ABSTRACT
Polycystic ovarian syndrome (PCOS) is a common heterogeneous disorder characterized by multiple clinical features including chronic anovulation, infertility, polycystic ovaries and hyperandrogenic signs. Heredity is an important factor for the development of PCOS, but the search for the specific PCOS related genes is still in progress. Neuroendocrine dysregulation might play a crucial role in the PCOS development, thus the hypothalamic–pituitary secretion as well as gonadotropin receptor changes are important target of research. Two single nucleotide polymorphisms, rs12470652 c.872A>G/p.Asn291Ser and rs2293275 c.935G>A/p.Ser312Asn, in the Luteinizing Hormone/Choriogonadotropin Receptor (LHCGR) gene were studied in healthy Bulgarian women and PCOS patients. The prevalence of both polymorphisms was within the expected ranges for Caucasians and did not differ between the two groups. The heterozygous rs2293275 c.935G>A/p.Ser312Asn PCOS carriers reported infertility more often than both homozygous groups (40% vs. 12.5%, p = 0.022). The same polymorphism was associated with insulin levels in obese PCOS women. The obtained results showed that the rs2293275 c.935G>A/p.Ser312Asn genetic variant could modulate the clinical and hormonal characteristics of PCOS, especially in overweight and obese patients. Thus, larger studies in different ethnic groups and variable PCOS subgroups could reveal important genotype–phenotype associations.
Introduction
Polycystic ovarian syndrome (PCOS) is a common heterogeneous disorder characterized by multiple clinical features including menstrual disturbances, infertility, hirsutism, acne, insulin resistance and higher cardiovascular risk [Citation1,Citation2]. The increased prevalence of oligomenorrhea and hyperandrogenism in monozygotic twins of PCOS females compared to their other siblings has emphasized the importance of genetic predisposition for the development of PCOS [Citation3]. Moreover, daughters of PCOS patients have shown higher insulin and androgen levels during puberty in comparison with their peers born from non-PCOS mothers [Citation4].
Despite the obvious role of heritability, current attempts to identify specific PCOS related genes are still unsuccessful. Multiple studies have explored the genes involved in adrenal and ovarian steroid biosynthesis as well as metabolic pathways, but contradictory results have been reported (reviewed in [Citation5]). The neuroendocrine dysregulation in PCOS is another important target of research, as PCOS patients have shown higher baseline and stimulated luteinizing hormone (LH) concentrations as well as increased LH to FSH (follicle stimulating hormone) ratio, regardless of their androgen levels [Citation6]. The genes encoding gonadotropins and their receptors might influence the ovarian function and reproductive success [Citation7]. Recently, large genome-wide association studies (GWAS) identified the 2p16.3 locus carrying the Luteinizing Hormone/Choriogonadotropin Receptor (LHCGR) gene as a risk factor for PCOS at least in some ethnic groups [Citation8,Citation9].
Multiple LHCGR gene polymorphisms have been described, but only a few of them have been considered as functionally significant. Two well-studied single nucleotide polymorphisms are rs12470652 c.872A>G/p.Asn291Ser and rs2293275 c.935G>A/p.Ser312Asn in exon 10 of LHCGR causing amino acid changes at positions 291 (p.Asn291Ser) and 312 (p.Ser312Asn) near glycosylation sites of the receptor protein [Citation10]. The double polymorphic 291Ser-312Asn LHCGR haplotype was associated with two-fold increase of the choriogonadotropin and LH potency in comparison to the 291Asn-312Asn variant LHCGR and 291Asn-312Ser wild type LHCGR [Citation10]. Both polymorphisms have been investigated in women with PCOS, but the results differ in distinct ethnic groups [Citation11,Citation12].
Therefore, the present study aimed to evaluate the potential influence of rs12470652 p.Asn291Ser and rs2293275 p.Ser312Asn polymorphisms on the PCOS manifestations in Bulgarian patients.
Subjects and methods
Subjects
A total of 100 women in reproductive age (18–48 years) were included in the study. Sixty of them (60%) fulfilled the ESHRE/ASRM criteria for PCOS by having two of the following three features: (i) oligo- or anovulation, (ii) clinical and/or biochemical signs of hyperandrogenism, or (iii) polycystic ovaries [Citation1]. A total of 40 controls were recruited among medical staff and students. The PCOS women were chosen among the outpatients who presented with hirsutism and/or menstrual irregularities. No one of them had taken any hormonal pills in the last three months, excluding progestins for inducing menstruation in anovulatory patients. Prolactinoma, untreated thyroid diseases, Cushing's syndrome, adrenal hyperplasia, androgen-producing tumour or other serious illnesses were excluded in all of them. The control group for a comparison of the genetic polymorphism prevalence consisted of non-hirsute women with regular menstrual cycle. The PCOS patients were significantly younger than controls (25.62 ± 4.95 vs. 35.50 ± 7.99, p < 0.001). The distribution of the investigated genotypes in healthy females was in agreement with the Hardy–Weinberg equilibrium.
