ABSTRACT
The present study aimed to describe the cDNA cloning of the cinnamate 4-hydroxylase (C4H) gene from ramie, predict the protein sequence and perform phylogenetic and structural analyses of this gene. C4H catalyses the hydroxylation of trans-cinnamic acid to p-coumaric acid, which plays a crucial role in lignin biosynthesis. In the present study, the gene encoding C4H was cloned by polymerase chain reaction (PCR) technology from cultivated ramie (Boehmeria nivea) and named BnGC4H. In addition, bioinformatics and tissue expression analyses were performed. The results showed that the cloned BnGC4H cDNA contained a 1518-bp open reading frame encoding a 505–amino acid protein. The sequence of BnGC4H is available from the GenBank database with accession number KY937946. The amino acid sequence and structural analysis revealed that BnGC4H shared conserved domains with other C4H forms, including cytochrome P450 domain and trans-cinnamate 4-monooxygenase domain. The phylogenetic analysis indicated that BnGC4H was closely related to Aquilaria sinensis C4H and Ruta graveolens C4H. Real-time quantitative reverse transcription–PCR and RNA in situ hybridization showed that BnGC4H was strongly expressed in the xylem during the maturity stage. The findings provided a theoretical basis for further exploring the function of BnGC4H in the lignin biosynthesis and regulation in ramie.
Introduction
Cinnamate 4-hydroxylase (C4H, EC 1.14.13.11) is a key enzyme in the phenylpropanoid pathway [Citation1]. The role of C4H is to catalyse the hydroxylation of trans-cinnamic acid to p-coumaric acid [Citation2]. Monooxygenase reactions are important in the biosynthesis of diverse metabolites, including fatty acids, phenylpropanoids, alkaloids and terpenoids, in plants. They are mediated by the CYP73 family and the phenylpropanoid pathway leading to monolignol biosynthesis [Citation3]. C4H also participates in these reactions as a member of CYP73 family [Citation4]. C4H is the first monooxygenase in the phenylpropanoid pathway identified from Arabidopsis thaliana [Citation1]. C4H, phenylalanine ammonialyase (PAL), 4-coumarate: CoA ligase (4CL) and chalcone synthase (CHS) also play a key role in the regulation of lignin biosynthesis [Citation5]. The sequences and expression patterns of C4H have been reported in several plants such as poplar (Populus trichocarpa × P. deltoides) [Citation6], tea (Camellia sinensis) [Citation7], oilseed rape (Brassica napus) [Citation8], garlic (Allium sativum) [Citation9], Scutellaria baicalensis [Citation10], Ornithogalum saundersiae [Citation11] and Angelica gigas [Citation12]. These studies provided basic information of C4H as an enzyme in synthetic pathways in plants.
Ramie (Boehmeria nivea) is a widely grown herbaceous plant in southern China that provides natural fibre. The phloem and xylem in ramie are involved in nutrition and water transport and utilization. The phloem can be used for generating bast fibres, whereas lignin from the xylem can be used for industrial ethanol production or fermentation to produce biofertilizers [Citation13,Citation14]. Thus, understanding the developmental mechanisms of these two different tissues in ramie is important to enhance the ramie fibre industry. In addition, C4H as a key gene in anabolism and represents an important step in characterizing the biosynthetic pathway. For instance, the overexpression of C4H prompts flavone accumulation in S. baicalensis hairy roots, while the downregulation of C4H leads to improved sugar release [Citation10]. Moreover, C4H is involved in the lignin biosynthetic pathway, and the downregulation of C4H reduces the overall lignin content by affecting the ratio of syringyl (S) to guaiacyl (G) [Citation15]. Hence, several studies have focused on the characterization of C4H in different plants. However, the information about the characterization of C4H in ramie is scarce so far.
