ABSTRACT
Nanobodies (Nbs) are variable domains of the heavy chain of the heavy-chain antibodies (VHHs) found only in camelids and sharks. They have the same binding capability as full-length immunoglobulin G (IgG) but exhibit improved thermal stability and permeability, pointing to potential scientific, medical, and industrial applications. The aim of the present study was to identify an alternative method for more efficient production of Nbs in the Escherichia coli-based expression system. Several fusion tags, including maltose-binding protein (MBP), small ubiquitin-related modifier (SUMO) and hexahistidine (6 × His), were examined to determine the optimal tag for the expression and purification of VHHs. In general, the SUMO fusion tag could improve the solubility, yield and antigen-binding activity of the VHHs, while the other two tags either decreased the antigen-binding activity (MBP) or decreased the yield (6 × His) of the VHHs. What is more, the SUMO tag was easily removed by SUMO protease 1 to release the native VHHs. These advantages indicated that SUMO fusion could be considered an alternative strategy to express VHHs in the cytoplasm of E. coli.
KEYWORDS:
Introduction
All antibodies (Abs) in mammalian species were believed to comprise two identical H-chains and two identical L-chains until 1989. A new type of Ab was discovered in the sera of dromedaries and then in all other species of the Camelidae family [Citation1], as well as in the nurse shark, wobbegong shark and ratfish [Citation2]. These Abs are devoid of light chains and the first constant domain, and so are called heavy-chain antibodies. The binding affinities and specificities of the variable domains of the heavy chain of camelid heavy-chain antibodies (VHHs) to their cognate antigens are similar to those of conventional Abs [Citation3,Citation4]. VHHs are named nanobodies (Nbs) because of the diameter of their crystal structure (ca. 2.5 nm), length (ca. 4 nm) and molecular size (ca. 15 kDa) [Citation5,Citation6]. Despite their small size, Nbs retain the same binding capability as full-length immunoglobulin G (IgGs). In comparison with conventional Abs, Nbs possess long complementarity determining regions, and they exhibit strict monomeric behaviour, high protein stability and solubility, reversible folding and resistance to proteolysis [Citation7–9]. The small size and long complementarity determining regions of Nbs enable them to recognize epitopes located in clefts and protein cavities [Citation10]. Given these advantages, Nbs have been widely applied in various fields, such as drug development, disease diagnostics, therapeutic applications and biotechnological applications [Citation11].
The characteristics of Nbs allow for efficient expression of bioactive molecules in different hosts, such as yeast, mammalian and bacterial cells. For any applicative purpose, large-scale production of recombinant proteins is essential. Escherichia coli remains the most suitable microorganism for the expression and purification of Nbs. Numerous strategies have been used to improve the solubility of recombinant proteins and reduce the formation of inactive inclusion bodies in E. coli-based expression systems. These include the development of strong promoters, co-expression with chaperones and use of protein fusion tags [Citation12–15]. Indeed, many described fusion tags have been developed, including glutathione S-transferase (GST), maltose-binding protein (MBP), thioredoxin, N-utilization substance A (NusA), ubiquitin (UB), hexahistidine (His6 tag) and small UB-related modifier (SUMO), to solve the problems of low expression, poor solubility, and misfolding in E. coli. Although Nbs are generally soluble and well expressed, Nbs fused to different fusion tags vary in terms of expression levels, protein solubility, yield and antigen-binding activity [Citation16,Citation17].
In the present study, three expression systems consisting of different fusion tags (MBP tag, SUMO tag and 6 × His tag) were used to express selected Nbs to determine the optimal tag for the expression and purification of VHHs in terms of solubility, yield and antigen-binding activity.
Materials and methods
Bacterial strains, vectors, enzymes and resins
The E. coli strains BL21 (DE3) (Novagen), TB1 (NEB) and Rosetta (Novagen) were used for protein production. The vectors pCold I DNA (Takara, Dalian, China), pMAL-c2X (NEB) and pE-SUMO (NEB) were used to express Nbs. SUMO protease 1 (Ulp1) was maintained in our laboratory. Amylose resin (NEB, USA) and TALON metal affinity resin (Clontech) were used for purification with the MBP and His tag fusion proteins, respectively. PCV2-positive serum and PCV2-negative serum were purchased from Wuhan Keqian Animal Biological Products Co., Ltd, China.
