ABSTRACT
Euphorbia helioscopia L. is an herbaceous species of Euphorbia (Euphorbiaceae). As an ancient folk herbal medicine, the most effective medicinal component is terpenoid. Diphosphomevalonate decarboxylase (MDC) is the last rate-limiting enzyme of generating the isopentenyl pyrophosphate precursor of terpenoid in MVA pathway. The gene of MDC was cloned successfully from E. helioscopia, and named EhMDC (accession number: KP995936). The full length cDNA of EhMDC was 1653 bp, and it contained an open reading frame of 1245 bp, a 5′ untranslated region of 184 bp and a 3′ untranslated region of 224 bp. EhMDC contained 415 amino acids. Homologous sequence analysis showed that amino acid sequence of EhMDC had the highest identity of 88% with Ricinus communis MDC. Phylogenetic analysis of EhMDC indicated E. helioscopia, Hevea brasiliensis, R. communis, and Jatropha carcas which all belonged to Euphorbiaceae were classified into one class. Real-time PCR assay demonstrated EhMDC was constitutively expressed in roots, stems and leaves with a similar transcription level. Furthermore, in combination with immunoblot analysis and transmission electron microscopy immunogold labeling after anti-EhMDC antibody preparation, the EhMDC was found to be located in the cisternae of the endoplasmic reticulum, small vacuoles from endoplasmic reticulum and cytoplasm of laticifers. As a result, terpenoid biosynthesis site and accumulation of E. helioscopia laticifers were speculated.
Introduction
Terpenoid biosynthesis pathway is one of the important metabolic pathways in plant. Plant terpenoid biosynthesis mainly have two ways, namely mevalonate (MVA) pathway and C-methyl-2-D-erythritol-4-phosphate (MEP) pathway [Citation1]. The similarity of these two pathways is taking the iso-amylene pyrophosphate (IPP) which is a precursor of terpenoids material synthesis as the main intermediate product. Their difference lies in the synthesis mechanism, the metabolic end product, and different location in plant cells of IPP and its isomer dimethylallyl pyrophosphate (DMAPP). MVA pathway occurs mainly in the cytoplasm, so it is also called the cytoplasm way (Eytosolic pathway). It was first first found in animals and yeast by Conrad Bloch and Feodor Lynen in 1958 [Citation2]. A hydroxy valeric acid pyrophosphate decarboxylase (MDC) catalyses the decarboxylation of the six-carbon mevalonate diphosphate (MVA-PP) into the five-carbon isopentenyl diphosphate (IPP), which is the final step of generating IPP in the MVA pathway, and the participation of ATP and magnesium 2+ is required [Citation3,Citation4]. Therefore, MDC is the last rate-limiting enzyme of generating the isopentenyl pyrophosphate precursor of terpenoid in MVA pathway. At present, the coding genes of MDC have been cloned from man (Homo sapiens) [Citation5], mice (Rattus norvegicus) [Citation6], yeast (Saccharomyces cerevisiae) [Citation7], Picrophilus torridus [Citation8], Leptinotarsa decemlineata [Citation9], Ginkgo biloba [Citation4,Citation10], Solanum lycopersicum [Citation11], and Bacopa monniera [Citation12] and so on.
Terpenoid not only plays significant role in maintaining the life activities of plants and regulating the relationship between environment and plants, but also has significant commercial value, because it is widely used in industrial manufacture, medicine and health. E. helioscopia is an herbaceous species of Euphorbia (Euphorbiaceae) and is distributed in most areas of China. As an ancient folk herbal medicine, E. helioscopia has the efficacy of reducing phlegm, eliminating edema, cruing skin eruption, reducing swelling and itching and others [Citation10,Citation13–Citation15]. Any aerial part of the E. helioscopia plant can be used in pharmacological evaluations [Citation16]. The most effective medicinal component is terpenoid [Citation10,Citation15,Citation17,Citation18]. Wang (2016) isolated two diterpenes from E. helioscopia, 15-O-Acetyl-3-O-benzoylcharaciol and helioscopinolide A, and found that they may provide potential therapeutic strategy for various neuroinflammatory diseases [Citation19]. Researches for some genes involved in the terpenoid biosynthesis from plants have been carried out [Citation20–Citation24]. However, there have been controversies about the terpenoid biosynthesis site, terpenoid transfer and accumulation pathway. Therefore, in this paper, EhMDC from E. helioscopia involved in the terpenoid biosynthesis was cloned using RACE methods, and immunoblotting and subcellular localization of EhMDC were carried out. All of these studies would lay the foundation for deeper understanding of terpenoid biosynthesis site, terpenoid transfer and accumulation pathway of E. helioscopia in laticifers, and also provide theoretical basis for the regulating and controlling of terpenoid biosynthesis and laticifer development.