Study protocol
All patients underwent a complete general assessment, including height, weight, waist circumference (WC), blood pressure, general condition, presence of hirsutism [modified Ferriman–Gallwey (FG) score ≥ 8] and acne. The history of menarche onset, pregnancies and infertility was self-reported. Blood samples for laboratory assay were collected on the third to fifth day from a spontaneous or induced (in case of amenorrhea) menstrual cycle in the morning between 08:00 and 10:00 am after an overnight fast. Fasting glucose, high-density lipoprotein cholesterol levels (HDL-ch), triglycerides (TG) and total cholesterol were also determined. The biochemical parameters were determined enzymatically by an automatic analyzer (Cobas Mira Plus; Hoffmann La Roche). Blood samples for immunoreactive insulin (IRI), total testosterone (T), LH, FSH and dehydroepiandrosterone-sulphate (DHEAS) were obtained. The hormonal levels were measured with commercially available DELFIA and RIA kits. Body mass index (BMI) was calculated according to the formula: BMI = weight (kg)/height (m)2. Homeostatic model assessment index of insulin resistance (HOMA-IR) was calculated according to the equation: fasting serum insulin (µU/mL) × fasting plasma glucose (mmol/L) /22.5 [Citation13].
Genetic polymorphisms
Total genomic DNA from peripheral ethylenediaminetetraacetic acid (EDTA)-blood was extracted using standard salt-extraction procedures. Both polymorphisms rs12470652 p.Asn291Ser and rs2293275 p.Ser312Asn were genotyped simultaneously because of their close localization in the genome (). Polymerase chain reaction (PCR) was performed with specific primers designed on the basis of open access program Primer3 (http://bioinfo.ut.ee/primer3-0.4.0/): Forward 5'-TAGGACAATGGTGCAGAACG-3', and Reverse 5'-CTTGATGCCAATTGCAAAGA-3'.
Figure 1. LHCGR rs12470652 c.872A>G/p.Asn291Ser and rs2293275 c.935G>A/ p.Ser312Asn polymorphisms – part of the LHCGR amino-acid chain and an example of A/G and G/A heterozygous sample analyzed by Sanger sequencing.
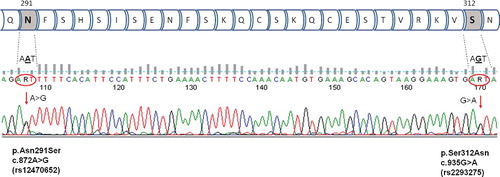
PCR was performed (Applied Biosystems 2720 Thermal Cycler, USA) in a final volume of 15 μL containing 10.8 μL dH2O, 1.0 μL genomic DNA (100–200 ng), 0.5 μL of each primer (with concentration 10 pmol/μL), 1.5 μL 10x Buffer (GeNetBio, Korea), 0.6 μL of 10 (mmol/L)/μL deoxyribonucleoside triphosphates (dNTPs) and 0.1 μL Taq-polymerase (GeNetBio, Korea). The cycling conditions were as follows: an initial denaturation at 95 °C for 5 min, followed by 30 cycles at 95 °C for 30 s, 60 °C for 40 s and 70 °C for 1 min, with a final extension step of 5 min at 72 °C in the last cycle.
Amplified products were analysed by Sanger sequencing. First, an aliquot of 1.0 μL of each product was treated by Exonuclease–Shrimp Alkaline Phosphatase clean-up enzymatic digestion by ExoSAP-IT (Affymetrix, USA) according to the manufacturer's instructions. Sequence reactions were carried out on the ExoSAP-IT-purified PCR products using ABI BigDyeTerminator Cycle Sequencing Mix v.3.1. (Applied Biosystems, USA). The reaction was performed in a final volume of 10 μL containing 2.0 μL of the ExoSAP-PCR product, 0.4 µL BigDye Terminator Mix, 0.4 µL of 10 pmol primer (forward or reverse), 2 µL of 5 × Sequencing Buffer and dH2O to the final volume. After an initial denaturation step at 96 °C for 5 min, 30 cycles of the reaction were performed at 96 °C for 20 s, 55 °C for 20 s and 60 °C for 2 min; followed by a final extension step at 60 °C for 5 min. Samples were dissolved in HiDi Formamide after 125 mmol/L EDTA/ethanol precipitation and processed on an ABI Prism 3130 Genetic Analyzer (Applied Biosystems, USA) according to the manufacturer's protocols. The obtained results were analysed and interpreted by using the FinchTV software (Geospiza Inc.). As a reference, the published LHCGR ENST00000294954.11 sequence was used.