Construction of expression plasmids with fusion tags
All the plasmids were constructed by standard methods. The VHH sequences against the anti-Cap protein of porcine circovirus type 2 (PCV2) were selected from a yeast two-hybrid library [Citation18]. The protein structure of VHHS was calculated by SWISS-MODEL software (Swiss Institute of Bioinformatics, Lausanne, Switzerland). His-Nb and MBP-Nb fusions were constructed by modifying the vectors pCold I DNA and pMAL-c2X, which were digested by BamHI and SalI restriction enzymes. His-SUMO-Nb fusions were constructed by cloning Nbs into the pE-SUMO expression vector using BsaI and BamHI cloning sites. All recombinant plasmids were sequenced by GenScript (Nanjing, China).
Expression of recombinant fusion proteins in E. coli
The recombinant plasmids pCold-Nbs, pSUMO-Nbs and pMAL-c2X-Nbs were transformed into competent E. coli BL2 (DE3), Rosetta and TB1 cells, respectively. The pCold-Nb/BL21(DE3) culture was induced by 0.4 mmol/L IPTG (isopropyl β-D-1-thiogalactopyranoside) at 15 °C for 6 h. The pMAL-c2X-Nb/TB1 culture was induced by 0.3 mmol/L IPTG at 37 °C for 3 h. The pSUMO-Nb/Rosetta culture was induced by 0.4 mmol/L IPTG at 25 °C for 4 h. The bacterial cells were then harvested and sonicated, and the supernatant was subjected to purification and analyzed by 12% sodium dodecyl sulfate-polyacrylamide gel electrophoresis (SDS-PAGE).
Cleavage of SUMO fusion proteins and purification of Nb2
The native VHH was released from the fusion protein by cleavage with Ulp1. The cleavage reaction was prepared using the optimal buffer components, pH and ratio of Ulp1 to tagged protein. Purified His-SUMO-Nb2 was dialyzed in phosphate buffered saline (PBS) at 4 °C for 4 h and collected in a conical tube. The cleavage reaction was developed by adding 1 unit of SUMO protease per 100 µg of tagged protein. The digestion mixture was slowly shaken at 4 °C for 12–16 h and then removed for cleavage efficiency analysis by SDS-PAGE. The mixture was loaded onto a 5 mL column with 1 mL of TALON metal affinity resin (Clontech), equilibrated with binding buffer (50 mmol/L Tris, 500 mmol/L NaCl, pH 8.0). Consequently, the his-tagged SUMO bound to the resin and the native VHH left in the downstream liquid was subjected to SDS-PAGE to analyze the purity. The VHH solution was determined by measuring the protein concentration by the bicinchoninic acid assay.
Indirect enzyme-linked immunosorbent assay (ELISA)
The antigen-binding activity of the purified VHH fusions was analyzed by indirect ELISA. Breifly, the purified native Cap protein (produced by our laboratory, 10 µg/well) was coated onto Maxisorp (Nunc) 96-well plates and then blocked with 5% (w/v) skimmed milk-PBS with 0.05% Tween 20 (PBST) for 1 h at 37 °C. After three washes with PBST, the purified MBP-Nb (containing C-terminal hexahistidine), His-SUMO-Nb, His-Nb, PCV2-positive serum and PCV2-negative serum were diluted in the same buffer and loaded for 2 h at 37 °C. Subsequently, the plates were incubated with horseradish peroxidase (HRP)-conjugated anti-His tag mouse monoclonal antibody (1:2000 dilution; CWBIO, China) for 1 h at 37 °C. For PCV2-positive serum and PCV2-negative serum, the plates were incubated with HRP-conjugated goat anti-pig IgG (1:5000 dilution; abcam, ab6915) for 1 h at 37 °C. After five washes with PBST, all the reactions were developed by adding tetramethylbenzidine (TMB; TIANGEN, Beijing). The absorbance at 450 nm (OD450) was determined.
Double antibody sandwich ELISAs
To evaluate the antigen-binding activity of monomeric Nb2, the plates were coated with Nb2 (2 nmol/L), His-SUMO-Nb2 (2 nmol/L), PCV2-positive serum (positive control) and PCV2-negative serum (negative control). The plates were blocked with 5% (w/v) skimmed milk for 1 h at 37 °C. After three washes with PBST, the PCV2 Cap protein (10 µg/mL) was added into the wells and incubated for 1 h at 37 °C. Then MBP-Nb1 (50 nmol/L) was incubated at 37 °C for 1 h, followed by the addition of HRP-conjugated anti-MBP tag mouse monoclonal antibody (1:2000 dilution; abcam, ab49923). The plates were then incubated for 1 h at 37 °C until the reaction was developed by TMB as described above. The absorbance at 450 nm (OD450) was determined.
