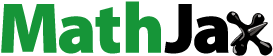
ABSTRACT
Bacterial cellulose (BC) is a nanostructured material mainly produced by Gluconacetobacter. The excellent physicochemical and mechanical properties of BC have made it become regarded as a kind of highly functional biopolymer in the application fields of bio-medicine, cosmetics and food industry. Using low-cost carbon sources as raw materials could make BC production more cost-effective. In this study, the pullulan fermentation wastewater with high chemical oxygen demand (COD) value of 39040 mg/L was used as substrate for BC production. After 10 days of fermentation, a BC yield of 1.177 g/L in wastewater was obtained, lower than that (1.757 g/L) produced in Hestrin-Schramm (HS) media. During the fermentation process, 59.49% of monosaccharide coupling with 18.9% of COD in wastewater decreased. There were no differences in the functional groups and crystal patterns of the BC samples produced in wastewater and HS medium. However, more pores and loose three-dimensional networks were observed in the microstructure of BC samples produced in wastewater, resulting in lower crystallinity (56.23%) and Young's modulus (3984.29 MPa) compared to that obtained from HS media. It indicated the nutrients composition of fermentation wastewater showed obvious effects on BC microstructure and mechanical properties. Our work demonstrated the possibility of using polysaccharide fermentation wastewater as inexpensive carbon source for BC production. Further study should be done in the improvements of the physical and chemical properties of BC sheets.
Introduction
Bacterial cellulose (BC) is a nano-structured material synthesized by some species of bacteria belonging to the genera Aerobacter, Agrobacterium, Rhizobium, Gluconacetobacter (presently Komagataeibacter), Acetobacter, Sarcina and Pseudomonas [Citation1]. However, as far as known, only Gluconacetobacter could produce high-quality cellulose efficiently and have the potential for commercial use [Citation2]. Like plant cellulose, the chemical composition of BC is that of connected glucose molecules via acetal linkages between C1 and C4 carbons. However, the physicochemical and mechanical properties of BC are different due to the uniform, continuous and nano-scalar network of cellulosic fibers (1–5 nm). BC is characterized by higher purity, higher degree of polymerization, higher crystallinity, higher water-absorbing and holding capacity, higher tensile strength and good biocapatibility, compared to plant cellulose [Citation3,Citation4]. These enhanced properties have made BC become considered a kind of highly functional biopolymer which has application potential in bio-medicine, cosmetics, high-end acoustic diaphragms, papermaking, food industry and other areas [Citation5,Citation6].
Although BC has excellent potential as material in many novel applications, the low yield and high production cost hinder its industrial-scale production and broad range of application. Therefore, looking into inexpensive feedstock as the culture medium is helpful to make BC production more cost-effective [Citation7].
Low-cost industrial fermentation wastewater, especially the polysaccharide fermentation waste, is rich in extracellular polysaccharides, low-molecular weight sugars, glycerol, proteins, vitamins and other nutrients, resulting in high chemical oxygen demand (COD) values. Traditionally, fermentation wastewater is treated by activated sludge. However, this method requires some special equipment such as Up-flow Anaerobic Sludge Blanket (UASB) [Citation8]; besides, the fermentable compounds in wastewater cannot be utilized to get higher value-added products. Therefore, using the cheap fermentation wastewater as a good substrate to cultivate BC-producing strains can make the cellulose production more cost-effective and decrease the environmental pollution associated with the disposal of industrial wastes.
To achieve rational utilization of polysaccharide fermentation waste, the possibility of using this substrate for BC production was initially evaluated. In this study, the COD of the wastewater was monitored during the cultivation of the BC-producing strain. The variations of the BC product in micro-structure, yields and mechanical properties were also studied. This research proved the possibility of bioconversion from low-cost carbohydrate waste (polysaccharides fermentation wastewater) to high value-added bio-polymer (BC).
