ABSTRACT
Hepatitis B virus (HBV) infection is a worldwide health problem. More than 400 million people are chronic HBV carriers in the world. Infected individuals are at a high risk of developing liver cirrhosis and hepatocellular carcinoma as the main consequences of HBV. The discoveries of fast diagnostic systems and new therapeutic applications are crucial in the fight against viral hepatitis. In this paper we present the generation of a single-chain variable fragment (scFv) from a mouse monoclonal antibody specific to the HBV surface antigen (HBsAg) and demonstrate its expression as a bacterial alkaline phosphatase (AP) fusion protein. In this study, we constructed scFvs from hybridoma cells expressing HBsAg-specific antibody using phage display technology and expressed them in Escherichia coli. The anti-HBsAg scFvs were inserted into pQE-2 vector to produce scFv antibody genetically fused to bacterial AP. Reproducibility of the recombinant HBsAg-scFv fusion protein was tested using Enzyme-linked Immunosorbent Assay (ELISA). Present preliminary findings indicate that the anti-HBsAg-scFv AP conjugate could be further used for the development of one-step ELISA for the detection of HBV.
Abbreviations | ||
ABTS: | = | with 2,2'-azino-bis (3-ethylbenzothiazoline-6-sulphonic acid); |
AP: | = | alkaline phosphatase; |
BSA: | = | bovine serum albumin; |
CDR: | = | complementarity-determining region; |
DAB: | = | 3,3′-Diaminobenzidine; |
ELISA: | = | Enzyme-linked Immunosorbent Assay; |
EIA: | = | Enzyme immunoassays; |
dNTP: | = | deoxynucleotide triphosphate; |
HBV: | = | hepatitis B virus; |
HBsAg: | = | hepatitis B virus surface antigen; |
HCC: | = | hepatocellular carcinoma; |
HRP: | = | horseradish peroxidase; |
IPTG: | = | isopropyl β-d-thiogalactoside; |
MAb: | = | monoclonal antibody; |
NHS: | = | N-hydroxysulfosuccinimide; |
pNPP: | = | para-Nitrophenylphosphate; |
PBS: | = | phosphate-buffered saline; |
PEG: | = | polyethylene glycol; |
Polymerase chain reaction: | = | PCR; |
SDSC: | = | San Diego Super Computer; |
scFv: | = | single-chain variable fragment; |
SDS PAGE: | = | Sodium Docecyl Sulphate Polyacrylamide Gel Electrophoresis; |
SPR: | = | Surface Plasmon Resonance; |
VH: | = | heavy-chain variable domain; |
VL: | = | light-chain variable domain; |
WHO: | = | World Health Organization |
Introduction
Hepatitis B virus (HBV) is a very important global health problem. World Health Organization (WHO) states that the number of HBV infected people has reached two billion worldwide and approximately 240 million of them have chronic infection. Chronic HBV infection is the primary cause of cirrhosis and hepatocellular carcinoma (HCC). HCC represents more than 5.7% of all cancers and it is the most common primary liver cancer (80%–90%). It is estimated that every year about 500,000–1,000,000 new cases are appearing, causing every year more than 780,000 deaths worldwide [Citation1]. Therefore, the development of economical and accurate detection systems and potential alternative antiviral approaches for HBV detection could be important for fighting with viral hepatitis.
HBV is from the DNA virus family of hepadnavirus. HBV genome is a 3200 base pairs long double-stranded circular DNA. The genome contains four overlapping open reading frames. These encode several viral products: the surface antigen S, the core protein C, protein X, and the DNA polymerase P [Citation2]. The virus is composed of a core particle where the viral genome is encapsulated and an outer lipid-based envelope where coat proteins called HBV surface antigens are embedded. The envelope proteins are involved in viral binding and are released into susceptible cells. The major humoural response to HBV is developed against HBsAg, with the appearance of anti-HBsAg antibodies in the patient's blood, in 6 months after exposure [Citation3].
HBV detection is crucial for an accurate HBV infection diagnosis. Various diagnosis kits were developed and commercialized over the past decade. However, these kits reveal high variability in terms of specificity and sensitivity [Citation4,Citation5]. Also, HBV detection methods differ in developed and developing countries according to the level of endemic HBV infections. Besides, automated HBsAg detection immunoassay systems have been developed and widely used [Citation6–9]. However, high specificity enzyme-linked immunosorbent assays (ELISAs) are still commonly used [Citation4,Citation5,Citation10,Citation11]. Low cost ELISA systems allow fast screening of a large number of samples even though many researches are still focused on increasing the sensitivity and the specificity of HBsAg detection assays [Citation9,Citation12–14]. However, most of them are based on the conventional ELISA system, relying on a cumbersome and time-consuming procedure. One-step ELISA is an alternative way to detect analytes with no need for enzyme-labelled secondary antibodies.
In this paper, we report the generation of single-chain variable fragments (scFv) from hybridoma cell lines (2B7 and 2G3) previously developed by Fatıma Yucel and Aynur Basalp at the Genetic Engineering and Biotechnology Institute of TUBITAK specific to HBsAg [Citation15–17]. The genes encoding the 2B7 and 2G3 antibodies were selectively amplified and used for the generation of the anti-HBsAg scFv genes. The scFvs were expressed first in E. coli as a single molecule, then as a genetically fused scFv-alkaline phosphatase (AP) bifunctional recombinant antibody. The findings reveal promising results regarding the use of a bifunctional anti-HBsAg recombinant antibody for the development of a one-step AP based ELISA detection system for HBV.