The formation of phloem and xylem in ramie is a major point of interest. The present study aimed to describe the cDNA cloning of C4H from ramie, predict the protein sequence and perform phylogenetic and structural analyses of this gene. Real-time quantitative reverse transcription–polymerase chain reaction (qRT-PCR) was used to analyse the expression profile of C4H mRNA in ramie from phloem and xylem during different developmental stages (young, mature and late mature). Furthermore, in situ hybridization was performed to identify the tissue distribution of C4H transcripts in the stems of ramie during these stages. The present findings would aid in understanding the role of C4H in ramie.
Material and methods
RNA isolation and cDNA synthesis
Ramie was planted in the College of Biological and Environmental Engineering, Changsha University, China. The total RNA was isolated from the stem of ramie using the SV Total RNA Isolation System (Promega, WI, USA), according to the manufacturer's instructions. The RNA integrity was detected by agarose gel electrophoresis and the RNA concentration was determined using an Eppendorf BioPhotometer UV/VIS Spectrophotometer (Hamburg, Germany). A reverse transcription kit (Invitrogen, MD, USA) was used to generate the first strand cDNA from 1 μg of total RNA following the manufacturer's protocol.
cDNA cloning of C4H in ramie
The Primer Premier 5.0 software (Premier Biosoft International, CA, USA) was used to design the primer according to the previous transcriptome data as follows. C4H: forward primer, 5’-CACCAATACAAAGCCACA-3’; reverse primer, 5’-AACAATCACCCACAATCC-3’. The PCR system comprised 16.2 µL of sterile water, 2.5 µL of 10 × PCR buffer, 2.5 µL of 2.5 mmol/L deoxynucleotide triphosphate mix, 1 µL of cDNA, 1.3 µL of sense primer, 1.3 µL of antisense primer and 0.2 µL of Takara Ex Taq Hot Start (TaKaRa, Tokyo, Japan). The PCR reaction was performed in CFX96 Real-Time System (Bio-Rad, CA, USA) as follows: 94 °C for 5 min, 30 cycles at 94 °C for 30 s, 57 °C for 30 s, 72 °C for 2 min, followed by 72 °C for 10 min. The PCR products were purified using a gel extraction kit (Omega Bio-Tek, GA, USA), followed by cloning into a pMD18-T vector (TaKaRa) and then sequencing (Sangon, Shanghai, China).
Bioinformatics analysis
The Open Reading Frame (ORF) Finder (https://www.ncbi.nlm.nih.gov/orffinder/) was used to search for the ORF of C4H. The Basic Local Alignment Search Tool (BLAST) software from the National Center for Biotechnology Information (NCBI, http://www.ncbi.nlm.nih.gov/) was used to identify the conserved domains and analyse the relevant protein sequence. The program Mega 4.1 was used to draw the phylogenetic tree of the orthologs among different plants. The program NetPhos 2.0 [Citation16] was used to identify phosphorylation sites.
RT-qPCR
The stem of ramie was collected during different developmental stages (young: 10 days after seedling; mature: 40 days after seedling; late mature: 60 days after seedling) for RT-qPCR analysis. After preparing the total RNA, cDNA was prepared with PrimeScript RT Reagent Kit and gDNA Eraser (TaKaRa) using 1 μg of total RNA. The primers were designed based on C4H from previous transcriptome data as follows. Real-time C4H: forward primer, 5’-CATTCCTGCGAGGCTACTTG-3’; reverse primer, 5’-CTGCTGAGCGTCAAGAATGTG-3’; real-time Actin1: forward primer, 5’-AGTGGAGAAGCGTGGTTT-3’; reverse primer, 5’-ATGAATGACGATAATGGC-3’. The RT-qPCR was performed for every run in four technical replicates. Each reaction involved a 12.5-μL system comprising 6.25 μL of 2 × SYBR Premix Ex Taq (TaKaRa), 1 μL of cDNA, 0.5 µL of sense primer (10 μmol/L), 0.5 µL of antisense primer (10 μmol/L) and 4.25 μL of sterile water. The reaction was performed on the Stratagene Mx3005 System (Stratagene, CA, USA) as follows: 95 °C for 30 s, 40 cycles at 95 °C for 5 s, and 55 °C for 30 s. The relative expression was calculated using the 2−ΔΔCt method. Ramie Actin1 was used as the internal control.
