role in cell sustainability [Citation41], this could be the reason why the cells experienced immediate upregulation of RAS1 gene for restoration of its normal physiological condition after the exposure to hydroxychavicol. Within this period, biological processes including cell cycle progression and life span were recovered by upregulation of RASI gene expression [Citation42,Citation43].
Figure 5. Illustration of the suggested mechanism of hydroxychavicol-induced delay of the yeast–hyphae transition in C. albicans. Note: Downregulate (downward paired arrows); upregulate (upward paired arrows); suppress (cross) and promote (tick)
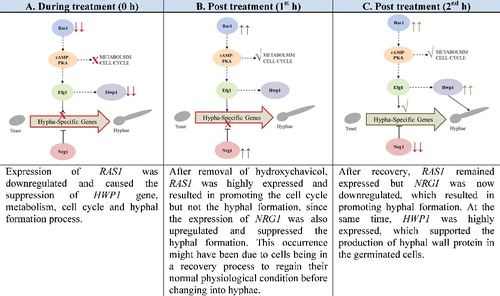
The downregulation of RASI gene expression lead to the inactivation of cAMP-PKA signalling pathway. However, with time, the expression of NRG1 was found to be downregulated and cells begin to regain its ability to germinate. This finding has answered the reason why the number of germinated cells was substantially elevated in the second and third hour after exposure to hydroxychavicol.
HWP1 initially downregulated during treatment but regained to its normal level of expression after the first hour and continued to be upregulated with time. Thus, the cell begins to produce an abundance of hyphal protein to support the growth of hyphae.
Our study has demonstrated that bakuchiol and PAB are antifungal agents against Candida strains but did not act as anti-hyphal agents. However, hydroxychavicol was found to possess anti-hyphal characteristic but was insufficient to eliminate C. albicans from salivary pellicle at dosage of 152 µg/mL. The possible mechanism of hydroxychavicol to inhibit the hyphal growth is by (1) altering the cellular morphology which lead to the unproductive cells and (2) delaying germination process by changing the expression of hyphae-related genes such RAS1, NRG1 and HWP1. Our finding strongly suggests that hydroxychavicol is effective against Candida species such C. albicans and this could support its usage in oral rinse to control and prevent candidal infection especially among target users like cigarette smokers [Citation44] and immunocompromised patients [Citation45].
Conclusions
In this study, we found that hydroxychavicol is one of the identified bioactive compounds that possessed antifungal properties against oral-associated Candida species, potent in inhibition of hyphae development of C. albicans by affecting the morphological and gene regulation. Since hydroxychavicol has poor anti-adherence activity against germinated cells, we recommend further study of the synergistic potential with other bioactive compounds to improve its properties towards preventing colonization of C. albicans in the oral cavity.
Acknowledgments
We are highly gratitude to the Balai Ungku Aziz Research Laboratory (BUARL), Faculty of Dentistry, University of Malaya for research facilities.
Disclosure statement
No potential conflict of interest was reported by the authors.
Additional information
Funding
References
- Pfaller MA, Diekema DJ. Epidemiology of invasive candidiasis: a persistent public health problem. Clin Microbiol Rev. 2007;20(1):133–163.
- Kourkoumpetis T, Manolakaki D, Velmahos G, et al. Candida infection and colonization among non-trauma emergency surgery patients. Virulence. 2010;1(5):359–366.
- Patil S, Rao RS, Majumdar B, et al. Clinical appearance of oral Candida infection and therapeutic strategies. Front Microbiol. 2015 [cited 2015 Dec 17];6:1391. DOI:10.3389/fmicb.2015.01391
- Whiteway M, Bachewich C. Morphogenesis in Candida albicans. Annu Rev Microbiol. 2007;61:529–553.
- Williams D, Lewis M. Pathogenesis and treatment of oral candidosis. J Oral Microbiol. 2011 [ cited 2011 Jan 28]; 3:5771. DOI:10.3402/jom.v3i0.5771
- Douglas LJ. Candida biofilms and their role in infection. Trends Microbiol. 2003;11(1):30–36.