Materials and methods
Generation of the scFvs and the display on filamentous phage
The scFvs were generated from two separate hybridoma cell lines, 2B7 and 2G3 [Citation15–17]. Total RNA was extracted using the TRI reagent (Sigma, USA). The scFvs were prepared according to the RPAS ScFv and Expression Modules protocol (Amersham Biosciences Corp., Piscataway, NJ) and cloned into the SfiI/NotI linearized pDUCK1 phagemid. The ScFv/pDUCK1 phagemid was then introduced into E. coli TG1 cells, and rescued with the helper phage M13KO7 (Amersham Biosceinces Corp., Piscataway, NJ) [Citation18,Citation19].
Identification of HBsAg-binding scFvs
ELISA plate wells were coated at 4 °C overnight with 500 ng of HBsAg (equimolar of adw AB91276 and ayw AB73741 subtypes; ABCAM, UK). The next day, the wells were washed with TPBS (0.1% Tween 20 in Phosphate Buffered Saline [PBS]) and blocked for 1 h with TPBS containing 1% bovine serum albumin (BSA). The wells were washed again with TPBS and the 1011 infective phages obtained from the phage rescue were incubated for 2 h at room temperature. Then, the wells were washed again with TPBS. Finally, horseradish peroxidase (HRP) conjugated anti-M13 - antibody (Pharmacia) was added to each well at 1/1000 dilution. The absorbance was measured with an ELISA reader (Bio-Tek ELISA Reader) at 410 nm after 1 h of incubation with 2,2'-azino-bis (3-ethylbenzothiazoline-6-sulphonic acid) (ABTS) substrate solution [Citation20,Citation21].
DNA sequencing reactions were prepared according to the Beckman Coulter GenomeLab Methods Development kit protocol, then the sequencing reactions were run on a CEQ 8800 sequencing system. The nucleic acid sequences of the variable regions of each clone and the Complementarity Determining Region of the scFvs were determined using the ImMunoGeneTics information and V-QUEST analysis system (IMGT; http://imgt.cines.fr/) [Citation22–24].
Soluble expression and characterization of anti-HBsAg scFv
The HBsAg-binding clone was identified by phage ELISA. The phagemid vector was purified and introduced into non-suppressor E. coli HB2151 cells. After 4 h of induction with 1 mmol/L isopropyl β-d-1-thiogalactopyranoside (IPTG), the cells were harvested and soluble scFv was recovered from the bacterial periplasm [Citation25]. The scFv expression was controlled with Coomassie stained 12% Sodium Docecyl Sulphate Polyacrylamide Gel Electrophoresis (SDS PAGE). After the transfer of the ScFv on a nitrocellulose membrane, the membrane was blocked with 1% Bovine Serum Albumin (BSA) in PBS. The detection was made with a mouse anti-c-myc antibody followed by an anti-mouse HRP conjugate. The colour reaction was determined with the 3,3′-Diaminobenzidine (DAB)/HRP system.
The binding assay was done using ELISA. Each well was coated with various amounts of recombinant HBsAg (equimolar of adw AB91276 and ayw AB73741 subtypes; ABCAM, UK) or purified HBsAg from human serum, ranging from 10 to 1000 ng in bicarbonate buffer (pH 9.5) at 4 °C overnight. The wells were washed the next day three times with TPBS (0.1% Tween-20 in PBS). The room temperature blocking in one hour was measured with blocking buffer (PBS + 1% BSA). The wells were then washed and 1 µg of anti-HBsAg recombinant antibody was incubated for 2 h at room temperature. HRP-conjugated anti-myc tag antibody (1:5000; Sigma) was added into the wells washed. After 1 h of incubation at room temperature, colour reaction was determined with ABTS substrate solution. The OD405 was measured with an ELISA reader (Bio-Tek) after 1 h of incubation at room temperature.
Reichert SPR instrument (Reichert Instrument, USA) and sensor chips were used for the assays. The temperature during the assays was 25 °C and the flow rate of the buffer was 100 µL/min. The immobilization of His-tagged anti-HBsAg scFv was achieved by physical adsorption of the scFv on bare Au SPR chips with 4-min injection of 0.3 µmol/L scFv. The surfaces of the scFv-coated chips were washed with buffer solution (100 m mol/L NaH2PO4, 10 m mol/L Tris-Cl and pH 8.0) until equilibration. Later, HBsAg binding to His-tagged anti-HBsAg scFv-immobilized surfaces was achieved by the injection of HBsAg at various concentrations (150 n mol/L, 200 n mol/L, and 300 n mol/L) (equimolar of adw AB91276 and ayw AB73741 subtypes; ABCAM, UK) for 4 min. SPR measurements were analyzed with BIAevaluation Software 4.1 (Biacore, Uppsala, Sweden)
Generation and expression of the scFv AP conjugate
Lig7 _AP
The E. coli AP gene was extracted from the pSB3057 (AP-5GBP) vector by Polymerase chain reaction (PCR) using the AP_Not_F and AP_R_Hind_III primers (5′-CACGTGCGGCCGCAGTTCTGGAAAACCGGGCTGCTCAGG-3′ and 5′-TGACTCGCAAGCTTGTTATTTCAGCCCCAGAGCG-3′, respectively). The amplified product (1.2 kb) was purified and double digested with NotI and HindIII restriction enzymes. The digested PCR product was inserted into the double digested pQE2 vector. The Lig7 anti-HBsAg scFv was amplified using 2 oligonucleotides containing NdeI and NotI restriction enzyme recognition sites (underlined): TUB_721_NdeI (5′-TCGCCATATGCAGGCCCAGGTGCAGCTGCAGGAGTCAGG-3′) and Lig_7_Reverse_Not_I (5′-GAGTCATTCTGCGGCCGCCCGTTTGATTTCCAGCT TGG-3′) and digested with the appropriate restriction enzymes. The digested product was then inserted into the NdeI/NotI-digested pQE2/AP vector [Citation26].
