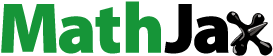
ABSTRACT
Xylose rich of lignocellulosic prehydrolysate could be used for fungal chitosan production effectively by Rhizopus oryzae. When 15 g/L xylose was used as carbon source for R. oryzae AS 3.819 fermentation, almost all of the xylose was consumed for cell growth (4.33 g/L of biomass) and the chitosan extraction yield was 0.11 g/g biomass. Corn stover prehydrolysate by dilute acid-assisted steam explosion pretreatment that contained 30 g/L xylose and 10 g/L glucose was fermented by R. oryzae AS 3.819 for 72 h, the biomass and the chitosan extraction yield were 10.96 g/L and 0.09 g/g biomass. The degree of deacetylation of the fungal chitosan derived from corn stover prehydrolysate (91.27%) was higher than that of the commercial chitosan extracted from natural shellfish exoskeleton (87.25%), and the viscosity of the fungal chitosan derived from corn stover prehydrolysate (2.67 mPa⋅s) had a large decrease compared to the commercial chitosan (22.25 mPa⋅s). The functional groups and thermostability of the fungal chitosan were proofed by Infrared (IR) spectra and Derivative thermogravimetric (DTG), respectively.
Introduction
Chitosan was deacetylated from Chitin, which was one of the most plentiful biopolymers on Earth [Citation1]. The structure of chitosan is a linear of polysaccharide consisted of abundant D-glucosamine and little N-acetyl-D-glucosamine residues connected by β-type glycosidic bonds [Citation2]. Chitosan has unique characteristics such as biodegradability, biocompatibility and inhibitory action [Citation3], so it could be applied in extensive fields, such as pharmaceutics and cosmetology [Citation4–Citation6]. Chitosan is traditionally produced from the waste of shellfish exoskeleton. High concentration of sodium hydroxide (45%–60%) is used in the process of extracting chitosan from chitin [Citation7], which could cause large scale environmental pollution [Citation8]. However, only 4% of sodium hydroxide concentration was used for chitosan extraction from fungal biomass [Citation9], which made fungal chitosan an environment-friendly production.
The filamentous fungi R. oryzae had been shown to be one of the for chitosan production strains [Citation9,Citation10]. R. oryzae TISTR 3189 was shown to have a relatively higher content of chitosan at 0.14 g/g biomass [Citation9]. R. oryzae could ferment glucose and cellobiose into lactic acid, however xylose in fermentation broth could improve the growth of R. oryzae [Citation11].
Lignocellulosic materials are plentiful renewable resources in the biosphere, which could be transformed into different kinds of energy and biochemicals by biorefining processes [Citation12]. Hemicellulose is one of the main components of lignocellulose, which accounts for 25-35% of the biomass [Citation13]. Dilute sulfuric acid pretreatment is an extensive and efficient method for the bioconversion of corn stover [Citation3]. According to previous studies, almost all of hemicellulose in biomass was degraded by mineral acid hydrolysis [Citation14]. However, the dilute acid prehydrolysate containing plenty of xylose and fermentation inhibitors, thus the appropriate xylose fermenting strains lack to grow in prehydrolysate [Citation15]. A lot of papers have been published concerning about xylose fermentation, effects of inhibitors on microbial growth and fermentation and prehydrolysate detoxification [Citation16–Citation18].
The aim of the current work was to produce fungal chitosan from lignocellulosics prehydrolysate by R. oryzae AS 3.819. The effects of glucose, xylose and corn stover prehydrolysate of dilute acid-assisted steam explosion on R. oryzae AS 3.819 fungal chitosan production were investigated, the characteristics of the fungal chitosan were compared with the commercial chitosan.
Materials and methods
Sugars and commercial chitosan
D-glucose, D-xylose and other chemicals were provided by Sigma Aldrich. Commercial chitosan (deacetylation degree 80.0%–95.0%, viscosity 20–500 mPa.s, molecular 100–300 kDa) extracted from natural shellfish exoskeleton was provided by Sigma Aldrich, USA.
