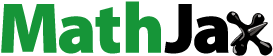
ABSTRACT
Heparinase has an important application in the preparation of low-molecular-weight heparins and the deheparinization of heparin-treated blood. To increase the soluble expression of heparinase I (HepI) in recombinant Escherichia coli, the hepA gene (coding for HepI) from Flavobacterium heparinum was obtained through chemical synthesis and fused with the gene encoding hexahistidine, small ubiquitin-like modifier (SUMO), a flexible peptide linker (G4S) and a bovine enterokinase site (D4K). The constructed fusion protein (SUMO–HepI) was expressed in E. coli BL21 (DE3), and then purified with Ni2+-chelating affinity chromatography. The fermentation conditions were optimized and the enzymatic properties were also analyzed. As the results showed, the recombinant SUMO–HepI was successfully expressed in E. coli BL21 (DE3) and purified with affinity chromatography. The recombinant protein reached the highest soluble expression when the expression strain was induced by 0.6 mmol/L isopropyl β-D-thiogalactoside and grown at 30 °C with a shaking speed of 150 r/min for 9 h. The fusion protein could exhibit high enzyme activity without requirements of in vitro refolding and SUMO-tag releasing process, and the optimum enzyme activity was obtained at 30 °C, pH 7.0 and 10 mmol/L Ca2+ in the reaction buffer. This work provided a novel simple approach for the soluble expression of HepI in E. coli, and might establish a foundation for the following production and application of HepI in the industry.
Introduction
Heparinase I (HepI) is a type of bacterial enzyme that is a powerful tool in the study of the physiological roles of heparin-like complex polysaccharides. Moreover, it can cleave heparin to produce low-molecular-weight heparin (LMWH) that can retain the anticoagulant properties. Therefore, HepI is valuable in the production of LMWH, which is widely used in the prevention and treatment of venous thromboembolism (deep vein thrombosis and pulmonary embolism) and in the treatment of myocardial infarction [Citation1,Citation2].
Natural HepI is mainly produced by Flavobacterium heparinum. Since the yield of HepI in F. heparinum is low, recombinant expression of HepI using genetic engineering strategies has received widespread attention. However, HepI expressed in Escherichia coli easily aggregates into insoluble inclusion bodies in an inactive form and is difficult to refold [Citation3]. To solve this problem, fusion expression with a tag which has ability to improve the formation of correct conformation is a useful approach for the soluble expression of this protein [Citation4].
In the present study, in order to establish a soluble expression system of HepI, the hepA gene (coding for HepI) was fused with a hexahistidine-tag (6 × His) and genes encoding a small ubiquitin-like modifier (SUMO), a flexible peptide linker (G4S) and a bovine enterokinase site (D4K), and then the expression and purification conditions were optimized and the enzyme activity of the recombinant product was characterized.
Materials and methods
Strains, plasmids and reagents
E. coli DH5α from a culture collection was used as the host for subcloning and plasmid amplification. E. coli BL21 (DE3) from a culture collection was used as the expression host. pE-SUMO with a 6 × His tag and a SUMO tag at the upstream of the multiple cloning sites was used as the expression vector. Heparin (140 IU/mg) was purchased from Solarbio company (Beijing, China).
Construction of hepA fusion vectors
As the efficiency of heterologous protein production in E. coli can be diminished by biased codon usage, to increase the expression of target protein, the coding sequence of F. heparinum hepA was optimized according to the codon usage table of E. coli (http://www.kazusa.or.jp/condon/) and was synthesized by Genewiz Beijing company [Citation5]. The hepA with a flexible peptide linker (G4S) sequence and a bovine enterokinase site (D4K) sequence at the 3’ end was then amplified using overlapping polymerase chain reaction (PCR). The resultant PCR products were purified, digested with BsaI and BamHI, and ligated into the BsaI–BamHI digested vector pE-SUMO to construct the recombinant plasmid pE-SUMO-hepA () [Citation6]. The resultant plasmid pE-SUMO-hepA was transformed into the E. coli strain DH5α by the CaCl2–heat shock method. The presence and the correct orientation of the insert sequence were confirmed by PCR and DNA sequencing (Genewiz Beijing company).