Materials and methods
Plant material
The healthy seedlings collected from the field at the Botanical Garden of Northwest University in Shaanxi Province (Shaanxi, People's Republic of China) were used for RNA extraction, Real-time PCR analysis, immunoblot analysis and electron microscopy analysis.
RNA extraction
Total RNA was extracted from the stems, leaves and roots using General plant total RNA Extraction Kit (BioTeke, Beijing, China) according to the manufacturer's instructions. The quality and concentration of RNA were determined by agarose gel electrophoresis and spectrophotometric analysis (Eppendorf, Germany). The cDNA was synthesized with a PrimeScript™ 1st Strand cDNA Synthesis Kit (Takara, Dalian, China).
Conserved fragment cloning of EhMDC
The primers of MDC-S: 5′ - GTATGTGGCTTAATGGAAAG - 3′ and MDC-A: 5′ - TTATCTTGTGGGATGTATCATTC - 3′ were designed according to the conserved nucleotide regions of several diphosphomevalonate decarboxylase genes from R. communis and other species in GenBank. The PCR amplification included an initial denaturation at 94 °C for 3 min, followed by 30 cycles of denaturation at 94 °C for 30 s, annealing at 55 °C for 30 s, and elongation at 72 °C for 1 min, with a final elongation step at 72 °C for 10 min. The products were purified (Tiangen, Beijing, China) and cloned into a pMD 19-T Vector (Takara, Dalian, China) before sequencing.
3′-RACE of EhMDC
According to the manufacturer's suggestion, two specific primers MDC-3-S1: 5′ - AGGCAAGGTTCAGGCAGTG - 3′ and MDC-3-S2: 5′ - GCGTGTCGCAGTTTATT TGG - 3′ were designed based on the cloned EhMDC fragment. The 3′-RACE was carried out with a 3′ - Full RACE Core Set (Takara, Dalian, China). The primary 3′-RACE PCR (Outer PCR) was performed with reaction condition including an initial denaturation at 94 °C for 3 min, then 25 cycles of amplification (94 °C for 30 s, 55 °C for 30 s and 72 °C for 2 min), and followed by a final elongation at 72 °C for 10 min. Using the Outer PCR products as templates, the second 3′-RACE PCR (Inner PCR) was performed by an initial denaturation at 94 °C for 3 min, then 35 cycles of amplification (30 s at 94 °C, 30 s at 56 °C and 2 min at 72 °C). The product was purified and cloned into a pMD 19-T Vector (Takara, Dalian, China) followed by sequencing.
5′-RACE of EhMDC
The 5′ RACE-ready cDNA was acquired using the SMARTer™ RACE cDNA Amplification Kit (Clontech, USA). Based on the cloned conserved fragment, the 5′-RACE primer MDC-5-A1: 5′ - CATCCCAATGCTTCTCATCCGCAACT - 3′ and MDC-5-A2: 5′ - CTGCGACACGCACTGCCTGAACC - 3′ were designed and synthesized. Using the 5′RACE-Ready cDNA as templates, the primary 5′-RACE PCR (Outer PCR) was performed with MDC-5-A1 and Universal Primer A Mix (UPM, provided in the kit) by 25 cycles of amplification (30 s denaturation at 94 °C, 30 s annealing at 68 °C and 2 min elongation at 72 °C). The product was used as templates for Inner PCR with MDC-5-A2 and Nested Universal Primer A (NUP, supplied in the kit). The inner PCR was performed by 20 cycles of amplification including 30 s denaturation at 94 °C, 30 s annealing at 68 °C and 1 min elongation at 72 °C). The product was purified and cloned into a pMD 19-T Vector (Takara, Dalian, China) and sequenced.
The full-length cDNA cloning of EhMDC
The conserved sequence, 3′-RACE and 5′-RACE products were assembled. As a result, the full-length cDNA sequence of the EhMDC gene was deduced. Based on the products cloned of 5′ RACE and 3' RACE, primers MDC-5′-S: 5′ - CACTAACCCACCAGTCCAACG - 3′ and MDC-3′-A: 5′ - CATCATTGGCAAA TAAGTCGG - 3′ were designed and synthesized. The coding sequence of EhMDC was amplified by RT-PCR. 2 μL 5′ RACE-Ready cDNA was used for the PCR in a total volume of 50 μL under the following conditions: 30 cycles of denaturation at 98 °C for 10 s, annealing at 55 °C for 5 s, and elongation at 72 °C for 2 min. The PCR product was purified and cloned into a pMD 19-T Vector (Takara, Dalian, China) before sequencing.