Lig7_Linker_AP
Cloning of the AP gene
The AP gene was amplified in terms of PCR, using the primers AP_Forward (5′-GAGTCATTCTGCGGCCGCAGTTCTGGAAAACCGGGC-3′) and AP_Reverse (5′-TGACTCGCAAGCTTGTTATTTCAGCCCCAGAGCG-3′). The restriction sites for NotI and HindIII were underlined in the primer sequences. The PCR reaction was prepared in 50 μL including 1 × reaction buffer, 2.5 m mol/L MgCl2, deoxynucleotide triphosphate (dNTP; 10 m mol/L each), 20 pmol of each primers, 200 ng of plasmid pSB3057 (AP-5GBP) [Citation27] and Taq DNA polymerase (1 U; Roche Molecular Biochemicals, Mannheim, Germany). The reaction was run in the T3000 thermal cycler (Biometra, Goettingen, Germany) for 30 cycles (1 min at 94 °C, 2 min at 55 °C, 2 min at 72 °C), with a final elongation step of 10 min. at 72 °C. The PCR fragments NotI and HindIII were double digested and purified with High Pure PCR Purification Kit (Roche). The PCR fragments were inserted into pQE2 vector (Qiagen, Hilden, Germany) in a ligation reaction mixture (20 μL) containing 2 μL of 10 × ligase buffer and 2.5 U T4 DNA ligase (Fermentas, Helsingborg, Sweden) at 16 °C overnight. E. coli BL21 (DE3) cells were transformed with the ligation product through CaCl2 transformation method [Citation28]. The insertion was examined with colony PCR method and DNA sequence analyses. The new vector was called pQE2/AP.
Cloning of the Lig7_linker gene
The Lig7 scFv gene including a DNA sequence that encodes the linker ‘ASGAEGGGSEGGGSG’ [Citation29] was cloned into the pQE2/AP vector. Lig7 was amplified by using Lig7_Forward and Lig7_Reverse_Linker primers (5′-TCGCCATATGCAGGCCCAGGTGCAGCTGCAGGAGTCAGG-3′ and 5′-AGAACTGCGGCCGCGCCAGAGCCACCACCCTCAGAGCCACCACCCTCAG CGCCAGAAGCCCGTTTGATTTCC-3′, respectively). After the double digestion of the PCR amplification product with NdeI and NotI restriction enzymes, the insert was cloned into the pQE2/AP vector following the same protocol as the one described above. The insertion was examined with colony PCR method followed by a DNA sequence analysis.
To produce the Lig7_AP and Lig7_linker_AP fusion proteins, the pQE2_Lig7_AP and pQE2_Lig7_linker_AP vectors were introduced into E. coli BL21 (DE3) cells using the Calcium Chloride (CaCl2) transformation method [Citation28]. Individual colonies were picked and grown overnight in ampicillin (100 µg/mL) containing 2 × YT medium at 30 °C. Then the pre-culture was inoculated into a fresh culture medium and incubated at 37 °C until OD600 reached 0.6. The culture was induced with 1 mmol/L IPTG, for 4 h at 30 °C [Citation30]. The culture was harvested by centrifugation at 2400 g for 10 min; the supernatants were analyzed by ELISA and the pellets were analyzed by SDS-PAGE and western blotting.
Binding assay and biological activity of the bifunctional recombinant antibody
ELISA plate wells were coated with the target antigen (500 ng) at 4 °C overnight. The next day the wells were washed three times with TPBS (0.1% Tween-20 in PBS). Blocking buffer (PBS + 1% BSA) was used for 1 h at room temperature for blocking the wells. After washing with TPBS, 100 µL of Lig7_linker_AP fusion protein extract was incubated at room temperature for 2 h. HRP-conjugated anti-His-tag conjugate (1:5000; Sigma) was incubated at room temperature for 1 h after the washing step. ABTS substrate was used to initiate a colour reaction (Pharmacia Biotech). After the absorbance at 405 nm, (A405) was measured with an ELISA reader (Bio-Rad) after one hour.
Varying volumes of culture medium from 10 µL to 100 µL containing the bifunctional recombinant antibodies were mixed with 100 µL of para-Nitrophenylphosphate (pNPP) substrate (1 mg/mL in 0,1 mol/L glycine, 1 mmol/L Magnesium chloride, 1 mmol/L Zinc chloride, and pH10.4 buffer) and incubated for 30 min at 37 °C. The activity of the enzyme was monitored with an ELISA reader at 405 nm.