- Yu SJ, Chang YL, Chen YL. Calcineurin signaling: lessons from Candida species. FEMS Yeast Res. 2015[ cited 2015 Apr 15]; 15(4):fov016. DOI:10.1093/femsyr/fov016
- Piispanen AE, Bonnefoi O, Carden S, et al. Roles of Ras1 membrane localization during Candidaalbicans hyphal growth and farnesol response. Eukaryot Cell. 2011;10(11):1473–1484.
- Lu Y, Su C, Liu H. Candidaalbicans hyphal initiation and elongation. Trends Microbiol. 2014;22(12):707–714.
- Orsi CF, Borghi E, Colombari B, et al. Impact of Candidaalbicans hyphal wall protein 1 (HWP1) genotype on biofilm production and fungal susceptibility to microglial cells. Microb Pathog. 2014;69–70:20–27.
- Staab JF, Bradway SD, Fidel PL, et al. Adhesive and mammalian transglutaminase substrate properties of Candida albicans Hwp1. Science. 1999;283(5407):1535–1538.
- Sundstrom P, Balish E, Allen CM. Essential role of the Candida albicans transglutaminase substrate, hyphal wall protein 1, in lethal oroesophageal candidiasis in immunodeficient mice. J Infect Dis. 2002;185(4):521–530.
- Nobile CJ, Nett JE, Andes DR, et al. Function of Candida albicans adhesin Hwp1 in biofilm formation. Eukaryot Cell. 2006;5(10):1604–1610.
- Sharkey LL, McNemar MD, Saporito-Irwin SM, et al. HWP1 functions in the morphological development of Candidaalbicans downstream of EFG1, TUP1, and RBF1. J Bacteriol. 1999;181(17):5273–5279.
- Nantel A, Dignard D, Bachewich C, et al. Transcription profiling of Candida albicans cells undergoing the yeast-to-hyphal transition. Mol Biol Cell. 2002;13(10):3452–3465.
- Aroonrerk N, Dhanesuan N. Candida inhibitory effects of six commercial mouthwashes. Ann Microbiol. 2007;57(3):449–452.
- Ali I, Khan F, Suri K, et al. In vitro antifungal activity of hydroxychavicol isolated from Piper betle L. Ann Clin Microbiol Antimicrob. 2010 [cited 2010 Feb 3];9:7. DOI:10.1186/1476-0711-9-7
- Hsu PJ, Miller JS, Berger JM. Bakuchiol, an antibacterial component of Psoralidium tenuiflorum. Nat Prod Res. 2009;23(8):781–788.
- Hasegawa M, Mitsuhara I, Seo S, et al. Analysis on blast fungus-responsive characters of a flavonoid phytoalexin sakuranetin; accumulation in infected rice leaves, antifungal activity and detoxification by fungus. Molecules. 2014;19(8):11404–11418.
- Sartori MR, Pretto JB, Cruz AB, et al. Antifungal activity of fractions and two pure compounds of flowers from Wedelia paludosa (Acmela brasiliensis) (Asteraceae). Pharmazie. 2003;58(8):567–569.
- Li E, Clark AM, Hufford CD. Antifungal evaluation of pseudolaric acid B, a major constituent of Pseudolarix kaempferi. J Nat Prod. 1995;58(1):57–67.
- Lau K-M, Fu L-H, Cheng L, et al. Two antifungal components isolated from Fructus Psoraleae and Folium Eucalypti Globuli by bioassay-guided purification. Am J Chin Med. 2010;38(5):1005–1014.
- Clinical and Laboratory Standards Institute. Reference method for broth microdilution antifungal susceptibility testing of yeast. Approved standard. 3rd ed. ( CLSI Document M38 A2). Wayne (IL): Clinical and Laboratory Standards Institute; 2008.
- Brayman TG, Wilks JW. Sensitive assay for antifungal activity of glucan synthase inhibitors that uses germ tube formation in Candida albicans as an end point. Antimicrob Agents Chemother. 2003;47(10):3305–3310.
- Sandini S, Stringaro A, Arancia S, et al. The MP65 gene is required for cell wall integrity, adherence to epithelial cells and biofilm formation in Candida albicans. BMC Microbiol. 2011 [ cited 2011 May 16];11(1):106. DOI:10.1186/1471-2180-11-106
- Marcos-Arias C, Eraso E, Madariaga L, et al. In vitro activities of natural products against oral Candida isolates from denture wearers. BMC Complement Altern Med. 2011 [ cited 2011 Nov 26];11:119. DOI:10.1186/1472-6882-11-119
- Carrasco H, Raimondi M, Svetaz L, et al. Antifungal activity of eugenol analogues. Influence of different substituents and studies on mechanism of action. Molecules. 2012;17(1):1002–1024.