Bioinformatics analysis
The EhMDC cDNA sequence was compared online to the non-redundant peptide database at the National Center for Biotechnology Information (NCBI) (http://www.ncbi.nlm.nih.gov). Multiple alignment analysis was performed with DNAMAN software. Subsequently, a phylogenetic tree was constructed using MEGA 6.0 software by applying the neighbour-joining method.
Quantitative real-time PCR analysis
Real-time PCR analyses were carried out by two-step PCR procedure with SYBR® Premix Ex Taq™ (Tli RNaseH Plus) (Takara, Japan) and the CFX96™ Real-Time PCR System (Bio-Rad, United States). The real-time PCR reaction mixture of 25 µL including: 1 µL cDNA solutions, 12.5 µL SBRY Premix Ex Taq, 9.5 µL DEPC-treated water, 1 µL forward primer and 1 µL reverse primer. PCR conditions were as follows: 95 °C for 30 s, 39 cycles of 95 °C for 10 s and 60 °C for 30 s. The immediate generation of melting curves enables to distinguish specific product from all products and exclude primer dimers, which is performed at 60 °C for a starting temperature and 95 °C for an end temperature, with a heating rate of 0.1 °C/s. The constitutively expressed actin gene served as the internal standard. The following primer pairs were used for target gene amplification: Actin, 5′-GGTAACATTGTGCTCAGTGGTGG-3′ and 5′-AACGACCTTAATCTTCATGCTGC-3′; EhMDC, 5′-AGCGACAGCCTTGCTATTCA-3′ and 5′-TTCGTGAACTTACCACAGCG-3′. Gene expression was quantified by the 2–ΔΔCT method. Experiments were performed in three biological replicates, and the results were represented as mean values ± standard deviation (SD).
Prokaryotic expression and antibody preparation
The plasmids used for overexpressing 6 × His-MDC recombinant protein in Rosetta cells were derived from pET-43a (+) vector (Novagen). The 1-507 nucleotide fragment was subcloned into pET-43a (+) and confirmed by sequencing. The cells were grown at 37 °C for more than 16 h, and then induced by the addition of isopropyl-D-thiogalactopyranoside. Cells were collected by centrifugation. Recombinant 6 × His-MDC proteins were purified as His fusion proteins using a nickel-nitrilotriacetic acid agarose column according to the manufacturer's instructions. Protein concentrations were determined with SDS-PAGE, and then the gel pieces containing the recombinant proteins were extracted and injected directly into healthy rabbits. Antibodies from the rabbits were affinity purified using Cyanogen bromide-activated sepharose (Sigma-Aldrich, USA) conjugated with recombinant proteins and the specificity of antibodies was proved by the ELISA and immunoblot (WB).
Immunoblot analysis
Western-blot analysis was performed as described by Chung with some modifications [Citation25]. Protein samples prepared from E. helioscopia latex were splitted in lysis buffer (7 mol/L Urea, 2 mol/L Thiourea, 4% CHAPS, 2 mmol/L EDTA, 2 mmol/L Tris, 1 mmol/L PMSF). Total proteins were separated by 12% SDS-PAGE and electrophoretically transferred onto nitrocellulose membrane. The nitrocellulose membrane was incubated with EhMDC antibodies (diluted 1/1,000 with TBST) overnight at 4 °C. Following incubation with the secondary antibody (diluted 1/2000 with TBST), horseradish peroxidase conjugated immunodetection was detected using the enhanced chemiluminescence system (Tanon, Shanghai, China).
Electron microscopy analysis
Tender buds were fixed in a solution of 0.25% glutaraldehyde and 4% paraformaldehyde in 0.1 mol/L phosphate buffer (pH 7.2) for 4 h at 4 °C, rinsed in 0.1 mol/L phosphate buffer (pH 7.2). After rinsing, samples were dehydrated stepwise in an acetone series from 30% to 100% and embedded in LR White resin (Sigma-Aldrich, USA). Thin sections (60–70 nm) collected on nickel grids (1-GN; 150 meshes) were washed with PBS for three times, and blocked in 3% BSA-TBST solution for 1 h at 37 °C. Subsequently, samples were incubated in anti-EhMDC antibodies at a dilution of 1:50 over night at 4 °C, 37 °C for 1 h, and washed with PBS three times. The primary antibody binding was detected using anti-rabbit IgG (10-nm gold; Bioss, Beijing, China). After washing with PBS and distilled water, the nickel nets were examined and photographed with JEM-1230 (TEM H-7650, Japan).