The experimental protocols were explained to all participants and written informed consent was obtained. The study was approved by the institutional review board.
Statistical analysis
All results were expressed as mean values with standard deviation (±SD)/median/. Frequency analyses and descriptive statistics were used. Differences between dichotomous variables were tested with Chi-square or Fisher's exact test. Differences among the two groups were evaluated with independent sample t-test or Mann–Whitney test after a Kolmogorov–Smirnov test for normality of the distribution. A Kruskal–Wallis test was used for multiple comparisons. A p-level < 0.05 was considered statistically significant. The data were analysed by MedCalc Software for Windows, version 15.0 (MedCalc Software, Ostend, Belgium).
Results and discussion
The prevalence of both LHCGR polymorphisms did not differ between patients and controls. The rs12470652 p.Asn291Ser minor allele G was found in 7.5% of healthy women and 6.8% of those with PCOS (p = 1.00). The genotype distribution of the investigated polymorphisms is presented in . Both polymorphisms were significantly related (p = 0.017).
Table 1. Genotype frequency of the investigated polymorphism in patients and controls. (A) rs12470652 p.Asn291Ser (c.872A>G) (n = 99) and (B) rs2293275 p.Ser312Asn (c.935G>A) (n = 100).
The established prevalence of rs12470652 p.Asn291Ser minor allele G and rs2293275 p.Ser312Asn minor allele A in the healthy Bulgarian women was within the expected ranges for Caucasians, and both polymorphisms were significantly linked as shown in other studies [Citation10,Citation12,Citation14]. Several other studies did not find any associations between the polymorphisms and the PCOS development [Citation12,Citation15]. However, the rs2293275 p.Ser312Asn polymorphic A allele was shown to be a significant risk factor for PCOS in Sardinian and Egypt patients, while in south India the same allele was considered as protective [Citation11,Citation16,Citation17]. These discrepancies between the studies might be explained by ethnic peculiarities, variable PCOS definitions and different control group inclusion criteria as well as pronounced PCOS clinical heterogeneity per se.
The most important characteristics of the patients with different rs2293275 p.Ser312Asn genotypes are shown in . The rs2293275 p.Ser312Asn polymorphism did not influence the metabolic or hormonal parameters of the whole group of PCOS patients (p > 0.05 for all). This LHCGR polymorphism was not significantly associated with the presence of hirsutism or oligomenorrhea (p > 0.05 for all). The prevalence of acne was higher in PCOS minor A allele carriers in comparison to GG carriers but the differences did not reach statistical significance (7.4% vs. 27.3%, p = 0.091). The heterozygous carriers reported infertility more often than both homozygous groups (40% vs. 12.5%, p = 0.022). The influence of the rs12470652 p.Asn291Ser variant on the metabolic and hormonal levels in PCOS patients was not established because of the low polymorphic variant prevalence.
Table 2. Clinical and laboratory characteristics of the PCOS patients with different rs2293275 p.Ser312Asn genotypes.
Oligo-ovulation in PCOS women often results in sub-fertility or infertility; nevertheless more than half of these patients could conceive spontaneously [Citation18]. Thus, exploring the environmental and genetic factors that might influence the gametogenesis and embryo-development would be of clinical importance. Interestingly, our study found a significantly increased risk of infertility in the heterozygous rs2293275 p.Ser312Asn AG carriers. Recently, a study of women undergoing in vitro fertilization reported that the presence of GG genotype was associated with the highest number of clinical pregnancies. However, the same linear association between the G alleles and the number of pregnancies became stronger after removing the PCOS cases from the cohort, suggesting different influence of the rs2293275 p.Ser312Asn polymorphism on the fertility in PCOS patients and in women with other conditions requiring in vitro fertilization procedures [Citation19].