Results and discussion
Despite worldwide vaccination programs, HBV continues to be a major cause of morbidity and mortality. HBV infection is classified according to the serologic markers or patterns, where each marker has a specific clinical significance [Citation31]. There are 5 serologic markers, including HBsAg, HBe antigen (HBeAg), antibody to HBsAg (anti-HBs), antibody to HBeAg (anti-HBe), and antibody to HBc antigen (anti-HBc), which are valuable for monitoring a HBV infection and vaccination efficacy. However, the first serologic marker that can be detected in the serum is the HBsAg which appears in 1–12 weeks after infection. The presence of HBsAg indicates that the person is infectious. Therefore, HBsAg is the primary target for the detection of HBV infection. There are many antibodies developed against HBsAg up to now, however the smaller the recognition molecules (such as single-chain variable fragments [scFv]), the more accurate the detection of a recombinant antibody, especially when fusion proteins are used for detection [Citation32,Citation33]. ScFvs are recombinant antibodies generated by the fusion of antibodies with variable heavy (VH) and variable light (VL) chains of immunoglobulins connected with a linker [Citation34,Citation35]. Because of its small size (∼25-35 kDa) and the fact that the scFv is a single-chain antibody, it is possible to fuse it easily with other proteins or toxins [Citation36].
Generation of scFvs and the display on filamentous phage
Two different recombinant antibodies were produced from the VH and VL encoding regions of two different hybridoma cell lines (2G3 and 2B7) expressing monoclonal antibodies against HBsAg. The VH and VL chains were linked together with a flexible (Gly4Ser) 3 linker. Each step of PCR amplification was controlled by Agarose gel electrophoresis (). The PCR amplification products of the VH and VL genes revealed a band size of ∼340 bp and ∼325 bp, respectively. The scFv size was ∼750 bp as expected. The scFv encoding genes were then cloned into the pDUCK1 phagemid vector and transferred into TG1 E. coli cells. The cloning experiment was examined with colony PCR method (data not shown).
Figure 1. ScFv development. Photographs of agarose gel showing VH (heavy chain), VL (light chain) and scFv (single-chain variable fragment). (A) Lane 1, pUC19 HinfI digestion marker (fragment sizes: 1419 bp, 517 bp, 396 bp, and 214 bp); lane 2, VL gene amplicon (325 bp); lane 3, VH gene amplicon (340 bp); (B) Lane 1, scFv gene amplicon (750 bp); lane 2, pUC19 HinfI digestion marker.
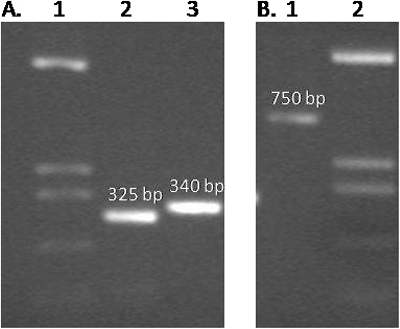
Identification of HBsAg-binding scFvs
Truncations and mutations may occur during the PCR and cloning steps. Therefore, we used phage display technology for selecting target specific clones and removing the mutant ones. We displayed the ScFvs on the surfaces of infectious phages by means of M13KO7 helper phage. After one round of enrichment with biopanning process, we randomly selected 30 scFv clones from each of the two groups derived from 2B7 and 2G3 hybridoma cell lines. All the clones were tested for their binding feature to HBsAg by ELISA. We detected that the numbers of HBsAg binders were respectively eleven and seventeen in the 2B7 and 2G3 hybridoma cell line derived ScFv groups. We employed DNA fingerprinting of PCR products with BstNI restriction enzyme digestion in order to determine the sequence diversity of the clones. We selected two HBsAg-specific clones exhibiting the highest reactivity to HBsAG; which are the 2B7F scFv from the 2B7 hybridoma cell line and the Lig7 scFv from the 2G3 hybridoma cell line.
The genes that encode the Lig7 and the 2B7F scFvs were sequenced by IONTEK company, Turkey. The DNA sequences, including the complementarity-determining regions (CDR), were analyzed at the IMGT web site, and the sequences are presented in and . The Lig7 and 2B7F scFvs are 720 bp and 711 bp long, respectively. The CDR of the two scFv sequences differ from the other CDR sequences on the database according to BLAST search and V-QUEST sequence analysis.
Figure 2. Sequences of VH and VL in Anti-HBsAg 2B7 scFv. Nucleotide and amino acid sequences of VH and VL in the anti-HBsAg 2B7 scFv. Complementarity-determining regions (CDRs) are represented with bold and italic fonts.
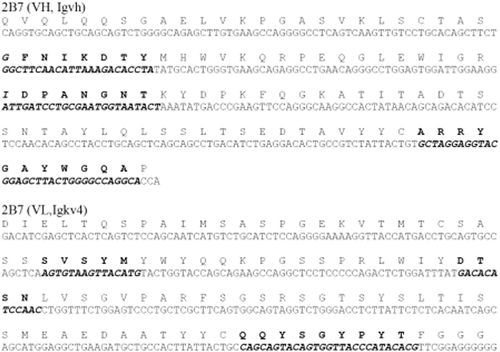
Figure 3. Sequences of VH and VL in Anti-HBsAg 2G3 scFv. Nucleotide and amino acid sequences of VH and VL in the anti-HBsAg 2G3 scFv. Complementarity-determining regions (CDRs) are represented with bold and italic fonts.