Results and discussion
The most effective medicinal component in Euphorbia helioscopia which is a traditional herb in China is terpenoid [Citation10,Citation15]. MDC is the last rate-limiting enzyme of generating the isopentenyl pyrophosphate precursor of terpenoid in MVA pathway [Citation3]. The diphosphomevalonate decarboxylase (MDC) could also be written as mevalonate pyrophosphate decarboxylase which is abbreviated as MVD. Based on an analysis of a positive correlation between the expression of the Gl-mvd gene and triterpene content changes in Ganoderma lucidum during development, and the overexpression of Gl-mvd increased the expression of several genes involved in the triterpene biosynthetic pathway and the triterpene accumulation compared with the wild-type strain suggested that mvd may play an important role in triterpene biosynthesis regulation [Citation26]. As a result, the molecular cloning and expression analysis of MDC in E. helioscopia was carried out.
The full length cDNA of EhMDC gene
The full length cDNA of MDC gene, named EhMDC was generated by joining the 5' end sequence, 3' end sequence and gene segments with the DNAMAN analysis software. The splicing cDNA sequence was verified by cloning of the open reading frame with the PrimeSTAR® HS DNA Polymerase. The length of EhMDC cDNA is 1653 bp, containing a length of 1245 bp open reading frame, coding a protein containing 415 amino acids with a calculated molecular weight of 45.971 kDa. The gene sequence has been submitted to the Genebank, and the accession number is KP995936. The nucleotide sequence and amino acid sequence are shown in .
The function domain analysis of the EhMDC protein
Using InterProScan online tools, the function structure of the EhMDC amino acid sequence domain are analyzed. EhMDC protein (EC: 4.1.1.33) belongs to the GHMP kinase superfamily. The role of this enzyme is transferring the phosphate group of ATP to the amino acid residues of a particular substrate, catalysing the protein phosphorylation and participating in a variety of signal transduction process. The family of the enzyme has the typical Pro-X-X-X-Gly-Leu-X-Ser-Ser-Ala sequential patterns [Citation27].
The segment of 169-112 amino acids is the GHMP kinase N-terminal conservative functional domains, the segment of 413-199 amino acids is the GHMP kinase C side structure domain.
EhMDC homology alignment of amino acid sequence and phylogenetic tree
The homology comparison of EhMDC amino acid sequence showed that the homology of EhMDC amino acid sequence with other species is very high using NCBI BLAST tools. The consistency of EhMDC with Ricinus communis is 88%, and the consistency with Jatropha curcas, Populus euphratica, Hevea brasiliensis and Populus trichocarpa were 87%. The multiple comparison of EhMDC amino acid sequence with the other 11 species using DNAMAN is shown in .
Figure 2. Multiple alignment of MDC amino acid sequences of Euphorbia helioscopia and other species. Black shading means 100% sequence identity, grey shading means more than 75% sequence identity, light grey means more than 50% sequence identity.
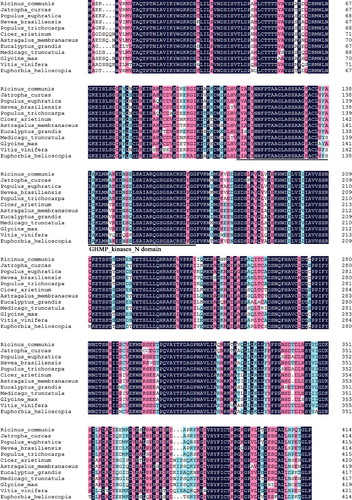
Amino acid sequences of 17 homologous proteins were selected for the phylogenetic tree analysis in order to understand EhMDC evolutionary relationships with other plants. The neighbour-Joining method of MEGA6 software was used to construct the phylogenetic tree (), the bootstrap is set to 1000, evolutionary distance using poisson correction method. The phylogenetic tree showed that the MDC of E. helioscopia, Hevea brasiliensis, R. communis, Populus euphratica and Jatropha carcas were classified into one class and exhibited close association. E. helioscopia, Hevea brasiliensis, R. communis, and Jatropha carcas belong to the same family, Euphorbiaceae. The MDC of E. helioscopia had parallel relationship with Brassica napus, Camelina sativa, and Arabidopsis thaliana. However, MDC displayed a more distantly related association with other plants including Prunus mume, Malus domestica, Fragaria vesca, Cicer arietinum, Astragalus membranaceus, Phaseolus vulgaris, Glycine max, Nicotiana sylvestris, Solanum tuberosum, Catharanthus roseus, Sesamum indicum, Platycodon grandiflorus and Panax ginseng. In addition, most of the species in each small branch belong to the same family. As a result, the phylogenetic relationships of EhMDC with the MDC from other species had a good consistence with the traditional botanical classification and showed that MDC in different species had been derived from a common ancestor.
