ABSTRACT
The aim of this study was to construct recombinant adenovirus vector carrying a short hairpin RNA (shRNA) that can exclusively target cyclooxygenase-2 (COX-2) gene expression and observe its inhibitory effect on hepatic fibrosis. We designed and synthesized three oligonucleotide sequences, and cloned those into a shuttle vector, pYr-1.1-hU6-EGFP, after annealing. The restriction-enzyme digestion and sequencing analyses confirmed that the constructed recombinant eukaryotic expression vector was correct. A recombination reaction using LR Clonase was performed for the pYr-1.1-hU6-EGFP COX-2shRNA and the adenovirus vector pAd/BL-DEST to form Ad-COX-2shRNA. The adenoviruses containing the recombinant plasmids were transfected into hepatic stellate cells (HSCs). The transfection efficiency of the three COX-2 shRNAs exceeded 70%. Reverse transcription PCR (RT-PCR) and western blot confirmed that the target gene expression was decreased at the level of mRNA and protein, and the interference effect of COX-2 shRNA-1 was better than that of the other two COX-2 shRNAs. COX-2 shRNA-1 recombinant adenovirus vectors (1 × 109 PFU/mL) were injected via the tail vein into rats fed a high-fat diet with a 40% carbon tetrachloride peanut oil lavage, which induced liver fibrosis. Rats were euthanized at the end of the 12th week, and their liver was removed. Liver expression of COX-2 mRNA and protein was detected by RT-PCR and immunohistochemistry, respectively. RT-PCR and immunohistochemistry showed that COX-2 shRNA-1 inhibited COX-2 expression in liver tissue. Hematoxylin/eosin and Masson staining showed that COX-2 shRNA-1 ameliorated the severity of liver fibrosis. The COX-2 shRNA eukaryotic expression vectors were constructed successfully and COX-2 shRNA-1 ameliorated liver fibrosis.
Introduction
COX-2 is connected with liver fibrosis and selective COX-2 inhibitors can relieve or prevent the formation and development of liver fibrosis [Citation1,Citation2]. However, the use of COX-2 inhibitors is limited due to the risk of adverse cardiovascular events and gastrointestinal bleeding [Citation3]. Therefore, increased attention has been given to the nucleic acid method of blocking gene expression. There are three main techniques to block or inhibit the expression of genes: antisense oligonucleotides, ribozymes and RNA interference (RNAi) [Citation4–6]. In the past, the antisense nucleic acid and enzyme technologies played important roles in inhibiting or blocking gene expression, but it is difficult to block protein expression to the levels required for the treatment of disease. Compared with previous gene-blocking technologies, RNAi is more efficient and specific for blocking the expression of a target gene [Citation7,Citation8].
The RNAi method for gene silencing is mediated by double-stranded RNA in an organism [Citation9,Citation10], and double-stranded RNA can be produced through RNA virus infection, exogenous gene transfer and other methods [Citation11,Citation12]. After transfection into a cell, the double-stranded RNA participates in RNAi in two ways [Citation13]. First, the double-stranded RNA is broken into double- or single-chain small interfering RNA (siRNA) by the Dicer enzyme, forming complexes with some proteins such as argonaute. These complexes then combine with mRNA that is complementary to the siRNA, and the mRNA is subsequently degraded by RNA enzymes. Second, the double-stranded RNA is amplified by RNA-directed RNA polymerase (RdRP) and then fragmented into siRNA by the Dicer enzyme. The second mechanism is different in that in the first mechanism, siRNA is used as the primer, and mRNA is used as the template. RdRP then catalyzes the compounding of these complexes with complementary chains of mRNA, and as a result, mRNA becomes double-stranded RNA, which is then broken into siRNAs by the Dicer enzyme. These newly formed siRNAs can also induce RNAi. Increasing the amount of siRNA in the cell can increase the inhibition of gene expression.