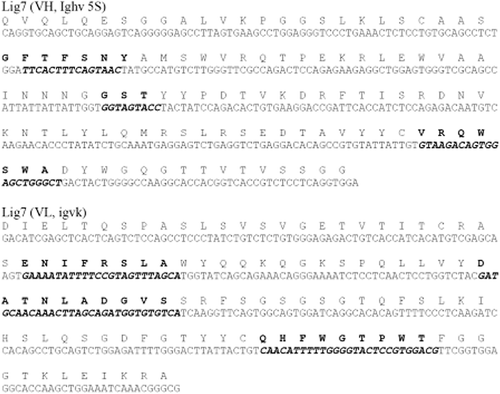
V-QUEST analyses on the variable regions of the both scFvs revealed that the light chain of the the Lig7 scFv matches with the identity of the IGKV12-46*01 F allele by 93.9%, and the heavy chain matches with the identity of Mus musculus IGHV5-6-2*01 and Mus musculus IGHV5-6-3*01 F by 92%. Therefore, the light chain of the Lig7 scFv is from the Kappa-V12 subclass and the heavy chain from the IgG-V5 subclass. The light chain of the 2B7F scFv matches with the identity of the Mus musculus IGKV4-62*01 by 97.46% and the heavy chain matches with the identity of Mus musculus IGHV14-3*02 by 99.31%. Therefore, the light chain of 2B7F scFv is from the Kappa-V4 subclass and the heavy chain from the IgG-V14 subclass. The canonical CDR classes of the Lig7 and 2B7F scFvs were predicted through the Web Antibody Modelling web page. The predictions revealed that CDR-L2, CDR-L3, and CDR-H1 are from the type-1 canonical class, CDR-L1 from the class 2, CDR-H2 from the class 3; and the 7-residue-long CDR-H3 has a kinked profile. The CDR analysis of the 2B7F scFv revealed that CDR-L1, CDR-L2, CDR-L3 and CDR-H1 are from the type-1 canonical class, CDR-H2 from the class 2, and the 11-residue-long CDR-H3 has a kinked profile.
The comparison of the Lig7 scFv CDRs with eight different recombinant antibodies generated against HBsAg revealed that CDR-H2 and CDR-L3 have a weak identity similarity to antibodies (1F6 and F124). IgBlast search revealed no sequence similarity to other anti-HBsAg antibodies. The Lig7 scFv is thus a totally new and different anti-HBsAg recombinant antibody. The Lig7 scFv has been selected for further experiments.
Soluble expression and characterization of anti-HBsAg scFv
The Lig7 scFv was expressed in the non-suppressor host E. coli HB2151 cell line. After His-tag affinity purification, the molecular weight of the scFvs was measured to be ∼33 kDa by SDS-PAGE and western blot analysis (). The binding capacity of Lig7 scFv to two different HBsAg was analyzed by ELISA (). The binding capacity of Lig7 scFv to the HBsAg purified from human serum was slightly better than that of the recombinant one produced in Pichia pastoris. The difference in the binding profiles might result from the antigenic heterogeneity of HBsAg.
Figure 4. Expression of the anti-HBsAg scFv. SDS-PAGE (A) and western blot (B) analyses showing the expression of anti-HBsAg scFv in soluble form. Lane 1, molecular mass marker (19, 26, 34, 48, 85, 117 kDa); lane 2, non-induced culture supernatant; lane 3, induced culture; lane 4, periplasmic extract; lane 5, affinity-purified Lig7 measured to be ∼33 kDa.
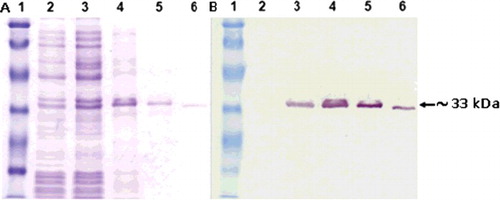
Figure 5. ELISA assay. Binding capacity of the Lig7 scFv to human-serum-purified HBsAg and mouse recombinant HBsAg analyzed by ELISA.
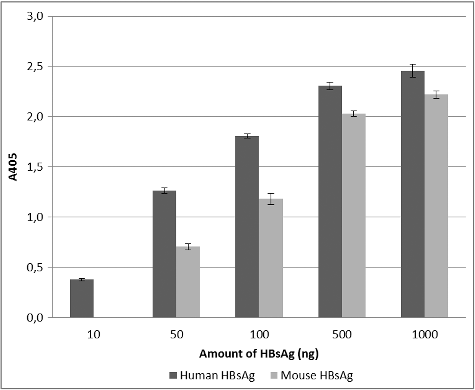
The binding affinity of Lig7 scFv for HBsAg was determined by SPR spectroscopy. The binding was measured as response units; and the results were analyzed with the Langmuir binding model using BIAevaluation software 4.1 ((A). According to the SPR measurements, an affinity constant (KD) of 31.4 ± 21.4 nmol/L was determined for Lig7 scFv binding to HBsAg. The affinity of Lig7 to HBsAg is much higher than the affinity of the anti-HBsAg scFv (23 μM) developed by Xiong [Citation37]. When compared to monoclonal antibodies developed against HBsAg, Lig7 scFv is within the range of high HBsAg affinity [Citation38,Citation39]. Therefore, as a single-chain variable fragment, the Lig7 clone has the potential to be used for diagnostic applications.
