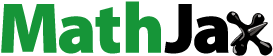
ABSTRACT
The peptides with angiotensin-I converting enzyme (ACE)-inhibition activity were prepared from Perinereis aibuhitensis protein (PAP) by hydrolysis with neutral protease, in which the ACE-inhibition rate of peptides derived from PAP was monitored. The hydrolysis conditions were optimised as follows: reaction time 5 h, temperature 50 °C, pH 7 and enzyme amount 0.5%. Under the optimum hydrolysis conditions, the ACE-inhibition rate of peptides of PAP reached up to 83.61%. The hydrolysates were fractionated into two molecular-weight ranges (below and above 10 kDa) by ultrafiltration. The below-10-kDa fraction with higher ACE-inhibitory was subsequently purified by Sephadex G-15 gel filtration chromatography. The structure of the active peptide was identified as Gly-Ala-Phe by high performance liquid chromatography (HPLC) coupled with quadrupole (Q) time-of-flight (TOF) mass spectrometry (HPLC-Q-TOF-MS). Thus, the peptide prepared from PAP by hydrolysis with neutral protease could be a beneficial ingredient of nutraceuticals and pharmaceuticals against hypertension.
Introduction
Hypertension is the most common serious chronic health problem and a high risk factor for myocardial infarction, arteriosclerosis, stroke and end-stage renal disease and affects 15% to 20% of adults worldwide [Citation1,Citation2]. Angiotensin-I converting enzyme (ACE; EC 3.4.15.1) is a Zn-metallopeptidase which plays an important role in regulating blood pressure and hypertension [Citation3]. Inhibition of ACE is an important therapeutic approach to controlling hypertension. ACE inhibitors are often used to treat myocardial infarction, hypertension and other cardio-related diseases [Citation4].
ACE inhibitors are divided into two groups, i.e. synthesised ACE inhibitors and natural ACE inhibitors. While ACE inhibitors are widely used in the treatment of cardiac diseases, effects like cough and angioedema are direct consequences of the inhibition of ACE and are invariably associated with ACE-inhibiting compounds. Synthetic ACE inhibitors may exert additional adverse side effects such as allergic reactions, taste disturbances, renal impairment, skin rashes, etc. [Citation5–7]. Therefore, some authors view natural ACE inhibitors with non-toxicity as potentially safe and economical alternatives to synthetic ones [Citation6,Citation8].
Many peptides derived from proteins have been reported to have ACE-inhibitory activities: the peptides from terrestrial plant proteins, terrestrial animal proteins, aquatic animal proteins, shellfish proteins and aquatic plant proteins [Citation9,Citation10]. However, data regarding ACE-inhibitory peptides derived from Perinereis aibuhitensis are limited.
The present work aimed to prepare ACE-inhibitory peptides derived from P. aibuhitensis protein (PAP) by hydrolysis with neutral protease, in which the optimum hydrolysis conditions and partial characteristics of the peptides were investigated.
Materials and methods
Materials and reagents
P. aibuhitensis (1.4–2.1 g wet weight) were collected in the tidal deposition flat sediments from the Xinyihe estuary, Lianyungang, China. The animals were transferred from the field to an ice cooler, along with sediments from the sampling site. Acetonitrile and trifluoroacetic acid (TFA) were HPLC (high performance liquid chromatography) grade (Thermo Fisher Scientific Inc., Shanghai, China). Neutral protease, with an enzymatic activity of 100,000 U/g, was purchased from Shanghai Luan Biological Technology Co., Ltd. (Shanghai, China). Captopril, a reference agent, was purchased from Shanghai Ziqi Biological Technology Co., Ltd. (Shanghai, China). Other reagents were analytical grade and were purchased from Sinopharm Chemical Reagent Company (Shanghai, China).
Preparation of PAP
P. aibuhitensis were washed with tap water, cut into thin sections and washed three times with five volumes of 0.1 mol/L KCl–20 mmol/L Tris–maleate buffer (pH 7.0). After adding three volumes of KCl–Tris–maleate buffer, the specimens were homogenised by using a homogeniser (GF-1, Beijing Zhongyi Zhonghe Biotechnology Co., Ltd, Beijing, China). The homogenate was filtered through a nylon net (#16) to remove the connective tissues, added 20% Triton X-100 to adjust the final concentration to 1%, allowed to stand for 30 min and centrifuged at 750 × g for 10 min. The sediment was washed with 5 volumes of KCl–Tris–maleate buffer and centrifuged again four times, mixed with five volumes of cold distilled water, washed by stirring, centrifuged at 4,000 × g for 10 min, centrifuged again at 25,000 × g for 20 min and freeze dried.