Corn stover prehydrolysate
Corn stover was collected from Yancheng, Jiangsu province, China. The cut corn stover (5–10 cm) was pretreated by acid-assisted steam explosion at 0.8 MPa and combined with 12.9 g/L of sulfuric acid for 5 min with a 3-L reactor [Citation19]. The pretreated corn stover was washed with water in the solid-to-liquid ratio of 1:15, after filtration and vacuum concentration (160 bar, 70 °C), the prehydrolysate containing 10.0 g/L glucose and 30 g/L xylose was obtained. The fermentation inhibitors composition of hydrolysate was acetic acid 2.92 g/L, furfural 0.55 g/L, hydroxymethyl furfural 0.35 g/L, formic acid 0.47 g/L, levulinic acid 0.18 g/L. The prehydrolysate was maintained at 4 °C for the later use.
Microorganism and fermentation
R. oryzae AS 3.819 was provided by China General Microbiological Culture Collection Center. The R. oryzae AS 3.819 strain grew on potato-dextrose agar (PDA) slant under the temperature of 30 °C for a week first and then was transferred to fresh PDA slants each month. For the experimentation, the fungal spores from the PDA slant were transferred into sterile water under temperature of 4 °C for spore suspension preparation.
0.2 L spore suspension with 1.0 × 108 cfu/mL of spores was inoculated into 3 L bioreactor (NBS BioFlo 115, USA), that contained 2 L of medium. The R. oryzae AS 3.819 fermentation was carried out at 35 °C and 200 r/min of agitation. After 72 h fermentation, the mycelium was collected, washed, and dried to constant weight at 70 °C. The pure sugar culture medium contained 15.0 g/L of glucose or xylose, and was supplemented with 4.0 g/L of urea, 0.6 g/L of KH2PO4, 0.65 g/L of MgSO4·7H2O, 0.02 g/L of ZnSO4·7H2O, 0.0012 g/L of FeSO4·7H2O. The mixed sugar medium contained 30.0 g/L of xylose and 10.0 g/L of glucose, the other supplemented medium compositions were the same with the pure sugar culture medium. The prehydrolysate culture medium was supplemented with the same compositions as the pure sugar culture medium. All the initial pH of the medium was adjusted to 6.0 using dilute sulfuric acid.
Chitosan extraction
Chitosan extraction from R. oryzae was performed according to the method of Chatterjee et al. [Citation20,Citation21]. The dry biomass was milled, and mixed with 1 mol/L sodium hydroxide solution at a solid-to-liquid ratio of 1:40 (w/v), then processed at 121 °C for 15 min. The solid portion was obtained from 5 min of centrifugation with a speed of 10,000 r/min and then underwent a repeated wash to pH 7.0. The washed solid was mixed with 2% acetate (1:100, w/v) at a temperature of 95 °C for 24 h. After 5 min of centrifugation with a speed of 10,000 r/min, sodium hydroxide was added to adjust the supernatant pH to 10 [Citation22]. Then the precipitate of fungal chitosan sample was obtained by ethanol washing and air drying to constant weight at 70 °C. The purity of fungal chitosan sample was determined according to sulfuric acid hydrolysis method by Zamani et al. [Citation23]. The purity of chitosan preparation was 96.89%. The chitosan extraction yield was calculated using the weight of fungal chitosan sample multiplied by the purity of fungal chitosan sample.
Analysis of sugars and lactic acid
Sugars, L-lactic acid, and fermentation inhibitors were determined by high performance liquid chromatography (Angilent 1100, Germany) with a Bio-Rad Aminex HPX-87H column and a refractive index detector at 50 °C, using 5 mmol/L H2SO4 as the eluent at a flow rate of 0.6 mL/min.
Characterization of chitosan
Degree of deacetylation
0.2 g of Chitosan was added to 60 mL of deionized water with stirring, 0.5 mol/L sodium hydroxide was added to adjust pH to 9.0 and a dispersed slurry was prepared under continuous stirring. Then, 0.1 mol/L HCl was added to adjust the pH to 3.0. At last, 0.05 mol/L of NaOH was added to adjust pH to 11.0 using a pH-Stat titration system at the speed of 0.1 mL/min. The titration curve was measured. From titration curve, the amino group content was calculated by modification method of Czechowska-Biskup et al. [Citation24]. The following equations were used to calculate the degree of deacetylation:
IR spectra
IR spectra of chitosan was determined by a FT-IR instrument (Nicolet, USA). Chitosan powder was completely mixed with KBr and crushed into a superfine powder using a mortar and a pestle. The mixture was pressed into a KBr pellet and then placed into a desiccator for 24 h at a temperature of 100 °C. The IR spectra recorded ranged from 4000 cm−1 to 400 cm−1.