Statistical analysis
All experiments were performed with at least three independent experiments. All the data were expressed as mean values with standard deviation (±SD). One-way analysis of variance (ANOVA) was employed for comparison among the groups, with Tukey's comparison tests of significant differences among groups in SPSS 19.0 (SPSS, Inc., Chicago, IL, USA). A value of P < 0.05 was considered to indicate statistically significant differences.
Results and discussion
Heterologous production of VHHs is possible in bacteria, yeast and mammalian cells. However, such production has various limitations, including reduced or absent antigen-binding capabilities due to O-glycosylation by yeasts and glycosylation by mammalian cells, the high cost of scaling up production and the low soluble expression and formation of inactive inclusion bodies in E. coli [Citation19]. In recent years, it has become clear that affinity fusion tags can have a positive impact on the yield, solubility and even the folding of their fusion partners. However, no single affinity tag is optimal with respect to all of these parameters [Citation20].
Herein, we screened the abilities of three candidate fusion tags (MBP, 6 × His and SUMO) to express VHHs. The MBP-Nb, SUMO-Nb and His-Nb fusions were well expressed in the periplasm of E. coli cells and purified consistently in soluble form by sequential amylose or metal affinity chromatography. Based on comprehensive analysis of the solubility, yield and antigen-binding activity of the VHHs, these results demonstrate that SUMO tag fusion has a great potential to be an effective strategy for large-scale production of bioactive VHHs in the cytoplasm of E. coli.
Cloning, expression, and purification of the recombinant fusion VHHs
Two characterized VHH sequences were chosen from the Cap-specific VHH library. The three-dimensional (3D) structure modeling showed that the two VHH sequences were completely different, namely, Nb1 and Nb2 (). Three expression vectors (pCold-Nbs, pSUMO-Nbs and pMAL-c2X-Nbs) were constructed ((A–C)).
Figure 2. Construction of three Nb expression vectors: pCold-Nb (A), pSUMO-Nb (B) and pMAL-c2X-Nb (C) and PCR amplification (D) of the VHH fragments: M, DNA marker; Lane 1, Nb1 containing a BamHI restriction site; Lane 2, Nb2 containing a SalI restriction site; Lane 3, Nb1 containing a BsaI restriction site; Lane 4, Nb2 containing a BamHI restriction site; Lane C, negative control.
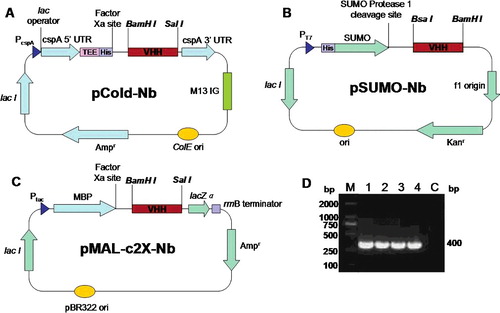
VHH fusions were obtained as described above, and both the supernatant and precipitate of induced lysates and uninduced lysates were analyzed by 12% SDS-PAGE (). MBP-Nb fusion proteins (60 kDa) were mostly expressed in the supernatant, but His-Nb (15 kDa) and His-SUMO-Nb (35 kDa) fusions exhibited only 50% or less solubility. Notably, the MBP tag was more favourable in enhancing the solubility of Nbs compared to the other two tags.
Figure 3. Expression and solubility evaluation of VHH (Nb1 and Nb2) fusion proteins in E. coli: MBP-Nb (55 kDa) in E. coli TB1 (A); His-SUMO-Nb (30 kDa) in E. coli Rosetta (B); His-Nb (20 kDa) in E. coli BL21 (DE3) (C). Arrows indicate the fusion proteins. M, protein marker; IS, supernatant of induced lysates; IP, precipitate of induced lysates; US, supernatant of uninduced lysates; UP, precipitate of uninduced lysates.