Preparation of protein hydrolysates from P. aibuhitensis
Five grams of PAP was mixed with 1000 mL distilled water. The mixture was adjusted to different pH (6–8) and added to specific amount of neutral protease (0.1%–0.9%). Enzymatic hydrolysis was carried out at different temperature (40–60 °C) for different time (3–7 h). The enzyme process was stopped by boiling for 10 min. The mixture was centrifuged at 5,000 × g (4 °C for 10 min). The supernatant was concentrated with a vacuum concentrator and stored at −20 °C until use.
Determination of ACE-inhibitory activity
The ACE-inhibitory activity was determined according to the modified spectrophotometric method as described by Pan et al. [Citation11]. The degree of ACE inhibition (in percentage) was calculated according to EquationEquation (1)(1)
(1) :
(1)
(1) where Ab is the absorbance without inhibitor (blank), As is the absorbance of the reaction mixture (sample) and Ac is the absorbance of the buffer (control). The activity of each sample was tested in triplicate. The half maximal inhibitory concentration (IC50), i.e. the concentration of peptide in milligrams per milliliter required to reduce 50% of ACE activity, was determined by regression analysis of ACE inhibition (%) versus peptide concentration. Peptide concentration was determined according to the method of Lu et al. [Citation12] using l-glutathione oxide (Sigma Chemical Co., St. Louis, MO, USA) as a standard.
Purification of ACE-inhibitory peptides from hydrolysates
The hydrolysate solution was passed through an ultrafiltration membrane with a 10 kDa molecular-weight (MW) cut-off on a membrane separation system (QuixStand™ Benchtop System, GE Healthcare Bio-Sciences Corp., Shanghai, China), Sephadex G-15 column (Φ1 cm × 60 cm) at a flow rate of 0.3 mL/min using a Biologic DuoFlow Pathfinder™ 20/80 system (Bio-Rad Laboratories, Inc., Shanghai, China) and semi-prep reversed phase high-performance liquid chromatography (RP-HPLC) equipped with a Sunfire™ C18 column as described by Pan et al. [Citation11].
Determination of the amino-acid sequence of the purified peptide
The molecular mass and amino-acid sequence of the purified peptide from P. aibuhitensis protein was determined by using an high performance liquid chromatography (HPLC) coupled with quadrupole (Q) time-of-flight (TOF) mass spectrometry (HPLC-Q-TOF-MS) mass spectrometer (WATERS MALDI SYNAPT Q-TOF MS, Waters, USA) equipped with an electrospray ionisation (ESI) source according to the method of Himaya et al. [Citation13].
Statistical analysis
Analysis of variance (ANOVA) was performed with SPSS 13.0 software (Armonk, NY, USA). Linear regression was performed using Microsoft Excel 2003 (Microsoft Corp., Seattle, WA, USA).
Results and discussion
Effect of time, temperature, pH and enzyme amount on the rate of ACE inhibition by PAP-derived peptide
Reaction time is important for efficient hydrolysis of PAP with neutral protease. The reaction temperature and pH of a reaction mixture crucially affect the neutral protease activity and ACE inhibition rate of peptide derived from PAP and hydrolysis of PAP by neutral protease. The enzyme amount is also important for efficient hydrolysis of PAP. In this study, the optimum hydrolysis conditions were determined to be: reaction time 5 h ((a)), temperature 50 °C ((b)), pH 7 ((c)) and enzyme amount 0.5% ((d)). Under the optimum hydrolysis conditions, the ACE-inhibition rate of PAP-derived peptides reached up to 83.61%. Prolonged reaction time and temperature that is too low or pH that is higher led to a decrease in the rate of ACE inhibition by PAP-derived peptide, while larger enzyme amounts did not further increase the ACE inhibition rate of peptide derived from PAP ()).