Figure 3. Phylogenetic analysis of MDC amino acid sequences of Euphorbia helioscopia and other species. Ricinus communis: (XP_002521 172.1); Populus euphratica: (XP_011024 216.1); Jatropha curcas: (KDP35789.1); Hevea brasiliensis: (BAF98285.1); Brassica napus: (CDX73494.1); Camelina sativa: (XP 010515 945.1); Arabidopsis thaliana: (AEE79204.1); Prunus mume: (XP_008232 018.1); Malus domestica: (XP_008345 749.1); Fragaria vesca: (XP_004307 061.1); Cicer arietinum: (XP_004497 159.1); Astragalus membranaceus: (AID51442.1); Phaseolus vulgaris: (XP_007142839.1); Glycine max: (XP_003555 870.1); Nicotiana sylvestris: (XP 009792 788.1); Solanum tuberosum: (XP_006361 468.1); Catharanthus roseus: (ADR65113.1); Sesamum indicum: (XP_011084 229.1); Platycodon grandiflorus: (AGZ15316.1); Panax ginseng: (ADI80345.1).
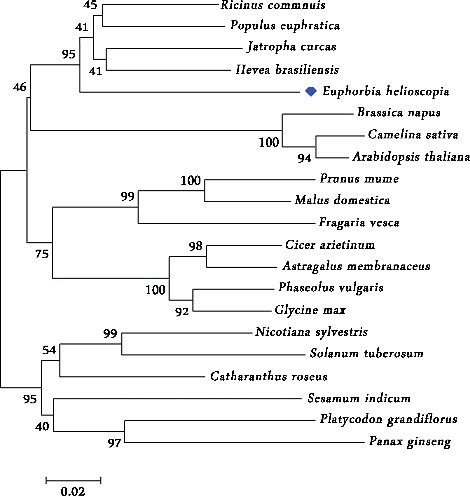
The organ-specific expression pattern of the EhMDC gene
EhMDC expression in roots, stems and leaves were performed using Real-time quantitative PCR. The results showed that EhMDC ubiquitously expressed in all the organs examined. EhMDC in roots was more abundant than that in stems and leaves (). Pang et al. (2006) tested the GbMVD expression in Ginkgo biloba using RT-PCR. They found that GbMVD was transcribed in root, stem and leaf, and there was no distinct difference in their transcription levels [Citation28]. To assess the organ-specific and environmentally responsive characteristics of GbMVD expression, Liao et al. (2016) fused GbMVDpro to GUS reporter gene. GbMVDpro:GUS was introduced into tobacco. Histological analysis of the transgenic tobacco showed that GUS mainly localized in the leaf epidermis, stem secondary xylem, and root vasculature [Citation4], which is closely correlated with the results previously reported by Pang et al. [Citation28]. The Expression of AtMVD1 was shown to be low in Arabidopsis thaliana because a weak signal was observed [Citation7]. We found the EhMDC gene was not differentially regulated in an organ-specific manner. The expression pattern in the root, stem and leaf was similar, because the technical standard deviation of RT-PCR analysis was not significantly different (P < 0.05). This pattern of expression was also found in A. thaliana MVK [Citation29], HMG1 [Citation30], and FPS1 genes [Citation31], which encode specific isoforms of the isoprenoid biosynthetic enzymes mevalonate kinase (MVK), 3-hydroxy-3-methylglutaryl coenzyme A reductase (HMGR) and farnesyl-diphosphate (FPP) synthase.
Figure 4. The expression of EhMDC in root, stem and leaf of Euphorbia helioscopia. Error bars on each column indicate the standard error (SE) from three biological replicates. All the reactions were normalized using the Ct value corresponding to the ACTIN gene. The level of root was particularly expressed as a ratio of the gene expression, which was set at 1. Bars represented the technical standard deviation was not significantly different (P < 0.05).