In non-obese patients, the rs2293275 p.Ser312Asn variant was not significantly related to any of the clinical, hormonal or metabolic characteristics (p > 0.05 for all). However, the overweight and obese PCOS women (BMI ≥25) carrying the polymorphic A allele reported menarche at a later age in comparison to other patients (13.00 vs. 12.08, p = 0.044). The LH to FSH ratio tended to be higher in the GG than in the GA and AA polymorphic carriers (2.13 ± 1.80 /1.47/ vs. 1.19 ± 1.74 /0.61/, p = 0.066). In obese PCOS patients (BMI ≥30), the prevalence of the polymorphic A allele was related to lower insulin levels (15.01 ± 6.91 /14.60/ vs. 26.80 ± 14.51 /22.80/, p = 0.045), higher HDL levels (1.29 ± 0.34 /1.19/ vs. 1.01 ± 0.12 /1.00/, p = 0.038), as well as a tendency to a higher FG score (12.43 ± 4.68 /12/ vs. 9.37 ± 4.47 /8/, p = 0.082).
The rs12470652 p.Asn291Ser and rs2293275 p.Ser312Asn polymorphisms have been associated with increased LHCGR functional activity and thus, a compensatory decrease in LH levels and LH to FSH ratio might be expected in minor allele carriers [Citation10]. Similar results were obtained in overweight south Indian PCOS patients [Citation17]. The present data in overweight and obese PCOS women supported these findings, as a tendency to increased LH to FSH ratio was observed in the common GG genotype carriers compared to the patients with GA and AA genotypes in our study. On the contrary, an Egypt study observed the lowest LH levels and LH to FSH ratio in GG patients compared to A allele carriers [Citation16]. However, most studies did not show associations between the rs2293275 p.Ser312Asn polymorphism and gonadotropin secretion in lean PCOS patients or infertile women [Citation11,Citation19]. Consequently, the LHCGR variant might exert population-specific modulation effects on the hypothalamic–pituitary–gonadal axis in obese PCOS patients.
Another important finding of our study was the association between the rs2293275 p.Ser312Asn polymorphism and the insulin levels in obese PCOS patients. Bulgarian A-allele carriers showed decreased insulin levels and better lipid profile with increased HDL-cholesterol levels, but tended to be more hirsute. Probably, the LHCGR rs2293275 p.Ser312Asn variant might aggravate the phenotype expression of PCOS even in patients with uncomplicated obesity and relatively lower insulin levels. Other studies did not find an association between the rs2293275 p.Ser312Asn polymorphism and serum fasting insulin levels, but interrelations between the variant A allele presence and the indices of insulin resistance and secretion have already been reported [Citation16,Citation17]. Hyperinsulinemia could enhance the ovarian androgen response to gonadotropins in PCOS patients [Citation20]. Obesity per se is associated with relatively decreased LH levels in PCOS patients [Citation21], but gonadotropin–insulin interrelations and their effects on the polycystic ovaries might be modulated by the LHCGR receptor functional activity.
The main limitation of this study was the small number of participants. Nevertheless, the results showed important associations between the rs2293275 p.Ser312Asn variant and the PCOS characteristics suggesting the need of more LHCGR polymorphism studies in different PCOS subgroups.
Conclusions
The present study showed the potential influence of the rs2293275 p.Ser312Asn polymorphism on infertility in PCOS women. This genetic variant could modulate the gonadotropin ratio and metabolic characteristics of the overweight and obese patients, suggesting that the LHCGR polymorphism might aggravate the PCOS phenotype expression in obese women. Further larger studies in different ethnic groups are needed to reject or prove this hypothesis as well as to clarify the proper associations between the different LHCGR polymorphisms and the PCOS clinical manifestations.
Disclosure statement
The authors report no conflicts of interest.
Additional information
Funding
References
- Rotterdam ESHRE/ASRM-Sponsored PCOS consensus workshop group. Revised 2003 consensus on diagnostic criteria and long-term health risks related to polycystic ovary syndrome (PCOS). Hum Reprod. 2004;19(1):41–47.
- Conway G, Dewailly D, Diamanti-Kandarakis E, et al. ESE PCOS Special Interest Group. The polycystic ovary syndrome: a position statement from the European society of endocrinology. Eur J Endocrinol. 2014;171(4):P1–29.
- Vink JM, Sadrzadeh S, Lambalk CB, et al. Heritability of polycystic ovary syndrome in a Dutch twin-family study. J Clin Endocrinol Metab. 2006;91(6):2100–2104.