Purification of ACE-inhibitory peptide from PAP
PAP was firstly separated by ultrafiltration, giving two fractions with molecular weight (MW) below and above 10 kDa. The below-10-KDa fraction had higher ACE-inhibitory activity, with IC50 = 0.19 ± 0.013 mg/mL, while the above-10-kDa fraction showed lower activity with IC50 = 14.61 ± 0.24 mg/mL, indicating that the MWs of most of the ACE-inhibitory peptides in the hydrolysates were less than 10 kDa. The below-10-KDa fraction was loaded into the Sephadex G-15 column, and three fractions were obtained (), among which fraction C had the highest ACE-inhibitory activity (IC50 = 0.052 ± 0.006 mg/mL). Therefore, fraction C was further separated by semi-preparative HPLC on a Zorbax C18 column, giving two peaks at 228 nm (). Peak 1 corresponded to lower ACE-inhibitory activity with IC50 = 0.071 ± 0.005 mg/mL, while Peak 1 showed higher activity with IC50 = 0.0062 ± 0.004 mg/mL. This was, however, lower than that (0.021 µmol/L) of captopril, the most widely used antihypertensive drug at present. Peak 1 with the higher ACE inhibitory activity was applied on a Sunfire™ C18 column RP-HPLC. The RP-HPLC spectrum showed a major peak after analysis of the purity of Peak 1 (), suggesting that this fraction had a satisfactory purity for amino acid sequencing.
Figure 2. Chromatogram of the fraction with MW below 10 kDa of Perinereis aibuhitensis protein hydrolysates on a Sephadex G-15 column.
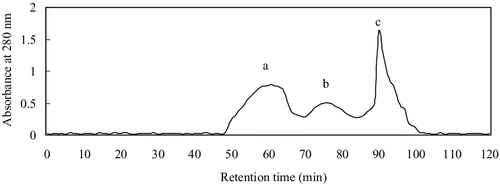
Amino-acid sequence of the purified peptide
The amino acid sequence of the purified peptide was determined to be Phe–Ala–Val by Q-TOF ESI MS (data not shown). In recent years, many peptides with ACE-inhibitory activity have been prepared from the proteins of various organisms, e.g. Enteromorpha clathrata [Citation11], Pacific cod (Gadus macrocephalus) skin gelatin [Citation13], Grass carp protein [Citation14], Agaricus bisporus [Citation15], fermented shrimp pastes [Citation16], silkworm pupa (Bombyx mori) protein [Citation17], lizard fish protein hydrolysates [Citation18], flounder fish (Paralichthys olivaceus) muscle [Citation19], sweet sorghum grain protein [Citation20] and cauliflower by-products protein hydrolysate [Citation21]. The amino-acid sequences of these ACE-inhibitory peptides are summarized in . The results indicate that the peptide obtained in this work had a different amino-acid sequence from other reported ACE-inhibitory peptides and thus was a novel peptide. Although the relationship between the structure and activity of ACE-inhibitory peptides has not yet been established, Kong et al. [Citation22] found that ACE-inhibitory peptides showed some common features: the structure–activity relationships among different ACE-inhibitory peptides suggest that binding to ACE is strongly dependent on the C-terminal tripeptide sequence of the substrate.
Table 1. Structure of ACE inhibitory peptides from various organisms.
In this study, we identified a novel ACE-inhibitory peptide with an amino-acid sequence of PAV from PAP hydrolysate containing a hydrophobic amino acid sequence at the C-terminal tripeptide sequence. This PAP derived ACE-inhibitory peptide could be considered as a potentially beneficial ingredient of nutraceuticals and pharmaceuticals against hypertension. However, further trials on evaluating the toxic potential, allergenic potential, etc., are necessary.
Conclusions
We prepared a novel ACE-inhibitory peptide (PAV) from PAP hydrolysates. The ACE-inhibition rate of the peptides was affected by reaction time, temperature, pH and enzyme amount. Hydrolysates from PAP are promising candidates for industrial production of functional peptides for blood level regulation for hypertensive patients.
Disclosure statement
The authors have declared that no competing interests exist.
Additional information
Funding
References
- Jung WK, Mendis E, Je JY, et al. Angiotensin I converting enzyme inhibitory peptide from yellowfin sole (Limanda aspera) frame protein and its antihypertensive effect in spontaneously hypertensive rats. Food Chem. 2006;94:26–32.
- Wang J, Hu J, Cui J, et al. Purification and identification of a ACE inhibitory peptide from oyster proteins hydrolysate and the antihypertensive effect of hydrolysate in spontaneously hypertensive rats. Food Chem. 2008;111:302–308.
- Skeggs LT, Kahn JE, Shumway NP. The preparation and function of the angiotensin I-converting enzyme. J Exp Med. 1956;103:295–299.