Materials and methods
Micro-organism, medium and growth conditions
The BC-producing strain Gluconacetobacter xylinum BC-11 was employed in this study. This strain has been isolated from grape fruit surface in Dazeshan coteau in Qingdao and has a strong ability to produce BC using glycerol and various carbohydrates such as glucose, sucrose and fructose. The strain was maintained on Hestrin & Schramm medium (HS) agar slants containing: 20 g/L glucose, 5 g/L yeast extract, 5 g/L peptone, 2.7 g/L Na2HPO4, 1.15 g/L citric acid and 20 g/L agar. The strain was stored at 4 °C in a refrigerator and sub-cultured every 1 month for seed culture. The wastewater after pullulan polysaccharide fermentation without adding any other nutrients was provided by our laboratory and was adopted as the medium (initial pH 7.0) for BC production. To develop the inoculum, 5 mL HS medium in a test tube with G. xylinum BC-11 was incubated statically for 1 week at 30 °C. The obtained pellicle was treated by adding 0.1% Cellic® Ctec2 (Ctec2) cellulose obtained from Novozymes (China) Biotechnology Co., Ltd. to release the wrapped cells in the net structure of the cellulose produced. The cells were harvested by centrifugation at 5000 × g and washed three times to remove the cellulose and re-suspended in fresh medium. Ten percent (v/v) of the prepared seed culture was transferred into the fermentation wastewater and/or HS medium and the fermentation was carried out at 30 °C for 10 days. All the experiments were performed in triplicate and an average value of BC yield was given.
Determination of the reducing sugar in the culture medium
The reducing sugar content in the fermentation wastewater was measured by using SBA-40 Biosensor Analyzer (Institute of Biology, Shandong Academy of Sciences, Shandong Province, Ji'nan). The samples were taken and centrifuged at 5000 × g to remove the solid impurities and cells. Twenty-five microliter samples were injected into the Biosensor Analyzer to determine the content of reducing sugar. The Biosensor Analyzer was calibrated using standard glucose solution (1.0 g/L) before use. To compare the differences in the cellulose micro-structure and mechanical properties, the reducing sugar content in HS medium and fermentation wastewater were adjusted to 150 g/L before fermentation.
Determination of COD in wastewater
The COD in the fermentation wastewater was measured by using 5B-3B Water Quality Analyzer (Lian-hua Technology Co., Ltd, Beijing) as per the operating instructions. The samples were taken and centrifuged at 5000 × g to remove the solid impurities and cells before use. Distilled water was used to prepare the control group for background correction.
BC purification and quantification
After cultivation, the BC membranes were rinsed three times with deionized water and then soaked in boiling 2% (w/v) NaOH solution for 1 h to remove and dissolve the bacteria cells entrapped in the microfibers. After turning transparent, the BC membranes were washed with deionized water several times to make the membranes neutralized. The purified cellulose was dried at 60 °C overnight and weighted. For each membrane, triplicate experiments were performed, and the mean values were calculated.
BC membrane analysis
The micro-structure observation of BC membranes
The Hitachi S-4800 field emission scanning electronic microscope (SEM) (Hitachi High-Technologies Corporation, Japan) was employed to observe the micro-structure of the BC produced in HS medium and wastewater. The air-dried BC membranes were coated with carbon using an ion sputter coater (Hitachi E-1045, Japan) and photographed at room temperature at 5.0 kV.
Fourier transforms infrared spectroscopy (FT-IR) analysis
Fourier transform infrared spectrometer (FT-IR) Nicolet iN10 equipped with the secondary module iZ10 (both purchased from Thermo Fisher Scientific Inc.) were employed to analyze the surface properties of the BC samples. The FT-IR spectra were recorded in spectral ranges of 4000–450 cm−1 at a resolution of 4 cm−1 with 32 scans. BC sample pellets were prepared by mixing with spectroscopic grade KBr and pressing in a standard device using a pressure of 200 bars. Data were analyzed with the OMNIC software v. 8.2.0.387.
Crystallinity degree of BC
X-ray diffractometer (XRD) (D8 ADVANCE-TXS, Bruker AXS, Germany) was employed to calculate the crystallinity of BC membranes. The X-ray diffraction patterns were recorded at room temperature using Ni-filtered Cu Kα radiation (λ = 1.54 Å). The operating voltage and electrical current were 40 kV and 40 mA, respectively. Data were collected at 2 degree per minute from 5 to 60 degree 2θ. MDI Jade 6 software (Materials Data, Inc., Livermore, CA, USA) was used to analyze the diffraction pattern and to calculate the crystallinity of BC. The degree of crystallinity was calculated from the diffracted intensity data using the empirical method proposed by Segal et al. [Citation9]:where I200 is the overall intensity of the peak at 2θ = 22.9 and Iam is the intensity of the baseline at 2θ = 18°.