RNA in situ hybridization
In situ hybridization was used to analyze the tissue distribution of C4H in the stem of ramie collected during different developmental stages. The tissues were fixed in 4% paraformaldehyde (Sangon) for 2 h at room temperature and 24 h at 4 °C, embedded in paraffin, and sliced into 4-µm slices. After baking overnight at 45 °C, the paraffin was dewaxed using xylene and hybridized with the probe at 42 °C overnight. The sequence of the probe was as follows: 5’-GGATGAAATCGCCGTAGTTGTACTCGAAGCTCTGCGCCAAGCGGCTACGCTCTCCGTTCAAGGCCCTGAGCTTGACGAAGAGCGGGTCTTCCTGGCTCTCGAAGCGCCGGTCGAACATGATCCTGTACATGTTGTTGTACATCAACAGCTGGAGCCTGCGGCGGAGGACGACGCCGTCCCGCGCGGCGTCGGGGTGCGCGCGCATGTCGTCGACGACGGCGGCGGCCTCGGCCTCCCAGCCGCCGCGGTACTGCTGGACGACCTTGTTGGTGAAGAACGG-3’. Following rinsing with saline sodium citrate and incubation in blocking solution, the slices were processed with mouse anti-digoxin-biotin antibody, peroxidase-conjugated streptavidin–biotin complex and streptavidin–horseradish peroxidase (Bost Bio-engineering, Wuhan, China) at 37 °C. Finally, the slices were stained using diaminobenzidine (DAB) and counterstained with hematoxylin (Bost Bio-engineering).
Statistical analysis
SPSS 17.0 (SPSS, Inc., IL, USA) was used for statistical analysis. The differential expression of C4H among different groups or periods was determined using the one-way analysis of variance and Tukey's multiple comparison test. A P value ≤0.05 was considered to indicate statistically significant differences.
Results and discussion
Ramie C4H cDNA sequence amplification and electrophoresis
RT-PCR was used to obtain a 1626-bp sequence of the ramie C4H gene. This PCR product was cloned into the pMD18-T vector and sequenced. The ramie C4H gene was used as BnGC4H. This sequence was deposited into GenBank and assigned the accession number KY937946. The nucleotide sequence analysis showed that it contained a 1518-bp ORF encoding a 505–amino acid peptide (). The cDNA sequence of C4H also contained an 18-bp 5’untranslated region (UTR) and a 90-bp 3’UTR.
The phenylpropanoid pathway is a biosynthetic pathway to generate hydroxycinnamic acid and other derivatives including multiple secondary substances of polyphenols that have important physiological functions such as lignin and flavonoid formation [Citation17]. Lignin is the main component of the plant cell wall that participates in the growth of roots and stems in plants with cellulose [Citation15]. The other secondary substances such as flavanones, flavonols, anthocyanins, proanthocyanidins (pigments in the seed coat), isoflavones and other flavonoid substances play an important role in plant growth, disease resistance and stress tolerance [Citation18]. Hence, C4H identified in different plants would play a key role in promoting economic crop production in the future. Although C4H has been identified and characterized in several plants, its presence in ramie has not been reported yet. This study identified C4H from ramie. The full-length ORF of C4H was obtained in ramie using gene cloning, and the gene was named BnGC4H. The BnGC4H protein was predicted to comprised 505 amino acids, similar to that in other plants, indicating high conservation among the plants.
Amino acid sequence analysis of the BnGC4H protein
The conserved domain was identified by NCBI BLAST analysis. The predicted BnGC4H protein contained two conserved domains including pfam00067 (cytochrome P450) and PLN02394 (trans-cinnamate 4-monooxygenase) ((A)). The residues of the catalytic sites of BnGC4H included I109, K113, V118, F220, E301, N302, I303, V305, A306, T310, R366, R368, A370, I371, P372, L374, V375, P376, H377, K484, F488 and L490. These catalytic sites were distributed in five special substrate-binding domains, including the SRSl (SRTRNVVFDIFTGKGQDMVFTVY), SRS2 (LAQSFEYNY), SRS4 (IVENINVAAIETTLWS), SRS5 (RMAIPLLVPH) and SRS6 (KGGOFSLHI). The NetPhos 2.0 prediction was used to identify 15 phosphorylation sites in the BnGC4H protein, including 8 serine, 3 threonine and 4 tyrosine residues ((B,C)).