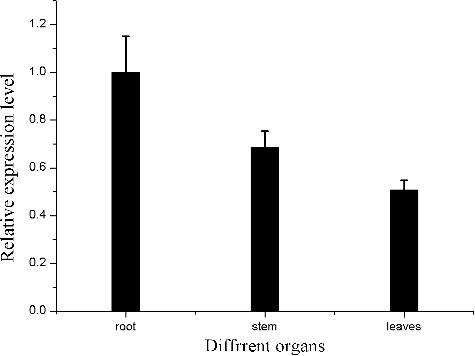
Immunoblotting and subcellular localization of EhMDC
E. helioscopia is a famous Chinese herbal medicine. The whole plant contains laticifers, a type of secretory tissue, secreting white milks called latex [Citation32]. The laticifers are an important site of terpenoids biosynthesis and accumulation [Citation33]. Tapped latex is capable of biosynthesis of triterpenoids from acetate indicating that laticifer cells are active in the production of these compounds as well as in their storage [Citation34]. Terpenoids are biosynthesized via two pathways: the mevalonate (MVA) pathway and the methylerythritol phosphate (MEP) pathway. The typical MVA pathway occurs in the cytoplasm and ER [Citation35], whereas the MEP pathway occurs in the plastids [Citation36]. Both pathways have isopentenyl diphosphate (IPP) as a major intermediate product. MDC is the last rate-limiting enzyme of generating the isopentenyl pyrophosphate (IPP) precursor of terpenoids in MVA pathway [Citation4,Citation12]. We used PredictProtein and TargetP 1.1 tools for the prediction of EhMDC subcellular localization, the results showed that the deduced EhMDC protein does not contain a typical signalling sequence of secretary pathway, and it is probably located in the cytoplasm. Therefore, to investigate EhMDC expression in laticifers of E. helioscopia, the anti-EhMDC antibody was prepared. Then latex proteins were exacted and were used for western blotting. Immunoblotting demonstrated that the antibody was specific for a band estimated to be about 45.971 kDa being consistent with the calculated molecular mass of EhMDC (), which proved that the EhMDC existed in laticifers of E. helioscopia.
Figure 5. Immunoblot analysis of EhMDC antibody from the protein extracts of Euphorbia helioscopia laticifers. lane 1: Marker; lane 2: the corresponding protein gel blot probed with affinity-purified anti-EhMDC antibody.
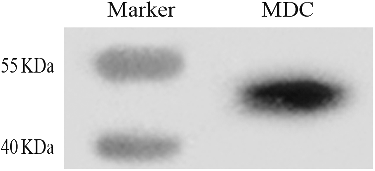
The subcellular localization of EhMDC in laticifers was observed using immunogold electron microscopy. In order to ensure the visibility of the antigen, stem apexes were not post fixed with osmium tetroxide, so the membrane in immunogolden electron microscopic pictures shows electronic transparence. In (b,c), it could be seen that golden particles with MDC antibodies were distributed in cisternae of endoplasmic reticulum and small vacuoles from endoplasmic reticulum ((b,c)), and also distributed randomly in protoplasm of laticifer cells ((b–d)), whereas, preimmune controls showed no immunogold labeling ((a)). From our early observation of E. kansui Liou laticifers at early developmental stages, some endoplasmic reticulum dilated and coalesced to form small vacuoles, which began to accumulate latex particles, and then transport and release these latex particles into the central vacuole with their coalesce [Citation37]. Skrukrud reported that mevalonic acid synthesize triterpenoid in the small vacuole of laticifers in Euphorbia Lathyri [Citation38]. Fineran's ullrastructural observations indicated that latex particles were synthesized in tubular vacuoles in the peripheral cytoplasm of developing E. pulcherrima laticifers [Citation39]. As a result, we speculated that the isopentenyl pyrophosphate (IPP) were synthesized in the endoplasmic reticulum, and then transported to parts which dilated into small vacuoles, and the terpenoid was further synthesized into latex particles in these small vacuoles.
Figure 6. Immunolocalization of the MDC antibody in Euphorbia helioscopia laticifers. (a) Preimmune controls showed no gold labelling in the cells. Bars = 200 nm. (b) MDC signals appeared to locate in small vacuoles from endoplasmic reticulum (arrows). Bars = 200 nm. (c) MDC signals appeared to locate in the cisternae of endoplasmic reticulum (arrows). Bars = 200 nm. (d) Golden particles conjugated MDC antibodies were found to locate in the cytoplasmic matrix randomly (arrow). Bars = 200 nm.