- Sir-Petermann T, Codner E, Pérez V, et al. Metabolic and reproductive features before and during puberty in daughters of women with polycystic ovary syndrome. J Clin Endocrinol Metab. 2009;94(6):1923–1930.
- Unluturk U, Harmanci A, Kocaefe C, et al. The genetic basis of the polycystic ovary syndrome: a literature review including discussion of PPAR-gamma. PPAR Res. 2007 [cited 2017 Nov 1];2007:49109. DOI: 10.1155/2007/49109
- Lewandowski KC, Cajdler-Luba A, Bieńkiewicz M, et al. Women with oligo-/amenorrhoea and polycystic ovaries have identical responses to GnRH stimulation regardless of their androgen status: comparison of the Rotterdam and Androgen Excess Society diagnostic criteria. Neuro Endocrinol Lett. 2011;32(6):847–856.
- Casarini L, Santi D, Marino M. Impact of gene polymorphisms of gonadotropins and their receptors on human reproductive success. Reproduction. 2015;150(6):R175–R184.
- Chen ZJ, Zhao H, He L, et al. Genome-wide association study identifies susceptibility loci for polycystic ovary syndrome on chromosome 2p16.3, 2p21 and 9q33.3. Nat Genet. 2011;43(1):55–59.
- Shi Y, Zhao H, Shi Y, et al. Genome-wide association study identifies eight new risk loci for polycystic ovary syndrome. Nat Genet. 2012;44(9):1020–1025.
- Piersma D, Verhoef-Post M, Look MP, et al. Polymorphic variations in exon 10 of the luteinizing hormone receptor: functional consequences and associations with breast cancer. Mol Cell Endocrinol. 2007;276(1–2):63–70.
- Capalbo A, Sagnella F, Apa R, et al. The 312 N variant of the luteinizing hormone/choriogonadotropin receptor gene (LHCGR) confers up to 2·7-fold increased risk of polycystic ovary syndrome in a Sardinian population. Clin Endocrinol (Oxf). 2012;77(1):113–119.
- Valkenburg O, Uitterlinden AG, Piersma D, et al. Genetic polymorphisms of GnRH and gonadotrophic hormone receptors affect the phenotype of polycystic ovary syndrome. Hum Reprod. 2009;24(8):2014–2022.
- Matthews DR, Hosker JP, Rudenski AS, et al. Homeostasis model assessment: insulin resistance and beta-cell function from fasting plasma glucose and insulin concentrations in man. Diabetologia. 1985;28(7):412–419.
- Simoni M, Tüttelmann F, Michel C, et al. Polymorphisms of the luteinizing hormone/chorionic gonadotropin receptor gene: association with maldescended testes and male infertility. Pharmacogenet Genomics. 2008;18(3):193–200.
- Almawi WY, Hubail B, Arekat DZ, et al. Leutinizing hormone/choriogonadotropin receptor and follicle stimulating hormone receptor gene variants in polycystic ovary syndrome. J Assist Reprod Genet. 2015;32(4):607–614.
- El-Shal AS, Zidan HE, Rashad NM, et al. Association between genes encoding components of the Leutinizing hormone/Luteinizing hormone-choriogonadotrophin receptor pathway and polycystic ovary syndrome in Egyptian women. IUBMB Life. 2016;68(1):23–36.
- Thathapudi S, Kodati V, Erukkambattu J, et al. Association of luteinizing hormone chorionic gonadotropin receptor gene polymorphism (rs2293275) with polycystic ovarian syndrome. Genet Test Mol Biomarkers. 2015;19(3):128–132.
- Vanky E, Backe B, Carlsen SM. Sex ratio and pregnancy complications according to mode of conception in women with polycystic ovary syndrome. Acta Obstet Gynecol Scand. 2009;88(11):1261–1266.
- Lindgren I, Bååth M, Uvebrant K, et al. Combined assessment of polymorphisms in the LHCGR and FSHR genes predict chance of pregnancy after in vitro fertilization. Hum Reprod. 2016;31(3):672–683.
- Tosi F, Negri C, Perrone F, et al. Hyperinsulinemia amplifies GnRH agonist stimulated ovarian steroid secretion in women with polycystic ovary syndrome. J Clin Endocrinol Metab. 2012;97(5):1712–1719.
- Morales AJ, Laughlin GA, Bützow T, et al. Insulin, somatotropic, and luteinizing hormone axes in lean and obese women with polycystic ovary syndrome: common and distinct features. J Clin Endocrinol Metab. 1996;81(8):2854–2864.