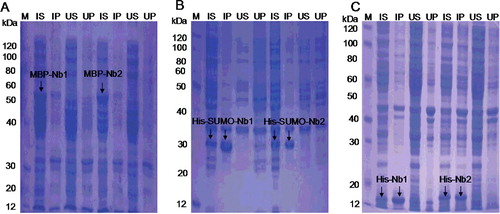
Actually, the MBP tag is a 42 kDa periplasmic protein encoded by the malE gene of E. coli [Citation21], which has been previously shown to increase the expression levels and solubility and assist in protein folding [Citation22–25]. SUMO is a small molecule UB protein found extensively in eukaryotes but notably absent in prokaryotes [Citation26]. The fusion of SUMO to target proteins is a highly regulated and dynamic process, thus leading to enhanced yield and solubility of recombinant proteins in E. coli [Citation27]. Therefore, our results were consistent with these previous reports.
Comparison of the fusion protein yield
The overall yield of purified fusion proteins was determined by assessing the protein concentration by the bicinchoninic acid asssy. Approximately 30–40, 50–65 and 18–20 mg of MBP-Nb, His-SUMO-Nb and His-Nb fusions were produced per liter of induced culture in shake flasks, respectively. These results indicate that the SUMO tag resulted in a significant increase in the yield of Nbs.
Indeed, many described sdAbs are expressed in E. coli cytoplasm with a yield of 1–10 mg per liter bacterial culture [Citation28]. Recently, several groups have demonstrated production of VHHs by refolding of inclusion bodies resulting in a promising yield [Citation19,Citation29]. However, the proper extraction and refolding of inclusion body proteins into bioactive forms is challenging. His-SUMO-Nb gave a yield of 50–65 mg/L in the cytoplasm as a soluble form in the present study. One advantage of being a cytoplasmic soluble protein is that it is convenient to be easily purified, making the process cost effective, without a complicated refolding process.
Analysis of the antigen-binding activity of the purified fusion VHHs
Indirect ELISAs were used to assess the antigen-binding activity of MBP-Nbs, His-SUMO-Nbs and His-Nbs. The generated binding bar graph () demonstrated that these fusion VHHs recognized their specific antigens. Among the fusion VHHs, the antigen-binding activity of His-SUMO-Nbs was the highest (P < 0.05). The affinity of the MBP-Nb fusion for its cognate antigen was significantly lower than that of the His-SUMO-Nb and His-Nb fusions (P < 0.05) (). We speculate that this result may be due to the steric hindrance of the MBP tag in the process of protein folding, which could interfere with antigen binding with the VHHs.
SUMO tag removal and purification of native VHH
The native VHH was released from the fusion protein by cleavage with Ulp1 (). Overnight incubation at 4 °C was performed to obtain 90% efficiency ((A)). The isolation of the native VHH protein from the cleavage reaction was done by TALON resin (Co2+) chromatography. The purified native VHH was about 15 kDa ((B), lane 2).
Figure 5. Removal of SUMO tag (A) and purification of native Nb2 (B). (A) Digestion efficiency of SUMO protease 1 (Ulp1) analyzed by 12% SDS-PAGE. (B) Purification of native Nb2. Lane M (A,B), protein maker; Lane 1 (A,B), purified His-SUMO-Nb2 fusion; Lane 2: His-SUMO-Nb2 digested by Ulp1 (A) and purified native Nb2 (B).
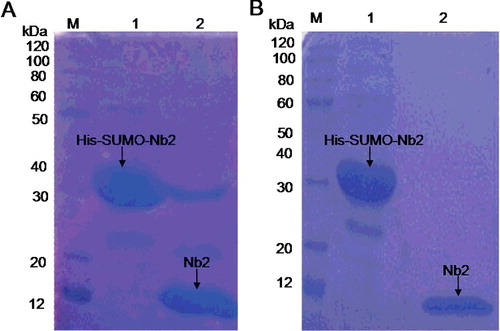
Affinity tags facilitate the process of protein expression and purification, but for therapeutic proteins, such as Abs, the final products must contain only authentic or native amino acid sequences. Therefore, fusion proteins should contain at least one specific protease cleavage site for removal of fusion tags [Citation30]. However, numerous proteases only recognize and cleave specific linear amino-acid sequences. The cleavage reaction of fusion proteins cannot be processed effectively, and often occurs at off-target cleavage sites. In contrast, SUMO protease Ulp1 recognizes the tertiary structure of SUMO. This feature prevents the erroneous cleavage of SUMO from the target protein [Citation31]. Therefore, the SUMO tag could be considered an ideal fusion tag for production of VHHs.