- Raghavan S, Kristinsson HG. ACE-inhibitory activity of tilapia protein hydrolysates. Food Chem. 2009;117:582–588.
- Antonios TFT, MacGregor GA. Angiotensin converting enzyme inhibitors in hypertension: potential problems. J Hypertens. 1995;13:S11–S16.
- Bougatef A, Nedjar-Arroume N, Ravallec-Plé R, et al. Angiotensin I-converting enzyme (ACE) inhibitory activities of sardinelle (Sardinella aurita) by-products protein hydrolysates obtained by treatment with microbial and visceral fish serine proteases. Food Chem. 2008;111:350–356.
- Lahogue V, Réhel K, Taupin L, et al. A HPLC-UV method for the determination of angiotensin I-converting enzyme (ACE) inhibitory activity. Food Chem. 2010;118:870–875.
- Qian ZJ, Jung WK, Lee SH, et al. Antihypertensive effect of an angiotensin I-converting enzyme inhibitory peptide from bullfrog (Rana catesbeiana Shaw) muscle protein in spontaneously hypertensive rats. Process Biochem. 2007;42:1443–1448.
- Suetsuna K, Chen JR. Identification of antihypertensive peptides from peptic digest of two microalgae, Chlorella vulgaris and Spirulina platensis. Marine Biotechnol. 2001;3:305–309.
- Suetsuna K, Nakano T. Identification of an antihypertensive peptide from peptic digest of wakame (Undaria pinnatifida). J Nutr Biochem. 2000;11:450–454.
- Pan SK, Wang SJ, Jing LL, et al. Purification and characterisation of a novel angiotensin-I converting enzyme (ACE)-inhibitory peptide derived from the enzymatic hydrolysate of Enteromorpha clathrata protein. Food Chem. 2016;211:423–430.
- Lu W, Ren GP, Song JM. [Determination of content of peptides in protein hydrolysates]. Food Sci. 2005;26:169–171. Chinese
- Himaya SWA, Ngo DH, Ryu B, et al. An active peptide purified from gastrointestinal enzyme hydrolysate of Pacific cod skin gelatin attenuates angiotensin-1 converting enzyme (ACE) activity and cellular oxidative stress. Food Chem. 2012;132:1872–1882.
- Chen J, Wang Y, Zhong Q, et al. Purification and characterization of a novel angiotensin-I converting enzyme (ACE) inhibitory peptide derived from enzymatic hydrolysate of grass carp protein. Peptides. 2012;33:52–58.
- Lau CC, Abdullah N, Shuib AS, et al. Novel angiotensin I-converting enzyme inhibitory peptides derived from edible mushroom Agaricus bisporus (J.E. Lange) Imbach identified by LC–MS/MS. Food Chem. 2014;148:396–401.
- Kleekayai T, Harnedy PA, O'Keeffe MB, et al. Extraction of antioxidant and ACE inhibitory peptides from Thai traditional fermented shrimp pastes. Food Chem. 2015;176:441–447.
- Wu QY, Jia JQ, Yan H, et al. A novel angiotensin-I converting enzyme (ACE) inhibitory peptide from gastrointestinal protease hydrolysate of silkworm pupa (Bombyx mori) protein: biochemical characterization and molecular docking study. Peptides. 2015;68:17–24.
- Lan XD, Liao DK, Wu SG, et al. Rapid purification and characterization of angiotensin converting enzyme inhibitory peptides from lizard fish protein hydrolysates with magnetic affinity separation. Food Chem. 2015;182:136–142.
- Ko JY, Kang N, Lee JH, et al. Angiotensin I-converting enzyme inhibitory peptides from an enzymatic hydrolysate of flounder fish (Paralichthys olivaceus) muscle as a potent anti-hypertensive agent. Process Biochem. 2016;51:535–541.
- Wu QY, Du JJ, Jia JQ, et al. Production of ACE inhibitory peptides from sweet sorghum grain protein using alcalase: hydrolysis kinetic, purification and molecular docking study. Food Chem. 2016;199:140–149.
- Xu Y, Bao T, Han W, et al. Purification and identification of an angiotensin I-converting enzyme inhibitory peptide from cauliflower by-products protein hydrolysate. Process Biochem. 2016;51:1299–1305.
- Kong X, Zhou H, Qian H. Enzymatic preparation and functional properties of wheat gluten hydrolysates. Food Chem. 2007;101:615–620.