Expression and SDS-PAGE analysis of HepI fusion protein
The plasmid with a correct insert sequence was transformed into E. coli BL21 (DE3) competent cells by the CaCl2–heat shock method. Cells were streaked onto Luria–Bertani (LB) plates containing 50 μg/mL of kanamycin and 34 μg/mL of chloramphenicol incubated at 37 °C until a single colony appeared. A single colony from culture plates was picked up separately with a sterile toothpick and resuspended into 5 mL of LB medium containing 50 μg/mL of kanamycin and 34 μg/mL of chloramphenicol, followed by incubation at 37 °C until the optical density at 600 nm (OD600) reached 0.6. The cells were then induced with 0.6 mmol/L isopropyl-beta-D-thiogalactopyranoside (IPTG) at 30 °C for 9 h. Cells were washed by Tris–HCl buffer (20 mmol/L, 200 mmol/L NaCl, 5 mmol/L CaCl2, pH 7.4) twice and resuspended in 5 mL of the same buffer, followed by sonication (sonication 5 s, pause 3 s, 300 W) on ice for 10 min to disrupt the cells. After centrifugation at 4 °C and 12,000 g for 20 min to remove cell debris, the supernatant was collected as the soluble protein sample for the subsequent experiment. Twenty-five microlitres the supernatant were taken for the sodium dodecyl sulphate–polyacrylamide gel electrophoresis (SDS-PAGE) analysis. The SDS-PAGE analysis (80 V for 30 min, 120 V for 150 min) was performed in a 12% running gel. The specific proteins stained with Coomassie Brilliant Blue R250 were visualized by Odyssey Infrared Imaging System (Gene Company, Beijing, China).
Purification of HepI fusion proteins
Fifty millilitres of cell cultures were harvested and resuspended in Tris–HCl buffer (50 mmol/L, 300 mmol/L NaCl, 5 mmol/L CaCl2, pH 7.4) and were lysed by sonication. After the cells were centrifuged for 20 min at 12,000 r/min at 4 °C, the supernatant was filtered using a 0.45 μm filter membrane and then loaded onto a Hi-Trap metal-chelating affinity column. The column was eluted with a buffer (50 mmol/L Tris, 300 mmol/L NaCl, 5 mmol/L CaCl2, 50 mmol/L imidazole, pH 7.4) to obtain the purified SUMO–HepI. The purity of heparinase was detected by SDS-PAGE analysis.
MALDI mass spectrometry
In this study, a novel proteomic approach based on matrix-assisted laser desorption/ionization time-of-flight mass spectrometry (MALDI-TOF-MS) analysis was evaluated for the identification and differentiation of protein samples. In the peptide mass fingerprinting (PMF) data generated by MALDI-TOF-MS, the mass values were MH+. The protein score was −10*Log(P), where P is the probability that the observed match is a random event. Protein scores derived from ion scores as a non-probabilistic basis for ranking protein hits, greater than 13, are significant (p < 0.05).
Protein quantification and enzymatic activity assay
Protein concentrations were determined using the Bradford protein assay kit (Solarbio). The total protein was analyzed by sodium dodecyl sulfate-polyacrylamide gel electrophoresis (SDS-PAGE). The proportion of fusion protein SUMO–HepI in the total protein fraction was analyzed using specialized software Quantity One (4.3.1). Heparinase activity was measured at 232 nm according to the ultraviolet (UV) method (Mapada, Shanghai, China) [Citation7]. The analysis of the heparinase activity was performed according to the method described by Sigma–Aldrich. The enzymatic reaction was carried out at 30 °C using heparin as the substrate in Tris–HCl buffer (pH 7.5) (containing 2% heparin, 50 mmol/L NaCl, 4 mmol/L CaCl2 and 20 mmol/L Tris-HCl, 0.01% bovine serum albumin (BSA). The relative activity was calculated as a ratio of the heparinase activity under the reaction conditions to that of the control sample. One unit of enzyme activity was defined as the amount of enzyme that forms 0.1 μmole of unsaturated uronic acid per hour at pH 7.5 at 30 °C. One International Unit (IU) is equivalent to approximately 600 Sigma units.
ct = 6 is a time correction factor (from 10 min to 1 h as per the unit definition);
V = 3 mL is the volume (in millilitres) of the assay;
cf = 10 is a conversion factor (from 1 µmole to 0.1 µmole) as per the unit definition;
ϵ = 5.50 L/(mmol cm) is the millimolar extinction coefficient of unsaturated uronic acid products at 235 nm;
Vsample = 0.075 mL is the volume (in millilitres) of crude enzyme used.
Data analysis
The results are presented as mean values from three experiments, with standard deviation (±SD).