- da Silva CR, de Andrade Neto JB, de Sousa Campos R, et al. Synergistic effect of the flavonoid catechin, quercetin, or epigallocatechin Gallate with fluconazole induces apoptosis in Candida tropicalis resistant to fluconazole. Antimicrob Agents Chemother. 2014;58(3):1468–1478.
- Hawser S, Islam K. Comparisons of the effects of fungicidal and fungistatic antifungal agents on the morphogenetic transformation of Candida albicans. J Antimicrob Chemother. 1999;43(3):411–413.
- Komljenovic I, Marquardt D, Harroun TA, et al. Location of chlorhexidine in DMPC model membranes: a neutron diffraction study. Chem Phys Lipids. 2010;163(6):480–487.
- Wong VK, Chiu P, Chung SS, et al. Pseudolaric acid B, a novel microtubule-destabilizing agent that circumvents multidrug resistance phenotype and exhibits antitumor activity in vivo. Clin Cancer Res. 2005;11(16):6002–6011.
- Straight AF, Marshall WF, Sedat JW, et al. Mitosis in living budding yeast: anaphase A but no metaphase plate. Science. 1997;277(5325):574–578.
- Sudbery PE. The germ tubes of Candida albicans hyphae and pseudohyphae show different patterns of septin ring localization. Mol Microbiol. 2001;41(1):19–31.
- de Billerbeck VG, Roques CG, Bessiere JM, et al. Effects of Cymbopogon nardus (L.) W. Watson essential oil on the growth and morphogenesis of Aspergillus niger. Can J Microbiol. 2001;47(1):9–17.
- Nordin MA, Wan Harun WH, Abdul Razak F. An in vitro study on the anti-adherence effect of Brucea javanica and Piper betle extracts towards oral Candida. Arch Oral Biol. 2013;58(10):1335–1342.
- Hazen KC, Brawner DL, Riesselman MH, et al. Differential adherence of hydrophobic and hydrophilic Candida albicans yeast cells to mouse tissues. Infect Immun. 1991;59(3):907–912.
- Biswas S, Van Dijck P, Datta A. Environmental sensing and signal transduction pathways regulating morphopathogenic determinants of Candida albicans. Microbiol Mol Biol Rev. 2007;71(2):348–376.
- Feng Q, Summers E, Guo B, et al. Ras signaling is required for serum-induced hyphal differentiation in Candida albicans. J Bacteriol. 1999;181(20):6339–6346.
- Lu Y, Su C, Wang A, et al. Hyphal development in Candida albicans requires two temporally linked changes in promoter chromatin for initiation and maintenance. PLoS Biol.2011[ cited 2011 July 19]; 9(7):e1001105. DOI:10.1371/journal.pbio.1001105
- Staab JF, Bahn YS, Tai CH, et al. Expression of transglutaminase substrate activity on Candida albicans germ tubes through a coiled, disulfide-bonded N-terminal domain of Hwp1 requires C-terminal glycosylphosphatidylinositol modification. J Biol Chem. 2004;279(39):40737–40747.
- Singh A, Sharma S, Khuller GK. cAMP regulates vegetative growth and cell cycle in Candida albicans. Mol Cell Biochem. 2007;304(1–2):331–341.
- Sun J, Kale SP, Childress AM, et al. Divergent roles of RAS1 and RAS2 in yeast longevity. J Biol Chem. 1994;269(28):18638–18645.
- Toda T, Uno I, Ishikawa T, et al. In yeast, RAS proteins are controlling elements of adenylate cyclase. Cell. 1985;40(1):27–36.
- Gunasegar S, Himratul-Aznita WH. Influence of nicotine on the adherence of Candida albicans ATCC 14053 and Candida parapsilosis ATCC 22019 and expression of selected binding-related genes. Biotechnol Biotechnol Equip. 2017;31(4):807–814.
- Garbee DD, Pierce SS, Manning J. Opportunistic fungal infections in critical care units. Crit Care Nurs Clin North Am. 2017;29(1):67–79.