Measurements of the activity of purified native VHH
To investigate whether the His-SUMO tag had an important effect on the antigen-binding activity of VHH, a double antibody sandwich ELISA was carried out to analyze the antigen-binding activity of the purified native VHH and His-SUMO-Nb. As shown in , the antigen-binding activity of the native VHH (Nb2) was a little lower than that of the His-SUMO-Nb2 fusions, but without significant difference (P > 0.05). Both native Nb2 and the His-SUMO-Nb2 fusions developed considerable higher antigen-binding activity than that of positive serum.
Figure 6. Comparison of the antigen-binding activity of His-SUMO-Nb2 and native Nb2 using double antibody sandwich ELISA. The plates were coated with His-SUMO-Nb2 (2 nmol/L), natural Nb2 (2 nmol/L), positive serum (positive control) and negative serum (negative control). The antigen was a purified native Cap protein, and MBP-Nb1 was added as the second antibody, followed by the addition of HRP-conjugated anti-MBP tag mouse monoclonal antibody.
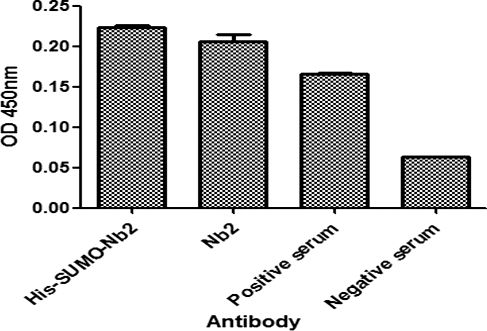
Interestingly, a previous study reported that MBP-NbHis6 fusion could improve Nbs yield significantly compared with the NbHis6 fusion (12–16.5 mg/L vs. 2–3.5 mg/L), and the MBP-NbHis6 fusions retained the antigen-binding activity of the native Nbs. However, MBP-NbHis6 fusions developed a significantly lower antigen-binding level than the native Nbs at the same concentration [Citation32]. Actually, the fusion tag had a negative effect on the antigen-binding activity of Nbs. In the present study, the His-SUMO-Nbs fusions achieved a yield of 50–65 mg/L and did not affect the antigen-binding activity of the VHHs considerably.
Conclusions
This study presents a new and effective strategy to produce functional VHHs in E. coli cytoplasm. The SUMO fusion tag facilitated a high yield of VHHs in E. coli in soluble form. The SUMO tag did not affect the antigen-binding activity of the VHHs, which could be easily removed from the fusion proteins. The method can be of general interest for efficient expression and purification of VHHs for research and industrial purposes.
Disclosure statement
The authors declare that they have no competing interests.
Additional information
Funding
References
- Hamers-Casterman C, Atarhouch T, Muyldermans S, et al. Naturally occurring antibodies devoid of light chains. Nature. 1993;363(6428):446–448.
- Roux KH, Greenberg AS, Greene L, et al. Structural analysis of the nurse shark (new) antigen receptor (NAR): molecular convergence of NAR and unusual mammalian immunoglobulins. Proc Natl Acad Sci USA. 1998;95(20):11804–11809.
- Muyldermans S, Cambillau C, Wyns L. Recognition of antigens by single-domain antibody fragments: the superfluous luxury of paired domains. Trends Biochem Sci. 2001;26(4):230–235.
- Muyldermans S. Nanobodies: natural single-domain antibodies. Ann Rev Biochem. 2013;82:775–797.
- Muyldermans S, Baral TN, Retamozzo VC, et al. Camelid immunoglobulins and nanobody technology. Vet Immunol Immunopathol. 2009;128(1–3):178–183.
- Vincke C, Muyldermans S. Introduction to heavy chain antibodies and derived nanobodies. Methods Mol Biol. 2012;911:15–26.
- Steyaert J, Kobilka BK. Nanobody stabilization of G protein-coupled receptor conformational states. Curr Opin Struct Biol. 2011;21(4):567–572.
- van der Linden RH, Frenken LG, de Geus B, et al. Comparison of physical chemical properties of llama VHH antibody fragments and mouse monoclonal antibodies. Biochim Biophys Acta. 1999;1431(1):37–46.
- Dumoulin M, Conrath K, Van Meirhaeghe A, et al. Single-domain antibody fragments with high conformational stability. Protein Sci. 2002;11(3):500–515.
- Stijlemans B, Conrath K, Cortez-Retamozo V, et al. Efficient targeting of conserved cryptic epitopes of infectious agents by single domain antibodies. African trypanosomes as paradigm. J Biol Chem. 2004;279(2):1256–1261.