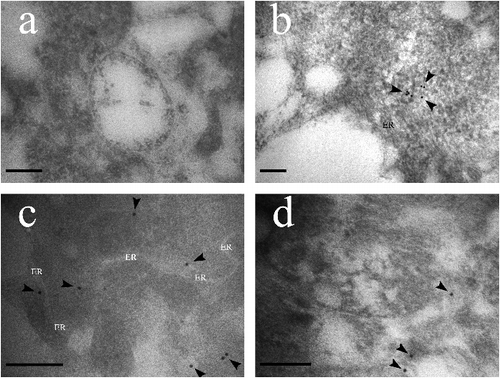
Conclusions
Our results suggested that the full length cDNA of the gene of diphosphomevalonate decarboxylase from Euphorbia helioscopia L. (EhMDC, accession number: KP995936) was 1653 bp, and EhMDC contained 415 amino acids. The EhMDC was found to be located mainly in the cisternae of the endoplasmic reticulum and small vacuoles from endoplasmic reticulum in laticifers. Therefore, the isopentenyl pyrophosphate (IPP) were speculated to be synthesized in the endoplasmic reticulum, and then transported to parts which dilated into small vacuoles, and the terpenoid was further synthesized into latex particles in these small vacuoles in E. helioscopia laticifers.
Disclosure statement
The authors declare no conflict of interest.
Additional information
Funding
References
- Rohmer M, Knani M, Simonin P, et al. Isoprenoid biosynthesis in bacteria: a novel pathway for the early steps leading to isopentenyl diphosphate. Biochem J. 1993;295:517–524.
- Lichtenthaler HK. The 1-deoxydxylulose-5-phosphate pathway of isoprenoid biosynthesis in plant. Annu Rev Plant Physiol Plant Mol Biol. 1999;50:47–65.
- Jabalquinto AM, Alvear M, Cardemil E. Physiological aspects and mechanism of action of mevalonate 5-diphosphate decarboxylase. Comp Biochem Physiol. 1988;90:671–677.
- Liao Y, Xu F, Huang X, et al. Characterization and transcriptional profiling of Ginkgo biloba, mevalonate diphosphate decarboxylase gene (GbMVD) promoter towards light and exogenous hormone treatments. Plant Mol Biol Report. 2015;34:1–16.
- Toth MJ, Huwyler L. Molecular cloning and expression of the cDNAs encoding human and yeast mevalonate pyrophosphate decarboxylase. J Biol Chem. 1996;271:7895–7898.
- Toth MJ, Huwyler L, Park J. Purification of rat liver mevalonate pyrophosphate decarboxylase. Prep Biochem Biotechnol. 1996;26:47–51.
- Cordier H, Karst F, Berges T. Heterologous expression in Saccharomyces cerevisiae of an Arabidopsis thaliana cDNA encoding mevalonate diphosphate decarboxylase. Plant Mol Biol. 1999;39:953–967.
- Rossoni L, Hall SJ, Eastham G, et al. The putative mevalonate diphosphate decarboxylase from Picrophilus torridus is in reality a mevalonate-3-kinase with high potential for bioproduction of isobutene. Appl Environ Microbiol. 2015;81:2625–2634.
- Li Q, Meng QW, Lü FG, et al. Identification of ten mevalonate enzyme-encoding genes and their expression in response to juvenile hormone levels in Leptinotarsa decemlineata (say). Gene. 2016;584:136–147.
- Schmidt RJ, Evans FJ. Skin irritants of the sun spurge (Euphorbia helioscopia L.). Contact Derm. 1980;6:204–210.
- Sun J. Cloning and analysis of related genes involved in the terpenoid biosynthesis pathway of tomato [master's degree dissertation]. Wuhan: Huazhong Agricultural University; 2008.
- Abbassi S, Patel K, Khan B, et al. Functional and conformational transitions of mevalonate diphosphate decarboxylase from Bacopa monniera. Int J Biol Macromolec. 2016;83:160–170.
- Zayed SM, Farghaly M, Soliman SM, et al. Dietary cancer risk from conditional cancerogens (tumor promoters) in produce of livestock fed on species of spurge (Euphorbiaceae). J Cancer Res Clin Oncol. 2001;127:40–47.
- Corea G, Di pietro A, Dumontet C, et al. Jatrophane diterpenes from Euphorbia spp. as modulators of multidrug resistance in cancer therapy. Phytochem Rev. 2009;8:431–447.
- Aslam MS, Choudhary BA, Uzair M, et al. A review on phytochemical constituents and pharmacological activities of Euphorbia helioscopia. Ind Res J Pharm Sci. 2014;1:86–95.
- Saleem U, Hussain K, Ahmad M, et al. Physicochemical and phytochemical analysis of Euphorbia helioscopia (L.). Pak J Pharm Sci. 2014;27:577–585.
- Nazir M, Ahmad W, Kreiser W. Isolation and NMR-assignments of 19αH-lupeol from E. helioscopia Linn (N. O. Euphorbiaceae). Pak J Sci Ind Res. 1998;41:6–10.
- Barla A, Birman H, Kultur S. Secondary metabolites from Euphorbia helioscopia and their vasodepressor activity. Turk J Chem. 2006;30:325–332.