The crystal size was calculated by the Scherrer equation [Citation10]:where β is the full width at half maximum (FWHM) of a specific phase (hkl), k is a constant that varies in the range from 0.89 to 1 based on the breadth adopted, λ is the wavelength of incident x-ray, and θ is the center angle of the peak.
Physical properties of BC
The mechanical properties of BC produced in HS medium and fermentation wastewater were compared using a CMT6503 electronic universal tensile tester (MTS Industrial Systems Co., Ltd., Shenzhen, China) on which the Young's modulus and breaking elongation values were determined. Tests were performed using a gauge length of 20 mm and at a strain rate of 10 mm/min.
Data analysis
Values are presented as means with standard deviation (±SD) from at least three independent experiments. Fisher's LSD method was used in analysis of variance (ANOVA) to define confidence intervals for all pairwise differences between factor level means. Differences were considered statistically significant at P < 0.05.
Results and discussion
BC production, sugar utilization and COD degradation during fermentation
Pullulan polysaccharide fermentation wastewater without any pretreatment was used as a low-cost culture medium for BC production. In order to obtain BC membranes, static fermentation was employed in this research. After inoculation, BC production and sugar utilization were measured along with the fermentation process. As shown in , no obvious BC pellicle could be observed on the culture medium surface in the flask during the first three days of fermentation, indicating that the growth of the BC-producing strain was in the lag phase. At Day 4, clear white BC membranes were shown on the medium surface and the dry weight yields in wastewater and HS medium were about 0.2 and 0.6 g/L, respectively. Before Day 8, the BC production rate in the wastewater was only 0.11 g/(L day), which was less than that in HS medium (0.29 g/(L day)). The maximum yields in wastewater and HS medium were 1.177 and 1.757 g/L at Day 10. Other cellulosic wastes from renewable non-food residues have also been used to produce BC. For example, konjac powder and olive mill residue hydrolysates have been used for BC production; however, the yields of BC were lower than that obtained in our study [Citation11,Citation12]. In addition, the raw materials need to be hydrolyzed to release the fermentable sugar, which might increase the cost of the BC production. This suggests that pullulan polysaccharide fermentation wastewater without any pretreatment could be reused as a microbial nutrients source for BC production, although the production rate and final yields were lower than that in the HS medium.
The residual sugar content was also monitored during the fermentation and sharp decreases were found in both of the two different media, as shown in . At the first 4 days, about 50 g/L and about 40 g/L reducing sugar were utilized in HS medium and wastewater, respectively, even though no BC were produced during this period. The consumed sugar might be metabolized for cell recovery and growth during the lag phase [Citation13]. During the BC-producing phase, the sugar content in the HS medium and wastewater continued to decline with an average rate of 6.25 and 7.5 g/(L day), respectively. At Day 10, there was also about 55 g/L residual sugar content both in the wastewater and HS medium. After removal of the BC pellicle from the culture medium, another round of fermentation could be done without inoculation and any nutrients addition (data not shown).
Fermentation wastewater is with high organic carbon content and other nutrients, which is an inexpensive substrate for microbial growth [Citation14]. Making use of such wastewater for production of high value-added chemicals would not only lower the production cost, but also reduce the pollution of the environment associated with the disposal of fermentation wastewater. In this study, the possibility of using pullulan polysaccharide fermentation wastewater for BC production was initially studied. As shown in , the COD in wastewater decreased sharply from 39 040 to 31 652.5 mg/L with a decrease ratio of 18.9% during the 10 days of fermentation, indicating that reuse of fermentation wastewater for BC production could be an economical method for wastewater treatment. However, we also should notice that the BC yield and COD reduction were still low, and the residual sugar content (about 55 g/L) and COD value (approximately 30 000 mg/L) in the reused fermentation wastewater were still high. Further study should focus on the strain selection and fermentation process optimization to improve the BC yields and COD reduction [Citation15,Citation16].
Comparisons of BC structures produced in wastewater and HS medium
Material micro-structures are always linked to the mechanical properties and applications resulting from the chemical composition, arrangements and processing conditions [Citation17]. In this study, the micro-structures of BC produced in wastewater and HS medium were compared by using SEM, FT-IR and XRD.