Viscosity
Chitosan viscosity in 1% (w/w) solution with 2% acetate solution was measured by a Multifunctional Rheometer (HAAKE RheoStress 6000, Germany) at 25 °C. Measurements were taken 5 min apart, which allowed the apparatus to rest.
Average molecular weight
Gel Permeation Chromatography (waters, USA) was used to measure the average molecular weight of chitosan, and equipped with a 2414 refractive index detector. The concentration of chitosan solution was 1 g/L in 2% acetate solution. The eluent solution was 0.2 mol/L acetate and 0.1 mol/L sodium acetate. Then the chitosan were eluted with a flow rate of 0.5 mL/min, column temperature was kept at 30 °C. The glucans (Mw 4400–670,000 Da) were used as standard samples.
Derivative thermogravimetric (DTG) analysis
Derivative thermogravimetric analysis was performed in TG 209F1 Iris thermal analysis instruments (Netzsch, Germany). 5 mg of chitosan powder was put into a special holder. The samples underwent a heat treatment with the protection of nitrogen (40 mL/min), the temperature was raised from 30–600 °C at a rate of 10 °C/min.
Results and discussion
Effects of glucose and xylose on fungal chitosan production
To compare the R. oryzae AS 3.819 fermentation in the medium containing glucose and xylose, 15 g/L glucose and 15 g/L xylose were used as carbon source, respectively. The effects of glucose and xylose on mycelia proliferation and L-lactic acid formation are shown in . R. oryzae AS 3.819 grew fast in the glucose medium. Glucose was completely fermented within 24 h ((A)). A short lag phase was observed at the initial fermentation. 2.36 g/L fungal biomass and 9.3 g/L lactic acid were detected in the broth at 72 h, while the chitosan extraction yield was 0.11 g/g biomass.
Xylose was consumed slower by R. oryzae AS 3.819 compared to glucose (as shown in (B)). 15 g/L xylose required 48 h of fermentation, and no lactic acid was detected in the culture broth. The formation of lactate product from xylose was probably influenced by fermentation conditions and R. oryzae strains. Mass et al. reported that the utilization of xylose was closely related to the C/N ratio, and the high C/N ratio could induce a metabolic pathway and promote cell growth [Citation25]. (B) showed that R. oryzae AS 3.819 grew slowly and had a long lag phase within 12 h. However, the biomass concentration of R. oryzae AS 3.819 increased dramatically, 4.33 g/L fungal biomass was obtained after 72 h ((B)), and the chitosan extraction yield was 0.13 g/g biomass. The experimental results showed that R. oryzae AS 3.819 had the metabolic capability to produce more fungal biomass using xylose as carbon source than glucose. The mycelium of R. oryzae AS 3.819 had been considered as an alternative source for chitosan production, which could be isolated easily by avoiding complicated treatment and reduced the environmental pollution than the chitosan production from the natural shellfish exoskeleton [Citation26].
Fungal chitosan production from mixture sugars and prehydrolysate
shows the curves of L-lactic acid and biomass from mixed sugars containing 30.0 g/L xylose and 10.0 g/L glucose. Xylose was consumed when the glucose titer reduced to 4.37 g/L. The low xylose uptake by cells, which might be caused by slower xylose transportation compared to that of glucose [Citation27] or strongly competitive inhibition by glucose [Citation28]. Glucose was firstly exhausted at 24 h, then exhaustion of xylose occurred at 48 h. The maximum R. oryzae AS 3.819 biomass reached 6.02 g/L with little L-lactic acid formation at 72 h. It demonstrated that the high biomass yield from xylose, it should be noted that cell respiration occupied high portion in the metabolic pathways, resulted in relatively low L-lactic acid yield [Citation25]. As shown in , glucose and xylose co-fermentation by R. oryzae AS 3.819 was regulated by the carbon catabolite repression, and glucose was preferentially metabolized compared to xylose [Citation28,Citation29].