Results and discussion
Construction of fusion expression vectors of F. heparinum HepI
HepI can specifically degrade heparin into LMWH. Thus, active and renewable HepI is essential for determining the subtle structure of heparin and producing LMWH for clinical applications. Commercial HepI is mainly produced from fermenting F. heparinum. However, it is expensive due to its low yield and activity. E. coli is one of the best host systems for producing recombinant HepI in a simple and economical way, but it has been shown that the expression of HepI from F. heparinum in E. coli is difficult for refolding issues and proneness to aggregation into insoluble inclusion bodies [Citation3,Citation8,Citation9]. According to previous studies, the fusion system is one of the most effective approaches to improve the soluble expression of recombinant proteins in E. coli [Citation10,Citation11]. Soluble partners are able to enhance the solubility of recombinant protein, such as glutathione S-transferase (GST), maltose-binding protein (MBP), thioredoxin (Trx), etc [Citation12,Citation13]. Among the expression partners, SUMO, a small ubiquitin-modifying protein which can dramatically improve the soluble expression, has attracted a lot of attention recently [Citation14,Citation15]. A comparison between SUMO fusion technologies and traditional gene fusion systems implies that SUMO is superior to traditional fusion tags in enhancing protein expression and solubility [Citation14]. In the present study, in order to avoid the potential interference of the His6-SUMO tag on the activity of HepI, the coding sequence of a flexible peptide linker (G4S) and a bovine enterokinase (D4K) site were added at the N-terminal of HepI using overlapping PCR, and then and the spliced fragment was inserted into the pE-SUMO vector. The map of the obtained pE-SUMO-hepA recombinant vector is shown in (a). As shown in (b), the results from the PCR verification suggested that the target fragment had been successfully inserted into the vector and the following DNA sequencing also validated that the G4S-D4K-HepI coding sequence was combined into one open reading frame with the His6-SUMO tag of the pE-SUMO vector.
Soluble expression analysis of the target protein
To confirm whether the fusion expression strategy employed in our study was able to enhance the solubility of recombinant HepI in E. coli, the recombinant strain was induced by IPTG at 30 °C. The supernatant and precipitate of the ultrasonic lysate of bacterial cells were separated by SDS-PAGE. As shown in , after the IPTG introduction, the HepI fusion protein expressed solubility at a high yield reaching about 87% of the total fusion protein, indicating that the fusion expression with a His6-SUMO tag might be an effective way to produce soluble HepI.
Optimization of the fermentation conditions for the soluble expression of the recombinant SUMO–HepI
In order to obtain optimal expression conditions for the soluble expression of recombinant HepI, the effect of four factors, including the shaking speed, the concentration of IPTG, temperature and induction times on the yield of soluble recombinant products (SUMO–HepI) were considered. As shown in and Figure S1 (Online supplemental data), the optimum procedure for soluble expression of SUMO–HepI was as follows: the recombinant strain was firstly cultured in LB medium at 37 °C. When the OD600 of the cultures reached 0.6, 0.6 mmol/L IPTG was added to the broth for induction of the recombinant protein at 30 °C, 150 r/min for 9 h.
Enzymatic properties of recombinant SUMO–HepI
Sometimes, a fusion tag might affect the structure and bioactivity of target proteins. Therefore, a G4S flexible peptide linker and a bovine enterokinase site were inserted between SUMO and HepI in the present study. The flexible linker could act as a hinge which makes HepI separated off from the His6-SUMO tag. The bovine enterokinase site was designed for cutting off the SUMO tag when the tag interfered with the activity of heparinase. As the results showed, the recombinant products could exhibit heparinase activities without requirements of in vitro refolding and SUMO-tag releasing processes. The optimal conditions for enzyme activity were 37 °C and pH7 ((a,b)). In addition, as Ca2+ can bind strongly with the sulphhydryl group of cysteine in enzyme molecules, this can modify the structure and activity of enzymes [Citation16–18]. The effect of different concentrations of CaCl2 on the activity of recombinant products was also tested, and the results showed that the highest heparinase activity was obtained when the concentration of CaCl2 was 10 mmol/L ((c)), suggesting that the interaction between calcium and HepI was important for proper functioning of the enzyme and CaCl2 could be used as an enhancer of our recombinant protein [Citation18].
Purification of the recombinant SUMO–HepI
As the recombinant fusion protein contained a 6 × His tags, the heparinase was purified by Ni2+-chelating affinity chromatography. The SDS-PAGE results showed that the fusion protein SUMO–HepI could be eluted effectively from the column with elution buffer containing 50 mmol/L imidazole ((a)). The purified protein was also confirmed correctly by MALDI mass spectrometry. Compared to the crude enzyme, the specific activity of the purified enzyme increased markedly. Besides, addition of 10 mmol/L Ca2+ could enhance the activity of both the crude enzyme and the purified enzyme ((b)). Thus, our results, taken together, demonstrated that fusion with a SUMO-tag could greatly improve the soluble expression of recombinant HepI and CaCl2 could be used as an enhancer of the recombinant HepI.