The surface and inner matrix of BC sheets were analyzed through SEM analysis, and the results are shown in . The microstructure of the BC produced in HS medium in this work was similar to the one previously reported [Citation16]. However, the microstructures of BC produced in HS medium were different from the one produced in the wastewater in our study. At the initial stage of fermentation (Day 4), clear networks of cellulose fibers produced in both of the two different culture media were observed; however, the space among the network of the BC samples was significantly different ((a,c)). BC produced in wastewater had relatively wider space with obvious pores in the inner matrix and thinner cellulose fibers (ranging from 0.01 to 0.1 μm). Random and loose patterns were shown in the micro-fibrils of BC membranes compared to that produced in HS medium. As the fermentation went on (Day 10), the BC network became denser and the space among the network became narrow ((d)). It indicated that more cellulose fibers were synthesized and crossed to create more layers in the inner matrix. The cellulose fibers obtained in HS medium became thicker (>0.1 μm) and randomly crossed to make the surface smoother and more compact without obvious pores and layers ((b)). Obviously, the structure with a denser network and thicker fibers can make the BC sheet have more strength. The different micro-morphologies of BC produced in fermentation wastewater and HS medium might impact the mechanical properties and water-holding capacity, which will be discussed later.
Figure 3. SEM micrographs of BC samples obtained in HS medium (a,b) and in polysaccharide fermentation wastewater (c,d) after 4 days (a,c) or 10 days (b,d) of fermentation.
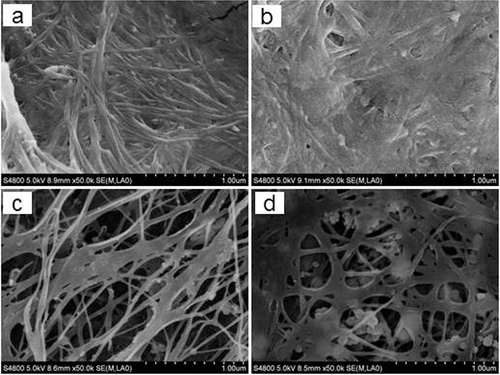
The functional groups of BC products obtained in the two different culture media were measured by FT-IR. As shown in (a–d), no significant differences were found in the functional groups of BC products during the fermentation in HS medium and wastewater. In the wavenumbers of 4000–400 cm−1 of the FT-IR spectra of the BC samples, the characteristic bands of cellulose (type I) synthesized in the two different media nearly appeared at the same wavenumbers of the FT-IR spectra, including 3406 cm−1 for the stretching vibration of hydroxyl groups (–OH), 2949 cm−1 for the asymmetric stretching vibration of methylene (–CH2–), 2849 cm−1 for the symmetric stretching vibration of methyl (–CH3–), 1432 cm−1 for the asymmetric deformation vibration of methyl and methylene and 1089 cm−1 and 915 cm−1 for the stretching vibration of C–O–C in the sugar ring, respectively [Citation18]. This indicated that the formula of the culture medium shows little influence on the functional groups of BC products.
Figure 4. FT-IR spectra of BC samples obtained in HS medium (a,b) and in polysaccharide fermentation wastewater (c,d) after 4 days (a,c) or 10 days (b,d) of fermentation.
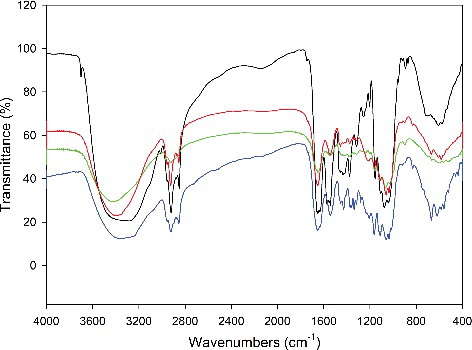
The XRD patterns obtained from the BC samples are shown in . The characteristic peaks that appeared at 14.5°, 16.9° and 22.9° stood for the crystal plane (1–10), (110) and (200), respectively [Citation19]. They were totally observed in all the BC samples tested, illustrating that the cellulose exhibited primarily the I-β pattern, regardless of the type of culture medium employed. However, the peak intensities of BC obtained in fermentation wastewater at 14.5°, 16.9° and 22.9° were much less than those in HS medium, indicating that the BC synthesized using wastewater as culture medium had much more amorphous component and less crystal component.
Figure 5. XRD patterns of BC samples obtained in HS medium (a,b) and in polysaccharide fermentation wastewater (c,d) after 4 days (a,c) or 10 days (b,d) of fermentation.