- Vanlandschoot P, Stortelers C, Beirnaert E, et al. Nanobodies(R): new ammunition to battle viruses. Antiviral Res. 2011;92(3):389–407.
- Chambers SP, Austen DA, Fulghum JR, et al. High-throughput screening for soluble recombinant expressed kinases in Escherichia coli and insect cells. Protein Expr Purif. 2004;36(1):40–47.
- Studier FW, Moffatt BA. Use of bacteriophage T7 RNA polymerase to direct selective high-level expression of cloned genes. J Mol Biol. 1986;189(1):113–130.
- Ikura K, Kokubu T, Natsuka S, et al. Co-overexpression of folding modulators improves the solubility of the recombinant guinea pig liver transglutaminase expressed in Escherichia coli. Prep Biochem Biotechnol. 2002;32(2):189–205.
- Marblestone JG, Edavettal SC, Lim Y, et al. Comparison of SUMO fusion technology with traditional gene fusion systems: enhanced expression and solubility with SUMO. Protein Sci. 2006;15(1):182–189.
- Arndt KM, Muller KM, Pluckthun A. Factors influencing the dimer to monomer transition of an antibody single-chain Fv fragment. Biochemistry. 1998;37(37):12918–12926.
- Worn A, Pluckthun A. Stability engineering of antibody single-chain Fv fragments. J Mol Biol. 2001;305(5):989–1010.
- Fu X, Gao X, He S, et al. Design and selection of a camelid single-chain antibody yeast two-hybrid library produced de novo for the cap protein of porcine circovirus type 2 (PCV2). PloS One. 2013;8(3):e56222.
- Maggi M, Scotti C. Enhanced expression and purification of camelid single domain VHH antibodies from classical inclusion bodies. Protein Expr Purif. 2017;136:39–44.
- Waugh DS. Making the most of affinity tags. Trends Biotechnol. 2005;23(6):316–320.
- Duplay P, Bedouelle H, Fowler A, et al. Sequences of the malE gene and of its product, the maltose-binding protein of Escherichia coli K12. J Biol Chem. 1984;259(16):10606–10613.
- Cho HJ, Lee Y, Chang RS, et al. Maltose binding protein facilitates high-level expression and functional purification of the chemokines RANTES and SDF-1alpha from Escherichia coli. Protein Expr Purif. 2008;60(1):37–45.
- Guo W, Cao L, Jia Z, et al. High level soluble production of functional ribonuclease inhibitor in Escherichia coli by fusing it to soluble partners. Protein Expr Purif. 2011;77(2):185–192.
- Hayhurst A. Improved expression characteristics of single-chain Fv fragments when fused downstream of the Escherichia coli maltose-binding protein or upstream of a single immunoglobulin-constant domain. Protein Expr Purif. 2000;18(1):1–10.
- Bach H, Mazor Y, Shaky S, et al. Escherichia coli maltose-binding protein as a molecular chaperone for recombinant intracellular cytoplasmic single-chain antibodies. J Mol Biol. 2001;312(1):79–93.
- Kerscher O. SUMO junction-what's your function? New insights through SUMO-interacting motifs. EMBO Rep. 2007;8(6):550–555.
- Butt TR, Edavettal SC, Hall JP, et al. SUMO fusion technology for difficult-to-express proteins. Protein Expr Purif. 2005;43(1):1–9.
- Harmsen MM, De Haard HJ. Properties, production, and applications of camelid single-domain antibody fragments. Appl Microbiol Biotechnol. 2007;77(1):13–22.
- Noguchi T, Nishida Y, Takizawa K, et al. Accurate quantitation for in vitro refolding of single domain antibody fragments expressed as inclusion bodies by referring the concomitant expression of a soluble form in the periplasms of Escherichia coli. J Immunol Methods. 2017;442:1–11.
- Lee CD, Sun HC, Hu SM, et al. An improved SUMO fusion protein system for effective production of native proteins. Protein Sci. 2008;17(7):1241–1248.
- Mossessova E, Lima CD. Ulp1-SUMO crystal structure and genetic analysis reveal conserved interactions and a regulatory element essential for cell growth in yeast. Mol Cell. 2000;5(5):865–876.
- Salema V, Fernandez LA. High yield purification of nanobodies from the periplasm of E. coli as fusions with the maltose binding protein. Protein Expr Purif. 2013;91(1):42–48.