Figure 2. Analysis of protein sequence of BnGC4H. (A) A conserved domain in BnGC4H of ramie. (B) Fifteen phosphorylation sites identified by NetPhos 2.0 prediction. (C) The sequence positions of predicted phosphorylation sites by NetPhos 2.0.
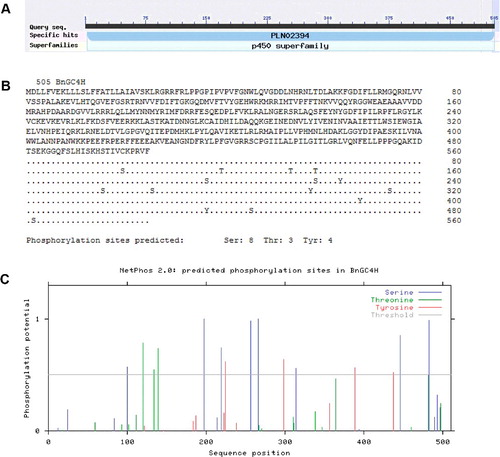
The protein domains were also found to be relatively conservative using the BLAST analysis. The cytochrome P450 and trans-cinnamate 4-monooxygenase domains were observed in the BnGC4H protein. This enzyme comprised a cytochrome P450 domain essential for the P450-containing system [Citation19]. The system served as a terminal oxidase in multicomponent electron transfer chains and was named the P450-containing monooxygenase system [Citation20]. This domain was a signature domain in C4H. Another signature domain of C4H is trans-cinnamate 4-monooxygenase [Citation21], also reported in the present study. These two conserved domains indicated that the gene cloned in the present study was C4H. Five special substrate-binding domains were identified in the BnGC4H protein, indicating the functional region of this protein. SRS5, SRS6 and SRS4 substrate-binding domains contained the aromatic rings that serve as the binding site for the substrate at the C-terminus [Citation22]. The N-termini of SRS4, SRS1 and SRS2 are key areas for binding the aliphatic chain of the substrate [Citation22]. These domains showed that BnGC4H is a member of the C4H/CYP73A5 family with catalytic function. Meanwhile, 15 phosphorylation sites were observed in the BnGC4H protein. These sites were predicted to play a key role in the catalytic activity.
Phylogenetic analysis of C4H in plants
The similarities of the C4H proteins were analyzed by multiple comparisons with different species of plants ((A)). BnGC4H had high similarity among all the plants (The similarity was higher than 90%). Based on the similarity analysis, 22 sequences were used for phylogenetic analysis ((B)). The C4H proteins in Aquilaria sinensis (C4H), Ruta graveolens (C4H) and ramie (C4H) were closely related.
Figure 3. Phylogenetic analysis of BnGC4H among different plants. (A) Coding region amino acid sequence alignment of BnGC4H in ramie and homologous genes. The sequences of Nelumbo nucifera (accession no. XP_010253 046.1), Gossypium raimondii (accession no. XP_012454 096.1) and Gossypium hirsutum (accession no. NP_001314 057.1) were downloaded from the NCBI GenBank database. (B) Phylogenetic tree of C4H from ramie and other plant species. The sequences of Nicotiana tabacum (C4H1) ABC69412; Nicotiana tabacum (C4H2) ABC69411; Petunia hybrida (C4H1) ADX33332; Petunia hybrida (C4H2) ADX33333; Arabidopsis thaliana (C4H) AAC99993; Brassica napus (C4H1) DQ485129; Brassica napus (C4H2) DQ485131; Gossypium arboreum (C4H1) AAG10197; Gossypium arboreum (C4H2) AAG10196; Gossypium hirsutum (C4H1) FJ848866; Gossypium hirsutum (C4H2) FJ848867; Epimedium sagittatum (C4H) AIS92509.1; Ruta graveolens (C4H) AAN63028.1; Aquilaria sinensis (C4H) AGP25594.1; Boehmeria nivea (C4H) KY937946; Miscanthus giganteus (C4H1) ANB43563.1; Miscanthus giganteus (C4H2) ANB43564.1; Panicum virgatum (C4H1) AFY17064.1; Panicum virgatum (C4H2) AFY17065.1; Sorghum bicolor (C4H) AAK54447.1; Zea mays (C4H) NP001151365.1; Oryza sativa Japonica Group (C4H) BAF45113.1 were downloaded from the NCBI GenBank database.