The time course of the R. oryzae AS 3.819 fermentation in corn stover prehydrolysate is shown in . Corn stover prehydrolysate contain a lot of inhibitors, which were generated from dilute sulfuric acid-assisted steam explosion pretreatment [Citation30], and might have caused the negative effects on fermentation. Generally, lignocellulose hydrolysate must be detoxified before fermentation [Citation31]. As shown in , the inhibitor in the prehydrolysate showed no effects on R. oryzae AS 3.819 growth, which indicates that R. oryzae AS 3.819 can resist the effect of inhibitors. The biomass yield and the chitosan extraction yield were 10.96 g/L and 0.09 g/g biomass after 72 h fermentation in corn stover preyhdrolysate. This result displays that the biomass yield was higher than that of in mixed sugars medium (6.02 g/L). Tai et al. had reported that hemicellulose hydrolysate increased R. oryzae AS 3.819 growth by 10.2% compared to the growth in xylose medium [Citation3].
Characterization of fungal chitosan
Degree of deacetylation (DD)
DD of chitosan was one of the important characteristics for its application. Therefore, the DD of chitosan should be determined as accurately as possible [Citation26]. Some methods have been reported to determine the DD of chitosan, such as elemental analysis, titration, chromatography, IR and NMR spectroscopy [Citation7,Citation24,Citation32]. Titration was applied to determine the DD of fugal chitosan in the paper.
The results of DD from the R. oryzae AS 3.819 chitosan and the commercial chitosan were determined. The DD of fungal chitosan that derived from corn stover prehydrolysate (91.27%) was higher than that from glucose and xylose medium (86.50% and 88.54%), and also higher than that of the commercial chitosan (87.25%). The higher DD made fungal chitosan more suitable for food industrial application, particularly when it was used as an antimicrobial agent [Citation33].
Molecular weight and viscosity
The viscosity of chitosan from the different fermentation processes by R. oryzae AS 3.819 was in the range of 1.74–2.67 mPa.s, which was considerably lower than that of the commercial chitosan ( and ). Results were similar to the previous research that the viscosity of fungal chitosan ranged from 1.02–1.18 mPa.s [Citation34,Citation35].
Table 1. Viscosity and average molecular weight of the fungal chitosan.
Figure 4. The viscosity curves of the chitosan from different source.
GC: Chitosan produced from glucose medium at 72 h; XC: Chitosan produced from xylose medium at 72 h; LXC: Chitosan produced from corn stover prehydrolysate at 72 h; CC: Commercial chitosan.
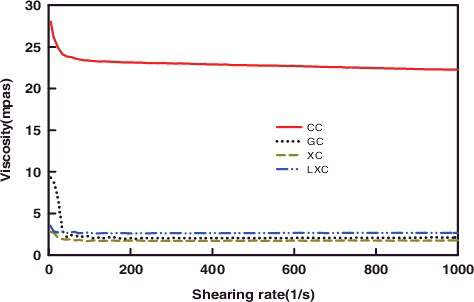
Viscosity is an important evaluated index of chitosan, which is closely associated with the molecular weight. The Mn and Mw of the fungal chitosan from corn stover prehydrolysate were 61.47 and 88.89 kDa, respectively, which corresponded to 27.00–190.00 kDa in previous published papers [Citation9]. The viscosity of the fungal chitosan was significantly lower than that of the commercial chitosan, which indicate that the molecular weight of fungal chitosan may be lower than that of the commercial chitosan. The polydispersity index (PD = Mw/Mn) was the most important parameter correlated with the molecular distribution of polysaccharides. The PD of the R. oryzae chitosan indicated that the fungal chitosan has a uniform molecular weight distribution.
IR spectra
shows the IR spectra of the fungal chitosan from different fermentations and the commercial chitosan. The fungal chitosan fermented from three medium and the commercial chitosan were extremely similar in IR spectra. In general, characteristic bands of chitosan at 3000–3500 cm−1 were usually attributed to intra- and intermolecular hydrogen bands [Citation36]. The absorption characteristic peaks at 2900 and 2860 cm−1 showed by spectra of the chitosan samples were attributed to C-H stretching. The characteristic peaks around 1650, 1550 cm and 1089 cm−1 of the chitosan samples were ascribed to the C = O stretching of amide I band, N-H bending vibration of amide II(-CONH-), and C-O stretching band, respectively. The band at 650 cm−1 was corresponded to -OH vibrations absorption out of plate, which might be due to the high DD of chitosan sample.