- Wang H, Liu Y, Zhang J, et al. 15-O-Acetyl-3-O-benzoylcharaciol and Helioscopinolide A, two diterpenes isolated from Euphorbia helioscopia suppress microglia activation. Neurosci Lett. 2016;612:149–154.
- Schulte AE, Van der heijden R, Verpoorte R. Purification and characterization of mevalonate kinase from suspension-cultured cells of Catharanthus roseus (L.) G. Don. Arch Biochem Biophys. 2000;378:287–298.
- Nakamura A, Shimada H, Masuda T, et al. Two distinct isopentenyl diphosphate isomerases in cytosol and plastid are differentially induced by environmental stresses in tobacco. FEBS Lett. 2001;506:61–64.
- Kai G, Miao Z, Zhang L, et al. Molecular cloning and expression analyses of a new gene encoding 3-hydroxy-3-methylglutaryl-CoA synthase from Taxus media. Biol Plant. 2006;50:359–366.
- Dai Z, Cui G, Zhou SF, et al. Cloning and characterization of a novel 3-hydroxy-3- methylglutaryl coenzyme A reductase gene from Salvia miltiorrhiza involved in diterpenoid tanshinone accumulation. J Plant Physiol. 2011;168:148–157.
- Ma Y, Yuan L, Wu B, et al. Genome-wide identification and characterization of novel genes involved in terpenoid biosynthesis in Salvia miltiorrhiza. J Exp Bot. 2012;63:2809–2823.
- Chung T, Suttangkakul A, Vierstra RD. The ATG autophagic conjugation system in maize: ATG transcripts and abundance of the ATG8-lipid adduct are regulated by development and nutrient availability. Plant Physiol. 2009;149:220–234.
- Shi L, Qin L, Xu Y, et al. Molecular cloning, characterization, and function analysis of a mevalonate pyrophosphate decarboxylase gene from Ganoderma lucidum. Mol Biol Rep. 2012;39:6149–6159.
- Zhou T, Daugherty M, Grishin NV, et al. Structure and mechanism of homoserine kinase: prototype for the GHMP kinase superfamily. Structure. 2000;8:1247–1257.
- Pang Y, Shen G, Bergès T, et al. Molecular cloning, characterization and heterologous expression in Saccharomyces cerevisiae of a mevalonate diphosphate decarboxylase cDNA from Ginkgo biloba. Physiol Plant. 2006;127:19–27.
- Lluch MA, Masferrer A, Arró M, et al. Molecular cloning and expression analysis of the mevalonate kinase gene from Arabidopsis thaliana. Plant Mol Biol. 2000;42:365–376.
- Enjuto M, Balcells L, Campos N, et al. Arabidopsis thaliana contains two differentially expressed 3-hydroxy-3methylglut aryl coenzyme A reductase genes, which encode microsomal forms of the enzyme. Proc Natl Acad Sci USA. 1994;91:927–931.
- Cunillera N, Arró M, Delourme D, et al. Arabidopsis thaliana contains two differentially expressed farnesyl-diphosphate synthase genes. J Biol Chem. 1996;271:7774–7780.
- Wei BF, Cai X, Hu ZH. Developmental anatomy study of vegetative organs in Euphorbia helioscopia L. Acta Bot Boreali-Occidential Sinica. 2011;31:1582–1589.
- Li W, Cai X, Wang YJ. Studies on anatomy of laticifers and localization of diterpene esters in vegetative organs in Euphorbia kansui. Guihaia. 2009;29:176–181.
- Ponsinet G, Ourisson G. Specific aspects of the biosynthese of triterpenes in the latex of Euphorbia. Phytochem. 1968;7:757–764.
- Chappell J. Biochemistry and molecular biology of the isoprenoid biosynthetic pathway in plants. Plant Biology. 1995;46:521–547.
- Lange BM, Wildung MR, McCaskill D, et al. A family of transketolases that directs isoprenoid biosynthesis via a mevalonate independent pathway. Proc Natl Acad Sci USA. 1998;95:2100–2104.
- Cai X, Li W, Yin LF. Ultrastructure and cytochemical localization of acid phosphatase of laticifers in Euphorbia kansui Liou. Protoplasma. 2009;238:3–10.
- Skrukrud CL. Terpenoid biosynthesis in Euphorbia lathyris and Copaifera ssp. Berkeley: Lawrence Berkeley Laboratory, University of California, Berkeley; 1987.
- Fineran BA. Differentiation of non-articulated laticifers in Poinsettia (Euphorbia pulcherrima Willd.). Ann Bot. 1983;52:279–293.