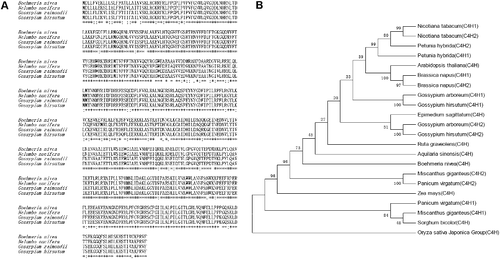
The high similarity of C4H proteins found among the analysed plants (over 90%) indicated that the protein is conserved. This result also demonstrated that the present cloned C4H was a BnGC4H gene. The phylogenetic tree showed a close relationship with A. sinensis (C4H) and R. graveolens (C4H), showing the evolutionary relationship among these plants. Since the structures of C4H proteins had a high similarity among these plants, it was presumed that the functions of these proteins were concordant. Furthermore, the spatiotemporal expression pattern of BnGC4H in ramie was characterized.
Expression analysis of the BnGC4H gene during different developmental stages in ramie
The changes in the expression of BnGC4H during different developmental stages in the xylem and phloem of ramie were determined by RT-qPCR. All the tested tissues expressed BnGC4H, indicating a widespread expression of this gene. The lowest expression level of BnGC4H was found in the phloem during the initial period, while the highest expression level was found in the xylem during the maturity stage. The expression levels at different stages were significantly higher in the xylem than in the phloem. Also, the highest expression level was found during the maturity stage in both the xylem and the phloem ().
Figure 4. Relative expression levels of BnGC4H during different developmental stages in the xylem and phloem of ramie. *P < 0.05.
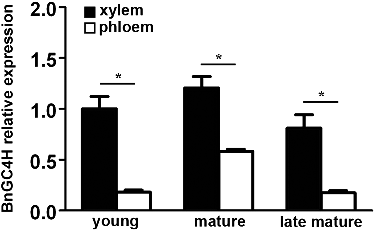
This result indicated that BnGC4H was highly expressed in the xylem, similar to previous studies. In poplar, C4H is reportedly localized in differentiating xylem on the rough endoplasmic reticulum and Golgi apparatus [Citation23]. C4H is necessary for lignin biosynthesis and vessel formation [Citation24]. C4H is also highly expressed in the apical bud of tea, indicating an inducing effect on tissue growth [Citation7]. C4H is also correlated with high catechin content [Citation7]. The high expression of C4H was also found in the xylem. A comparison of different developmental stages showed that the highest expression of C4H was found during the maturity stage. C4H is highly expressed during the early stage of development in kenaf (Hibiscus cannabinus) [Citation25]. Moreover, the expression of C4H has been reported to be the highest in the mature flower [Citation25]. Also, the higher expression of C4H in mature leaves is reported in Cannabis sativa [Citation26]. This evidence suggests that the expression of C4H is most likely in accordance with the lignin growth. Thus, the higher expression of BnGC4H in mature xylem is understandable.