Derivative thermogravimetric (DTG)
illustrates the DTG curves for the chitosan samples at temperatures range from 30–600 °C. Two endothermic peaks were recorded. The first peak appeared around 50–130 °C corresponding to dehydration reaction, that the free and bound water was lost from the chitosan sample. The second peak emerged at about 294 °C, which was related to the decomposition of amine residues. The DD and molecular structure of chitosan might affect the endothermic peak area.
Conclusions
Glucose is a more favorable carbon source for most microorganisms compared to xylose. However, xylose was more suitable for chitosan preparation by R. oryzae fermentation than glucose. Lignocellulosic prehydrolysate from dilute acid-assisted steam explosion pretreatment containing quantities of inhibitors and xylose, which could be effectively applied for R. oryzae proliferation without detoxification, and further used for the preparation of fungal chitosan. The fungal biomass and chitosan extraction yield were 10.96 g/L and 0.09 g/g biomass, respectively. The IR and DTG characteristics of the fungal chitosan and the commercial chitosan extracted from natural shellfish exoskeleton were similar. However, the degree of deacetylation of fungal chitosan that derived from corn stover prehydrolysate was higher than that of the commercial chitosan, and the viscosity of the fungal chitosan was much lower than that of the commercial chitosan.
Disclosure statement
No potential conflict of interest was reported by the authors.
Additional information
Funding
References
- Kittur F, Prashanth KH, Sankar KU, et al. Characterization of chitin, chitosan and their carboxymethyl derivatives by differential scanning calorimetry. Carbohyd Polym. 2002;49:185–193.
- Kasaai M. A review of several reported procedures to determine the degree of N-acetylation for chitin and chitosan using infrared spectroscopy. Carbohyd Polym. 2008;71:497–508.
- Tai C, Li S, Xu Q, et al. Chitosan production from hemicellulose hydrolysate of corn straw: impact of degradation products on Rhizopus oryzae growth and chitosan fermentation. Lett Appl Microbiol. 2010;51:278–284.
- Rabea EI, Badawy ME-T, Stevens CV, et al. Chitosan as antimicrobial agent: applications and mode of action. Biomacromolecules. 2003;4:1457–1465.
- Silva HS, dos Santos KS, Ferreira EI. Chitosan: hydrossoluble derivatives, pharmaceutical applications and recent advances. Quim Nova. 2006;29:776–785.
- Synowiecki J, Al-Khateeb NA. Production, properties, and some new applications of chitin and its derivatives. Crit Rev Food Sci Nutr. 2003;43:145–171.
- Yen M-T, Mau J-L. Physico-chemical characterization of fungal chitosan from shiitake stipes. LWT – Food Sci Technol. 2007;40:472–479.
- Rungsardthong V, Wongvuttanakul N, Kongpien N, et al. Application of fungal chitosan for clarification of apple juice. Process Biochem. 2006;41:589–593.
- Pochanavanich P, Suntornsuk W. Fungal chitosan production and its characterization. Lett Appl Microbiol. 2002;35:17–21.
- Ghosh B, Ray RR. Current commercial perspective of Rhizopus oryzae: a review. J Appl Sci. 2011;11:2470–2486.
- Park EY, Anh PN, Okuda N. Bioconversion of waste office paper to L(+)-lactic acid by the filamentous fungus Rhizopus oryzae. Bioresour Technol. 2004;93:77–83.
- Kobayashi H, Ohta H, Fukuoka A. Conversion of lignocellulose into renewable chemicals by heterogeneous catalysis. Catal Sci Technol. 2012;2:869.
- Peng P, She D. Isolation, structural characterization, and potential applications of hemicelluloses from bamboo: a review. Carbohyd Polym. 2014;112:701–720.
- Karimi K, Emtiazi G, Taherzadeh MJ. Ethanol production from dilute-acid pretreated rice straw by simultaneous saccharification and fermentation with Mucor indicus, Rhizopus oryzae, and Saccharomyces cerevisiae. Enzyme Microb Technol. 2006;40:138–144.
- Smith J, Van Rensburg E, Görgens JF. Simultaneously improving xylose fermentation and tolerance to lignocellulosic inhibitors through evolutionary engineering of recombinant Saccharomyces cerevisiae harbouring xylose isomerase. BMC Biotechnol. 2014;14:41.
- Chandel AK, da Silva SS, Singh OV. Detoxification of lignocellulose hydrolysates: biochemical and metabolic engineering toward white biotechnology. Bioenerg Res. 2013;6:388–401.