Conclusions
The results from this study demonstrated that fusion with a hexahistidine-tagged SUMO could significantly improve the soluble expression of recombinant HepI. The recombinant protein SUMO–HepI reached the highest soluble expression when the expression strain was induced by 0.6 mmol/L IPTG at 30 °C with a shaking speed of 150 r/min for 9 h. The analysis of enzymatic characteristics indicated that the fusion protein could exhibit high enzyme activity without requirements of in vitro refolding and a SUMO-tag release process, and the suitable conditions for the enzyme activity were 30 °C, pH 7.0, and with 10 mmol/L Ca2+ in the reaction buffer. These data suggested that our fusion expression strategy could facilitate the production and applications of heparinase I, especially for the industrial preparation of LMWHs.
Supplementary_Data.pdf
Download PDF (119.7 KB)Disclosure statement
No potential conflict of interest was reported by the authors.
Additional information
Funding
References
- Laurent P, Dussarat GV, Bonal J, et al. Low molecular weight heparins: a guide to their optimum use in pregnancy. Drug. 2002;62(3):463–477.
- Godavarti R, Sasisekharan R. Heparinase I from Flavobacterium heparinum. Role of positive charge in enzymatic activity. J Biol Chem. 1998;273:248–255.
- Ernst S, Venkataraman G, Winkler S, et al. Expression in Escherichia coli, purification and characterization of heparinase I from Flavobacterium heparinum. J Biol Chem. 1996;315(2):589–597.
- Chen Y, Xing X-H, Ye F, et al. Production of MBP–HepA fusion protein in recombinant Escherichia coli by optimization of culture medium. Biochem Eng J. 2007;34:114–121.
- Kane JF. Effects of rare codon clusters on high-level expression of heterologous proteins in Escherichia coli. Curr Opin Biotechnol. 1995;6:494–500.
- Terpe K. Overview of tag protein fusions: from molecular and biochemical fundamentals to commercial systems. Appl Microbiol Biotechnol. 2003;60:523–533.
- Bernstein H, Yang VC, Cooney CL, et al. Immobilized heparin lyase system for blood deheparinization. Methods Enzymol. 1988;137:515–529.
- Sasisekharan R, Bulmer M, Moremen KW, et al. Cloning and expression of heparinase I gene from Flavobacterium heparinum. Proc Natl Acad Sci USA. 1993;90(8):3660–3664.
- Shpigel E, Goldlust A, Efroni G, et al. Immobilization of recombinant heparinase I fused to cellulose-binding domain. Biotechnol Bioeng. 1999;65(1):17–23.
- Davis GD, Elisee C, Newham DM, et al. New fusion protein systems designed to give soluble expression in Escherichia coli. Biotechnol Bioeng. 1999;65(4):382–388.
- Wang C, Castro AF, Wilkes DM, et al. Expression and purification of the first nucleotide-binding domain and linker region of human multidrug resistance gene product: comparison of fusions to glutathione S-transferase, thioredoxin and maltose-binding protein. Biochem J. 1999;338(Pt 1):77–81.
- Esposito D, Chatterjee DK. Enhancement of soluble protein expression through the use of fusion tags. Curr Opin Biotechnol. 2006;17(4):353–358.
- Jia B, Jeon CO. High-throughput recombinant protein expression in Escherichia coli: current status and future perspectives. Open Biol. 2016;6(8):160196. DOI: 10.1098/rsob.160196
- Marblestone JG, Edavettal SC, Lim Y, et al. Comparison of SUMO fusion technology with traditional gene fusion systems: enhanced expression and solubility with SUMO. Protein Sci. 2006;15(1):182–189.
- Butt TR, Edavettal SC, Hall JP, et al. SUMO fusion technology for difficult-to-express proteins. Protein Expr Purif. 2005;43(1):1–9.
- Liu D, Shrive Z, Godavarti R, et al. The calcium-binding sites of heparinase I from Flavobacterium heparinum are essential for enzymatic activity. J Biol Chem. 1999;274(7):4089–4095.
- Ma X, Wang Z, Li S, et al. Effect of CaCl2 as activity stabilizer on purification of heparinase I from Flavobacterium heparinum. J Chromatogr B Analyt Technol Biomed Life Sci. 2006;843(2):209–215.
- Shriver Z, Liu D, Hu Y, et al. Biochemical investigations and mapping of the calcium-binding sites of heparinase I from Flavobacterium heparinum. J Biol Chem. 1999;274(7):4082–4088.