Tissue distribution of BnGC4H during different stages in ramie detected using in situ hybridization
After confirming the higher expression of BnGC4H in the xylem, the stems from different stages were selected for in situ hybridization to determine the spatial expression patterns of BnGC4H. Digoxin-labelled RNA probes specific to BnGC4H mRNA were used. The result showed that the signals of BnGC4H were more abundant in the xylem compared with those in the phloem. Meanwhile, the signals were more abundant during the maturity stage, consistent with the RT-qPCR result. The present study also showed high expression during the late maturity stage using in situ hybridization ().
Figure 5. In situ hybridization (ISH) of BnGC4H transcripts during different developmental stages of the ramie stem. Transverse sections hybridized with antisense probes at young stage (A and B), maturity stage (C and D) and late maturity stage (E and F).
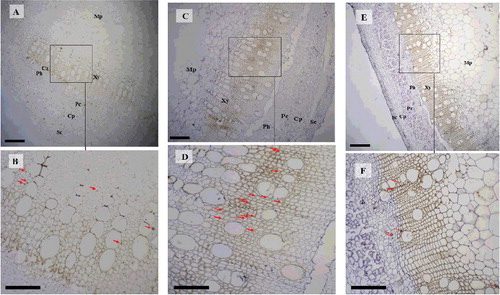
As we found, BnGC4H was highly expressed in mature xylem from the stem, similar to the RT-qPCR result. However, BnGC4H expression was also found during the late maturity stage, inconsistent with the RT-qPCR result. This might be due to differences in the detection methods. In situ hybridization is a more suitable approach for gene distribution analysis than for detecting expression levels. Consequently, the high expression of BnGC4H in the mature xylem could be confirmed.
Conclusions
C4H cDNA from ramie was isolated in this study, and the gene was named BnGC4H. Subsequently, the conserved domains were identified, suggesting a similar function of this gene among the plants. The phylogenetic analysis showed that BnGC4H was highly conservative among plants. The spatiotemporal expression pattern during different developmental stages in the xylem and phloem of ramie was evaluated using RT-qPCR and in situ hybridization. High expression of BnGC4H was found in the mature xylem. These pieces of evidence suggested that BnGC4H plays an important role in xylem development in ramie.
Disclosure statement
No potential conflict of interest was reported by the authors.
Additional information
Funding
References
- Li W, Yang L, Jiang L, et al. Molecular cloning and functional characterization of a cinnamate 4-hydroxylase-encoding gene from Camptotheca acuminata. Acta Physiol Plant. 2016 [cited 2017 Apr 14];38(11):256. DOI:10.1007/s11738-016-2275-7
- Kumar R, Vashisth D, Misra A, et al. RNAi down-regulation of cinnamate-4-hydroxylase increases artemisinin biosynthesis in Artemisia annua. Sci Rep. 2016 [cited 2017 Apr 14];6:26458. DOI:10.1038/srep26458
- Umemoto N, Nakayasu M, Ohyama K, et al. Two cytochrome P450 monooxygenases catalyze early hydroxylation steps in the potato steroid glycoalkaloid biosynthetic pathway. Plant Physiol. 2016;171(4):2458–2467.
- Du H, Ran F, Dong H L, et al. Genome-wide analysis, classification, evolution, and expression analysis of the cytochrome P450 93 family in land plants. PloS One. 2016 [cited 2017 Apr 14];11(10):e0165020. DOI:10.1371/journal.pone.0165020
- Li G, Zhu S, Wu W, et al. Exogenous nitric oxide induces disease resistance against Monilinia fructicola through activating the phenylpropanoid pathway in peach fruit. J Sci Food Agric. 2017;97(9):3030–3038.
- Ro DK, Mah N, Ellis BE, et al. Functional characterization and subcellular localization of poplar (Populus trichocarpa× Populusdeltoides) cinnamate 4-hydroxylase. Plant Physiol. 2001;126(1):317–329.
- Singh K, Kumar S, Rani A, et al. Phenylalanine ammonia-lyase (PAL) and cinnamate 4-hydroxylase (C4H) and catechins (flavan-3-ols) accumulation in tea. Funct Integr Genomics. 2009;9(1):125–134.