- Tomek KJ, Saldarriaga CR, Velasquez FP, et al. Removal and upgrading of lignocellulosic fermentation inhibitors by in situ biocatalysis and liquid-liquid extraction. Biotechnol Bioeng. 2015;112:627–632.
- Yong Q, Li X, Yuan Y, et al. An improved process of ethanol production from hemicellulose: bioconversion of undetoxified hemicellulosic hydrolyzate from steam-exploded corn stover with a domesticated Pichia stipitis. Appl Biochem Biotechnol. 2012;167:2330–2340.
- Zhu J, Yang J, Zhu Y, et al. Cause analysis of the effects of acid-catalyzed steam-exploded corn stover prehydrolyzate on ethanol fermentation by Pichia stipitis CBS 5776. Bioprocess Biosyst Eng. 2014;37:2215–2222.
- Chatterjee S, Das SK, Chakravarty R, et al. Interaction of malathion, an organophosphorus pesticide with Rhizopus oryzae biomass. J Hazard Mater. 2010;174:47–53.
- Chatterjee S, Chatterjee S, Chatterjee BP, et al. Enhancement of growth and chitosan production by Rhizopus oryzae in whey medium by plant growth hormones. Int J Biol Macromol. 2008;42:120–126.
- Kumaresapillai N, Basha RA, Sathish R. Production and evaluation of chitosan from Aspergillus niger MTCC strains. Iran J Pharm Res. 2011;10:553.
- Zamani A, Jeihanipour A, Edebo L, et al. Determination of glucosamine and N-acetyl glucosamine in fungal cell walls. J Agric Food Chem. 2008;56:8314–8318.
- Czechowska-Biskup R, Jarosińska D, Rokita B, et al. Determination of degree of deacetylation of chitosan-comparison of methods. Prog Chem Appl Chitin Deriv. 2012;17:5–20.
- Maas RH, Springer J, Eggink G, et al. Xylose metabolism in the fungus Rhizopus oryzae: effect of growth and respiration on L+-lactic acid production. J Ind Microbiol Biotechnol. 2008;35:569–578.
- Di Mario F, Rapana P, Tomati U, et al. Chitin and chitosan from Basidiomycetes. Int J Biol Macromol. 2008;43:8–12.
- Maas RH, Bakker RR, Eggink G, et al. Lactic acid production from xylose by the fungus Rhizopus oryzae. Appl Microbiol Biotechnol. 2006;72:861–868.
- Zhang M, Jiang St, Zheng Z, et al. Cloning, expression, and characterization of a novel xylose reductase from Rhizopus oryzae. J Basic Microbiol. 2015;55:907–921.
- Saito K, Hasa Y, Abe H. Production of lactic acid from xylose and wheat straw by Rhizopus oryzae. J Biosci Bioeng. 2012;114:166–169.
- Olsson L, Hahn-Hägerdal B. Fermentation of lignocellulosic hydrolysates for ethanol production. Enzyme Microb Tech. 1996;18:312–331.
- Lee KM, Min K, Choi O, et al. Electrochemical detoxification of phenolic compounds in lignocellulosic hydrolysate for Clostridium fermentation. Bioresour Technol. 2015;187:228–234.
- Sato H, Mizutani S-i, Tsuge S, et al. Determination of the degree of acetylation of chitin/chitosan by pyrolysis-gas chromatography in the presence of oxalic acid. Anal. Chem. 1998;70:7–12.
- Tayel AA, Moussa S, Opwis K, et al. Inhibition of microbial pathogens by fungal chitosan. Int J Biol Macromol. 2010;47:10–14.
- Dakia PA, Blecker C, Robert C, et al. Composition and physicochemical properties of locust bean gum extracted from whole seeds by acid or water dehulling pre-treatment. Food Hydrocolloids. 2008;22:807–818.
- Dhillon GS, Kaur S, Sarma SJ, et al. Integrated process for fungal citric acid fermentation using apple processing wastes and sequential extraction of chitosan from waste stream. Ind Crops Prod. 2013;50:346–351.
- Kucukgulmez A, Celik M, Yanar Y, et al. Physicochemical characterization of chitosan extracted from Metapenaeus stebbingi shells. Food Chem. 2011;126:1144–1148.