With the continuous research on the molecular mechanism of RNAi, as a new means to block the expression of a gene, the RNAi methodology is also being perfected and becoming a routine technology [Citation14,Citation15]. At present, there are many methods for producing siRNA, which are mainly divided into two categories [Citation8,Citation12]: first, siRNA is derived from in vitro transcription or chemical synthesis and mediates RNAi after transfection into cells [Citation16,Citation17], and second, a shRNA (short hairpin RNA) expression vector is built to mediate RNAi after transfection into cells [Citation7,Citation13,Citation18–21]. We aimed to build a COX-2 shRNA expression vector, which will be a valuable tool for studies on COX-2 function and gene therapy involving the COX-2 gene. Studies on the use of RNAi technology to treat liver fibrosis have been reported, including using siRNAs targeting transforming growth factor-β1 (TGF-β1) to inhibit the activation and proliferation of hepatic stellate cells (HSCs), suppress extracellular matrix (ECM) synthesis, and block liver fibrosis [Citation22]. However, similar studies on the COX-2 gene have not been reported yet.
Materials and methods
Plasmids, cells and animals
The shuttle plasmid pYr-1.1-hU6-EGFP and adenovirus genomic plasmid Adeno-pAd/BL-DEST were purchased from Yingrun Biotechnologies Inc. (Changsha, China). The adenovirus packaging cell line HEK293A cells were provided by Invitrogen (Carlsbad, CA, USA). The hepatic stellate cells-T6 (HSC-T6) and Sprague Dawley (SD) rats weighing 200 ± 20 g were obtained from the Shanghai Institutes for Biological Sciences, CAS (Shanghai, China; license number of SD rats: SCXK 2013-0016). All animal procedures were approved by the Animal Care and Use Committee of Nanhua Hospital affiliated to University of South China.
Construction of COX-2 shRNA expression vector
Choice of target sequences and primers synthesis
According to one 21-nt rat COX-2shRNA and two 29-nt human COX-2 shRNA sequences obtained from the TRC and OriGene GenBank databases and the shRNA design principles [Citation23], we choose three target sequences: the COX-2shRNA-1 sequence, 5′-CCTCGTCCAGATGCTATCTTT-3′; COX-2shRNA-2 sequence, 5′-AGTTCCAGTATCAGAACCGCATTGCCTCT-3′; and COX-2shRNA-3 sequence, 5′-GACACCTTCAACATTGAAGACCAGGAGTA-3′. The primers for the target sequence () were synthesized and annealed in annealing buffer (10 mmol L−1 Tris-HCl, pH 8.0, 50 mmol L−1 NaCl, 1 mmol L−1 ethylene diamine tetraacetic acid).
Table 1. Primers used for the single-stranded oligonucleotide synthesis of the target sequence.
Bsal II digestion and connection reaction
The shuttle plasmid was digested by BsaI II restriction enzyme (New England Biolabs LTD, Beijing, China) to produce linear pYr-1.1-hU6-EGFP fragments. The annealed, double-stranded DNA of COX-2 and linear pYr-1.1-hU6-EGFP fragments were connected using T4 DNA ligase (New England Biolabs LTD, Beijing, China) to form pYr-1.1-hU6-EGFP-COX-2shRNA recombinant vectors.
Transformation, Xho I digestion and sequencing
The COX-2shRNA recombinant vectors were transformed into the host bacteria (DH5a, Yingrun Biotechnologies Inc., Changsha, China), and picked the cloning for identification by Xho I digestion. The fragmentation of the digestion was examined by agarose gel electrophoresis, and sequencing analysis was performed using an ABI 3730 automated DNA sequencer (Perkin-Elmer Applied Biosystems, Foster City, USA).
Construction of shRNA recombinant adenovirus vector
The pYr-1.1-hU6-EGFP-COX-2shRNA 1,2,3 vector and the adenovirus vector pAd/BL-DEST were recombined using LR Clonase to form Ad-COX-2shRNA 1,2,3. The recombinant adenovirus vector was transfected into HEK293A cells and the titer of virus was calculated by the following formula: titer of virus [TU µL−1] = number of fluorescent cells/corresponding dilution ratio.
Identification of the optimal shRNA sequence in vitro
Cell culture and transfection
The HSC-T6 were cultured in Dulbecco's modified Eagle's medium (DMEM, USA) containing 10% fetal bovine serum (FBS, USA), glutamine (20 mmol L−1), penicillin (100 U mL−1) and streptomycin (100 μg mL−1). A total of 107 HSC-T6 in the logarithmic growth phase were inoculated into a 250-mL bottle. After 24 h, the media were changed to media containing COX-2shRNA1,2,3, or empty adenovirus vector. Cells were collected after transfection for 24, 48 and 72 h, respectively. Then, the green fluorescence expression was observed under a fluorescence microscope (Olympus Inc., Tokyo, Japan).