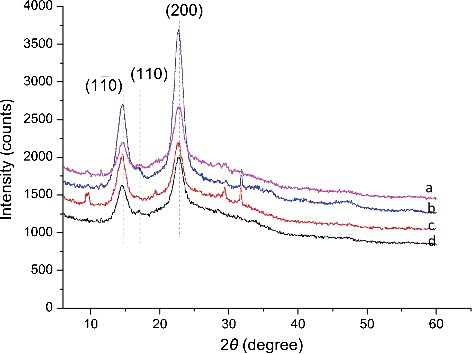
Crystallinity was calculated based on the Segal method [Citation9] by using the peak intensities of the (200) peak (I002) and the height of the minimum (Iam) between the (200) and the (110) peaks. As shown in , the crystallinity of BC samples obtained in fermentation wastewater was 50.66% and 56.23% at Day 4 and Day 10 respectively, which were lower than that obtained in HS medium (53.87% and 72.10% at Day 4 and Day 10).
Table 1. Crystallinity and crystal size of BC samples produced in HS medium and fermentation wastewater.
The crystallinity results were in well in accordance with the XRD patterns of the different BC samples. The BC samples produced in fermentation wastewater had less crystal component ((c,d)). BC materials with lower crystallinity obtained in fermentation wastewater have greater water-holding capacity but less strength than those obtained in HS medium, usually used as a new medical material in surgical dressing, artificial skin and artificial blood vessels [Citation20,Citation21]. The crystal size on crystal planes (1ī0), (110) and (200) of BC samples were also calculated (). It showed that a clear time-dependent trend appeared on the increase of the crystal size of the cellulose obtained in HS medium, especially on the (110) plane (it increased from 134.75 to 171.93 Å). However, the crystal size on the (1ī0) and (200) planes of BC samples produced in fermentation wastewater decreased significantly with a time-dependent pattern (p < 0.05). The different changes in the crystallinity and crystal size of the BC samples produced in HS medium and wastewater, not like the functional groups, revealed that the nutrient compositions of the culture medium might affect the cellulose micro-structure. It has been proved that different additives such as agar, carboxymethylcellulose (CMC) and sodium alginate added into the fermentation medium could change the productivity, crystallinity and crystal size of cellulose [Citation19]. In our study, BC samples with different micro-structure were also obtained in two different culture media. Further study should focus on the optimization of the nutrients composition according to the utilization of the BC products.
Mechanical properties of BC produced in wastewater and HS medium
The mechanical properties (including the Young's modulus, tensile strength and strain at break) of the BC samples obtained in our study were also evaluated. As shown in , the BC samples obtained from HS medium showed a significant increase in Young's modulus, tensile strength and strain at break compared to BC formed in wastewater (p < 0.05). For example, at Day 4, the Young's modulus of BC produced in HS medium was 6165.73 MPa, much higher than that obtained from wastewater (1469.43 MPa). The tensile strength increased from 64.84 to 107.67 MPa and the strain at break increased from 1.85% to 3.40%, respectively. Also a time-dependent increase in the Young's modulus, tensile strength and strain at break were observed both in HS medium and in wastewater. This indicated that extending the fermentation time might increase the polymerization degree of the cellulose, by which the mechanical properties of BC could be improved [Citation16].
Table 2. Mechanical properties of bacterial cellulose sheets produced in different medium.
Conclusions
In this study, it was demonstrated that pullulan polysaccharide fermentation wastewater has great potential to be used as a suitable low-cost substrate for BC production. Moreover, during the fermentation, the COD of the wastewater decreased, meaning that reuse of fermentation wastewater for the production of high-value added materials could be an economic approach to minimize the pollution caused by the industrial fermentation wastewater. The microstructure and mechanical properties of BC produced from wastewater were different from those obtained from the conventionally used chemical medium (HS medium). More pores and clear three-dimensional network structures were found in the BC sheet produced from fermentation wastewater. However, the mechanical strength of the BC pellets synthesized by wastewater should be modified in further studies, including strain mutations, fermentation conditions optimization, medium components and additives.
Acknowledgments
The authors would like to thank the public laboratory in Qingdao Institute of Bioenergy and Bioprocess Technology for the services of the SEM, FT-IR and XRD analysis.
Disclosure statement
No potential conflict of interest was reported by the authors.