Figure 6. Binding dynamics of the bifunctional scFvs (LAL13 and RANA clones). (A) Surface plasmon resonance sensorgram. Affinity kinetics of various concentrations of HBsAg (150 nM, 200 nM, and 300 nM) on Lig7 scFv. (B) SDS-PAGE (a) and western blot (b) analyses of LAL13 and RANA clones. Lane 1, molecular mass marker (in kDa); lane 2, Lig7 scFv; lane 3, LAL13 clone; lane 4, RANA clone. (C) HBsAg-binding capacities of LAL13 clone, RANA clone and Lig7 scFv. (D) Alkaline phosphatase activity of LAL 13 and RANA clones. The activity was analyzed with ELISA Reader at 405 nm.
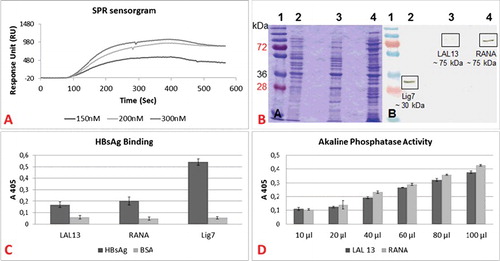
Generation and expression of the scFv AP conjugate
The 1200 bp E. coli alkaline phosphatase gene (AP) amplified from the plasmid pSB3057 (AP-5GBP) was cloned into the pQE2 expression vector by PCR. The cloning experiment was examined with colony PCR method first, then with DNA sequence analysis. Once the AP gene sequence was confirmed, the Lig7 scFv was inserted into the new vector either by direct insertion or by addition of a linker between two proteins. The sequences of the new clones ‘Lig7_AP/pQE2 (RANA)’ and ‘Lig7_linker_AP/pQE2 (LAL13)’ were checked for mutation and frameshift by DNA sequence analysis.
Lig7 scFv, LAL13 and RANA fusion antibodies were expressed in E. coli BL21 (DE3) cells and extracted from the cells by treatment with sucrose followed by a buffer with MgSO4. We obtained 294 µg of Lig7, 808 µg of RANA and 207 µg of LAL out of a culture of 250-mL after His-tag affinity purification. With SDS-PAGE and western blot analysis, the molecular weight of Lig7 scFv was confirmed to be ∼33 kDa and the molecular weight of LAL13 and RANA to be ∼75 kDa ((B).
Binding and biological activity of the bifunctional recombinant antibody
The binding capacities of the Lig7, the LAL13 and RANA clones to HBsAg were measured by ELISA ((C). The LAL13 and RANA clones’ HBsAg-binding signals (A405) were 0.17 and 0.2, respectively; which denote significance compared to the cross-reactivity results of Bovine Serum Albumin by 0.06 and 0.048, respectively. However, the HBsAg-binding signal of the Lig7 scFv itself was 0.540. The decrease in the binding capacities of LAL13 and RANA clones might have resulted from the steric hindrance effect which is probably caused by the fusion between the Lig7 scFv and AP. To avoid such conformational problems, we added the ‘ASGAEGGGSEGGGSG’ linker between the scFv and AP [Citation40]. The results show that this linker has no significant effect on the binding capacity of Lig7 to HBsAg. Therefore, it would be more appropriate to use another linker with a different or longer sequence, as mentioned by Gu et al. [Citation41].
The alkaline phosphatase activities of the bifunctional scFvs were tested with the addition of the pNPP substrate. The activity signal of the Alkaline Phosphatase increased in correlation with the increase in the amount of the bifunctional antibody ((D). Therefore, the fusion strategy was successful.
Serologic tests for the diagnosis of HBV infection require the detection of several HBV-specific antigens and their host antibodies [Citation42–44]. Thanks to the researches and improvements in the field, the detection of serological markers is becoming easier day by day in terms of detection period and labour intensity. Today, the detections can be made by fully automated processes [Citation45]. However, colourimetric detection methods consist of chemical couplings of enzymes, such as HRP and AP. By using an appropriate substrate solution, a colour reaction is initiated with the presence of enzymes [Citation46,Citation47]. These methods are labour intensive, are prone to handling errors and require long detection periods. Therefore, alternative methods are required.
Most of antibody conjugates are chemically coupled. Chemical coupling may harm proteins’ functions or simply block the active site of either the antibody or the conjugated protein [Citation48]. Therefore, non-chemical coupling methods have great advantages. Genetically-fused proteins constitute a good example for antibody conjugate generation. Genetically-fused antibody conjugates eliminate a number of experimental steps in ELISA, so that ELISA can be done in a single step. The potential use of this method has been shown with various examples [Citation49].
Conclusions
In this study, we report the successful development of a new anti-HBsAg scFv which is genetically fused with the AP gene for the detection of HBsAg. We generated and analyzed two single-chain antibodies (Lig7 and 2B7F) from hybridoma cells expressing HBsAg-specific antibodies. ELISA results show that the Lig7 and 2B7F scFvs differ from each other with respect to HBsAg binding capacity. We also described the production of an anti-HBsAg/Alkaline phosphatase bifunctional scFv in E. coli. Furthermore, we report the HBsAg-binding capacity of the bifunctional antibody and the AP activity determined by ELISA. Preliminary data suggest that our anti-HBsAg scFvs have the potential to be used in one-step ELISA after further linker optimization studies.