- Chen AH, Chai YR, Li JN, et al. Molecular cloning of two genes encoding cinnamate 4-hydroxylase (C4H) from oilseed rape (Brassica napus). BMB Rep. 2007;40(2):247–260.
- Tuan PA, Park NI, Li X, et al. Molecular cloning and characterization of phenylalanine ammonia-lyase and cinnamate 4-hydroxylase in the phenylpropanoid biosynthesis pathway in garlic (Allium sativum). J Agric Food Chem. 2010;58(20):10911–10917.
- Xu H, Park NI, Li X, et al. Molecular cloning and characterization of phenylalanine ammonia-lyase, cinnamate 4-hydroxylase and genes involved in flavone biosynthesis in Scutellaria baicalensis. Bioresource Technol. 2010;101(24):9715–9722.
- Kong JQ, Lu D, Wang ZB. Molecular cloning and yeast expression of cinnamate 4-hydroxylase from Ornithogalum saundersiae baker. Molecules. 2014;19(2):1608–1621.
- Park JH, Park NI, Xu H, et al. Cloning and characterization of phenylalanine ammonia-lyase and cinnamate 4-hydroxylase and pyranocoumarin biosynthesis in Angelica gigas. J Nat Prod. 2014;73(8):1394–1397.
- Kandimalla R, Kalita S, Choudhury B, et al. Fiber from ramie plant (Boehmeria nivea): a novel suture biomaterial. Mat Sci Eng C. 2016;62:816–822.
- Kipriotis E, Heping X, Vafeiadakis T, et al. Ramie and kenaf as feed crops. Ind Crops Prod. 2015;68:126–130.
- Mottiar Y, Vanholme R, Boerjan W, et al. Designer lignins: harnessing the plasticity of lignification. Curr Opin Biotechnol. 2016;37:190–200.
- Blom N, Gammeltoft S, Brunak S. Sequence and structure-based prediction of eukaryotic protein phosphorylation sites. J Mol Biol. 1999;294(5):1351–1362.
- Xiong D, Lu S, Wu J, et al. Improving key enzyme activity in phenylpropanoid pathway with a designed biosensor. Metab Eng. 2017;40:115–123.
- Ferreyra MLF, Rius SP, Casati P. Flavonoids: biosynthesis, biological functions, and biotechnological applications. Front Plant Sci. 2012 [cited 2017 Apr 14];3:222. DOI:10.3389/fpls.2012.00222
- Liu Z, Tavares R, Forsythe ES, et al. Evolutionary interplay between sister cytochrome P450 genes shapes plasticity in plant metabolism. Nat Commun. 2016 [cited 2017 Apr 14];7:13026. DOI:10.1038/ncomms13026
- Gordeziani MS, Varazi TG, Pruidze MV. Structural–functional organization of cytochrome P450 containing monooxygenase and some aspects of modeling. Ann Agrar Sci. 2016;14(2):82–94.
- Schomburg D, Stephan D. Trans-cinnamate 4-monooxygenase. In: Enzyme handbook. New York: (NY): Springer; 1994. p. 441–444.
- Rupasinghe S, Baudry J, Schuler MA. Common active site architecture and binding strategy of four phenylpropanoid P450s from Arabidopsis thaliana as revealed by molecular modeling. Protein Eng. 2003;16(10):721–731.
- Sato T, Takabe K, Fujita M. Immunolocalization of phenylalanine ammonia-lyase and cinnamate-4-hydroxylase in differentiating xylem of poplar. C R Biol. 2004;327(9–10):827–836.
- Zhao Q. Lignification: flexibility, biosynthesis and regulation. Trends Plant Sci. 2016;21(8):713–721.
- Kim J, Choi B, Natarajan S, et al. Expression analysis of kenaf cinnamate 4-hydroxylase (C4H) ortholog during developmental and stress responses. Plant Omics. 2013;6(1):65–72.
- Docimo T, Consonni R, Coraggio I, et al. Early phenylpropanoid biosynthetic steps in Cannabis sativa: link between genes and metabolites. Int J Mol Sci. 2013;14(7):13626–13644.