Additional information
Funding
References
- Ross P, Mayer R, Benziman M. Cellulose biosynthesis and function in bacteria. Microbiol Rev. 1991;55:35–58.
- de Melo CV, Souza-Moreira T, Valentini SR, et al. Cloning of upstream region and cellulose synthase operon genes involved in bacterial cellulose biosynthesis by Gluconacetobacter hansenii ATCC23769. BMC Proc. 2014 [cited 2017 May 04];8:1. DOI:10.1186/1753-6561-8-S4-P176
- Klemm D, Schumann D, Udhardt U, et al. Bacterial synthesized cellulose-artificial blood vessels for microsurgery. Prog Polym Sci. 2001;26:1561–1603.
- Ul-Islam M, Khan T, Park JK. Water holding and release properties of bacterial cellulose obtained by in situ and ex situ modification. Carbohydr Polym. 2012;88:596–603.
- Aramwit P, Bang N. The characteristics of bacterial nanocellulose gel releasing silk sericin for facial treatment. BMC Biotechnol. 2014 [cited 2017 May 04];14:1. DOI:10.1186/s12896-014-0104-x
- Shah N, Ul-Islam M, Khattak WA, et al. Overview of bacterial cellulose composites: a multipurpose advanced material. Carbohydr Polym. 2013;98:1585–1598.
- Cavka A, Guo X, Tang S-J, et al. Production of bacterial cellulose and enzyme from waste fiber sludge. Biotechnol Biofuels. 2013 [cited 2017 May 04];6:1. DOI:10.1186/1754-6834-6-25
- Wei C, Zhang T, Feng C, et al. Treatment of food processing wastewater in a full-scale jet biogas internal loop anaerobic fluidized bed reactor. Biodegrad. 2011;22:347–357.
- Segal L, Creely J, Martin A, et al. An empirical method for estimating the degree of crystallinity of native cellulose using the X-ray diffractometer. Text Res J. 1959;29:786–794.
- Popescu MC, Popescu CM, Lisa G, et al. Evaluation of morphological and chemical aspects of different wood species by spectroscopy and thermal methods. J Mol Struct. 2011;988:65–72.
- Hong F, Qiu K. An alternative carbon source from konjac powder for enhancing production of bacterial cellulose in static cultures by a model strain Acetobacter aceti subsp. xylinus ATCC 23770. Carbohydr Polym. 2008;72:545–549.
- Gomes FP, Silva NH, Trovatti E, et al. Production of bacterial cellulose by Gluconacetobacter sacchari using dry olive mill residue. Biomass Bioenergy. 2013;55:205–211.
- Liu Z, Slininger P, Dien B, et al. Adaptive response of yeasts to furfural and 5-hydroxymethylfurfural and new chemical evidence for HMF conversion to 2, 5-bis-hydroxymethylfuran. J Ind Microbiol Biotechnol. 2004;31:345–352.
- Aktas ES, Imre S, Ersoy L. Characterization and lime treatment of olive mill wastewater. Water Res. 2001;35:2336–2340.
- Huang C, Guo H-J, Xiong L, et al. Using wastewater after lipid fermentation as substrate for bacterial cellulose production by Gluconacetobacter xylinus. Carbohydr Polym. 2016;136:198–202.
- Lin D, Lopez-Sanchez P, Li R, et al. Production of bacterial cellulose by Gluconacetobacter hansenii CGMCC 3917 using only waste beer yeast as nutrient source. Bioresour Technol. 2014;151:113–119.
- Ciechańska D. Multifunctional bacterial cellulose/chitosan composite materials for medical applications. Fibres Text East Eur. 2004 [cited 2017 May 04];12(4):69–72.
- Park JK, Jung JY, Park YH. Cellulose production by Gluconacetobacter hansenii in a medium containing ethanol. Biotechnol Lett. 2003;25:2055–2059.
- Cheng KC, Catchmark JM, Demirci A. Effect of different additives on bacterial cellulose production by Acetobacter xylinum and analysis of material property. Cellulose. 2009;16:1033–1045.
- Watanabe K, Tabuchi M, Morinaga Y, et al. Structural features and properties of bacterial cellulose produced in agitated culture. Cellulose. 1998;5:187–200.
- Fu L, Zhang J, Yang G. Present status and applications of bacterial cellulose-based materials for skin tissue repair. Carbohydr Polym. 2013;92:1432–1442.