Disclosure statement
No potential conflict of interest was reported by the authors.
Additional information
Funding
References
- World Health Organization. 2017. Available from: http://www.who.int/mediacentre/factsheets/fs204/en/, Hepatitis B, Fact sheet. Accessed June.
- Malik AH, Lee WM. Chronic hepatitis B virus infection: treatment strategies for the next millennium. Ann Intern Med. 2000;132:723–731.
- Bose B, Chugh DA, Kala M et al. Characterization and molecular modeling of a highly stable anti-hepatitis B surface antigen scFv. Mole Immunol. 2003;40(9):617–631.
- Scheiblauer H, El-Nageh M, Diaz S, et al. Performance evaluation of 70 hepatitis B virus (HBV) surface antigen (HBsAg) assays from around the world by a geographically diverse panel with an array of HBV genotypes and HBsAg subtypes. Vox Sang. 2010;98(3):403–414.
- Huh HJ, Chae SL, Cha YJ. Comparison study with enzyme immunoassay and chemiluminescence immunoassay for hepatitis B virus surface antigen detection. Korean J Lab Med. 2007;27:355–359.
- Chen Y, Wu W, Li LJ, et al. Comparison of the results for three automated immunoassay systems in determining serum HBV markers. Clin Chim Acta. 2006;372:129–133.
- Ismail N, Fish GE, Smith MB. Laboratory evaluation of a fully automated chemiluminescence immunoassay for rapid detection of HBsAg, antibodies to HBsAg, and antibodies to hepatitis C virüs. J Clin Microbiol. 2004;42:610–617.
- Miedouge M, Legrand-Abravanel F, Lalanne C, et al. Laboratory evaluation of the UniCel DxI 800 analyser (Beckman Coulter) for detecting HBV and HCV serological markers. J Clin Virol. 2009;44:134–137.
- Mühlbacher A, Weber B, Bürgisser P, et al. Multicenter study of a new fully automated HBsAg screening assay with enhanced sensitivity for the detection of HBV mutants. Med Microbiol Immunol. 2008;197(1):55–64.
- Acar A, Kemahli S, Altunay H, et al. The significance of repeat testing in Turkish blood donors screened with HBV, HCV and HIV immunoassays and the importance of S/CO ratios in the interpretation of HCV/HIV screening test results and as a determinant for further confirmatory testing. Transfus Med. 2010;20(3):152–159.
- Ziraba AK, Bwogi J, Namale A, et al. Sero-prevalence and risk factors for hepatitis B virus infection among health care workers in a tertiary hospital in Uganda. BMC Infect Dis. 2010;10:191.
- Moerman B, Moons V, Sommer H, et al. Evaluation of sensitivity for wild type and mutant forms of hepatitis B surface antigen by four commercial HBsAg assays. Clin Lab. 2004;50:159–162.
- Weber B, Dengler T, Berger A, et al. Evaluation of two new automated assays for hepatitis B virus surface antigen (HBsAg) detection: IMMULITE HBsAg and IMMULITE 2000 HBsAg. J Clin Microbiol. 2003;41:135–143.
- Lin YH, Wang Y, Loua A, et al. Evaluation of a new hepatitis B virus surface antigen rapid test with improved sensitivity. J Clin Microbiol. 2008;46(10):3319–3324.
- Yücel F, Çırakoğlu B. MAbs against hepatitis B surface antigen. Hybridoma. 2000;19:501–502.
- Başalp A. Monoclonal antibodies against hepatitis B virus surface antigen. Hybridoma Hybridomics. 2002;21(1):81–82.
- Yücel F, Manav Aİ, Başalp A. Production and characterization of monoclonal antibodies against hepatitis B viruses and application of a quick sandwich ELISA. Hybrid Hybridomics. 2003;22:173–177.
- Bajrovic K, Erdag B, Atalay OE, et al. Full resistance to tobacco mosaic virus infection conferred by the transgenic expression of a recombinant antibody in tobacco. Biotechnol Biotechnol Equip. 2001;15(1):21–27.
- Erdag B, Balcioglu BK, Ozdemir Bahadir A, et al. Identification of novel neutralizing single-chain antibodies against vascular endothelial growth factor receptor 2. Biotechnol Appl Biochem. 2011;58(6):412–422.
- Bahadir AO, Balcioglu BK, Uzyol KS et al. Phage displayed HBV core antigen with immunogenic activity. Appl Biochem Biotechnol. 2011;165:1437–1447.
- Atalay EÖ, Erdag B, Çırakoglu B. The use of Polypeptide Probes selected from Artificial Peptide libraries for the recognation and differentiation of DNA sequences. Tr J Med Sci. 1999;29:349–354.
- Ruiz M, Lefranc MP. IMGT gene identification and Colliers de Perles of human immunoglobulins with known 3D structures. Immunogenetics. 2002;53:857–883.
- Kaas Q, Ehrenmann F. Lefranc MP, IG, TR and IgSF, MHC and MhcSF: what do we learn from the IMGT Colliers de Perles? Brief Funct Genomic Proteomic. 2007;6:253–264.
- Ehrenmann F, Giudicelli V, Duroux P, et al. IMGT/Collier de Perles: IMGT standardized representation of domains (IG, TR, and IgSF variable and constant domains, MH and MhSF groove domains). Cold Spring Harbor Protoc. 2011;6:726–736.
- Kala M, Bajaj K, Sinha S. Direct antigen capture by soluble scFv antibodies. A method for detection, characterization, and determination of affinity. Appl Biochem Biotechnol. 2001;90(1):11–22.
- Balcioglu BK. Ozdemir-Bahadir A, Hinc D, et al. Cost effective filamentous phage based immunization nanoparticles displaying a full-length hepatitis B virus surface antigen, Adv Biosci Biotechnol. 2014;05:46–53.
- Kacar T, Zin MT, So C, et al. Directed self-immobilization of alkaline phosphatase on micro-patterned substrates via genetically fused metal-binding peptide. Biotechnol Bioeng. 2009;103(4):696–705.
- Sambrook J, Fritsch EF, Maniatis T. Molecular Cloning: a laboratory manual. 2nd ed. New York (NY): Cold Spring Harbor Laboratory Press; 1989, p. 1659.
- Lindner P, Bauer K, Krebber A, et al. Specific detection of his-tagged proteins with recombinant anti-His tag scFv-phosphatase or scFv-phage fusions. Bio. Techniques. 1997;22:140–149.
- Sánchez L, Ayala M, Freyre F, et al. High cytoplasmic expression in E. coli, purification, and in vitro refolding of a single chain Fv antibody fragment against the hepatitis B surface antigen. J Biotechnol. 1999;72:13–20.
- Custer B, Sullivan SD, Hazlet TK, et al. Global epidemiology of hepatitis B virus. J Clin Gastroenterol. 2004;38:S158–S168.
- Siegemund M, Richter F, Seifert O, et al. Expression and purification of recombinant antibody formats and antibody fusion proteins. Monoclonal Antibodies, Methods Mole Biol. 2014;1131:273–295.
- Kierny MR, Cunningham TD, Kay BK. Detection of biomarkers using recombinant antibodies coupled to nanostructured platforms. Nano Rev. 2012;3:17240 DOI:10.3402/nano.v3i0.17240.
- Xu L, Tang WH, Huang CC, et al. Systemic p53 gene therapy of cancer with immunolipoplexes targeted by anti-transferrin receptor scFv. Mol Med. 2001;7:723–734.
- Boado RJ, Ji A, Pardridge WM. Cloning and expression in Pichia pastoris of a genetically engineered single chain antibody against the rat transferrin receptor. J Drug Target. 2000;8:403–412.
- Rathore D, Batra JK. Construction, expression and characterization of chimaeric toxins containing the ribonucleolytic toxin restrictocin: intracellular mechanism of action. Biochem J. 1997;324(3):815–822.
- Sheng X, Xiangrong R, Xing Y, et al. Purification of an anti-HBsAg scFv and measurement of its affinity constant. Chin J Pathophysiol, 2002;18(9):1069–1073.
- Tan W, Meng Y, Li H, et al. A bispecific antibody against two different epitopes on hepatitis B surface antigen has potent hepatitis B virus neutralizing activity. 2013;5:946–955.
- Shin YW, Ryoo, KW Hong, et al. Human monoclonal antibody against Hepatitis B virus surface antigen (HBsAg). Antiviral Res. 2007:75;113–120.
- Seo HD, Lee J, Kim YJ, et al. Alkaline phosphatase-fused repebody as a new format of immuno-reagent for an immunoassay. Analytica Chimica Acta. 2017;950:184–191.
- Gu X, Jia X, Feng J, et al. Molecular modeling and affinity determination of scFv antibody: proper linker peptide enhances its activity. Ann Biomed Eng. 2010;38(2):537–554
- Hoogenboom HR, Griffiths AD, Johnson KS, et al. Multi-subunit proteins on the surface of filamentous phage: methodologies for displaying antibody (Fab) heavy and light chains. Nucl Acids Res. 1991;19:4133–4137.
- Mahoney FJ. Update on diagnosis, management, and prevention of hepatitis B virus infection. Clin Microbiol Rev. 1999;12:351–366.
- Trépo C, Chan HL, Lok A. Hepatitis B virus infection. The Lancet. 2014;384(9959):2053–2063.
- Pérez D, Martínez-Flores JA, Serrano M, et al. Evaluation of three fully automated immunoassay systems for detection of IgA anti-beta 2-glycoprotein I antibodies. Int Jnl Lab Hem. 2016 Oct;38(5):560–568.
- Avrameas S, Ternynck T, Guesdon JL. Coupling of enzymes to antibodies and antigens. Scand J Immunol. 1978;8(7):7–20.
- Yücel F, Manav AI, Başalp A. Production and characterization of monoclonal antibodies against hepatitis B viruses and application of a quick sandwich ELISA. Hybridoma Hybridomics. 2003;22(3):173–177.
- Tanf DF, Zhu HF, Wang ZH, et al. Construction of single chain Fv antibody against transferrin receptor and its protein fusion with alkaline phosphatase. World J Gastroenterol. 2005 Jun 7;11(21):3300–3303.
- Rau D, Kramer K, HockJ B. Single-chain Fv antibody-alkaline phosphatase fusion proteins produced by one-step cloning as rapid detection tools for ELISA. J Immunoassay Immunochemistry. 2002;23(2):129–143.