COX-2 mRNA expression in HSCs
The COX-2 mRNA expression in HSCs was examined by reverse transcription polymerase chain reaction (RT-PCR), as reported previously [Citation24]. Rat glyceraldehyde-3-phosphate dehydrogenase (GAPDH) served as an internal standard to quantify the PCR products. The primers for the COX-2 gene consisted of the forward primer 5′-AATGAGTACCGCAAACGCTT-3′ and the reverse primer 5′-ATCTAGTCTGGAGTGGGAGG-3′ and the GAPDH primer sequences used were 5′-ACCAC AGTCCATGCCATCAC-3ʹ and 5′-TCCACCACCCTGTTGCTGTA-3′.
COX-2 proteins expression in HSCs
The expression of COX-2 proteins in HSCs was examined by western blot, as reported previously [Citation25]. GAPDH served as an internal standard to quantify the expression of COX-2.
Anti-fibrosis effect of recombinant adenovirus vectors in vivo
Grouping and treatment of SD rats
The SD rats were randomly assigned to the following four groups (10 animals per group): normal control, model, Ad-COX-2-shRNA-1 (COX-2-shRNA-1) and empty adenovirus vector (empty vector) group. Except for the normal control group, other groups were fed a high-fat diet with a 40% carbon tetrachloride (CCL4) peanut oil lavage (2 mL∙kg−1∙5 day−1) to establish the rat model of liver fibrosis. One week after the model was established, the Ad-COX-2-shRNA-1 and empty adenovirus vector (1 × 109 PFU mL−1) were injected in the rats via the tail vein. The same volume of normal saline was given to the normal control group and model group. After transfection for 12 weeks, the rats were euthanized (anesthetized by injection of 1 mL 10% chloral hydrate and then decapitated) and their liver was removed.
Expression of COX-2 mRNA in liver tissue
The COX-2 mRNA expression in liver tissue was detected by RT-PCR. Total RNA was extracted from liver tissue using TRIzol reagent (Invitrogen, USA). The methods, procedures and primers are the same as those used in the experiments for COX-2 mRNA expression in HSCs.
Expression of COX-2 proteins in liver tissue
The COX-2 proteins expression in liver tissue was detected by immunohistochemical staining. The samples were immerged in peroxidase blockers and then subjected to conventional slicing, heating repair and washing with distilled water and phosphate buffered saline (PBS). After incubation with the primary rabbit anti-rat COX-2 polyclonal antibody (Abcam, USA) overnight, the samples were blocked by bovine serum albumin (BSA; Sigma, USA) and then washed three times with TBS+Tween (TBST) buffer. Then, the samples were incubated with the second antibody conjugated with biotin and streptavidin-peroxidase at room temperature. After three more washes, the samples underwent DAB (3,3′-diaminobenzidine) staining, hematoxylin staining and finally dehydration and were then mounted. Slides were observed under light microscope (Olympus Inc., Tokyo, Japan). The brown granules in the cytoplasm indicate COX-2-positive cells, which were present in large numbers in the portal area and hepatic sinusoid. Five high-magnification (400×) fields were randomly selected and analyzed using Image-pro Plus5.1 Image Analysis Software. For each field, 200 cells were counted and the average percentage was calculated.
Pathological changes in liver tissue
The lipid droplets formed in liver cells were confirmed by liver histology staining with Sudan IV. The fibrous lesion severity was analyzed by hematoxylin/eosin and Masson staining. The fibrous lesion area of the liver tissue was measured with an image analyzer.
Data analysis
The results are expressed as mean values with standard deviation (±S.D.). Statistical analysis was performed using one-way analysis of variance as appropriate, and the comparisons between each two groups were analyzed by the LSD-t test using SPSS software for Windows, version 18.0 (SPSS Inc., Chicago, IL). P < 0.05 was considered significant.
Results and discussion
Construction of COX-2 shRNA eukaryotic expression vector
Choice of target sequences and length design of shRNA
RNA interference has become a powerful technique for studying gene function, biological pathways and the physiology of diseases [Citation12,Citation13,Citation21]. RNAi, which is designed for different parts of the target mRNA, has a strong effect on the inhibition of the target gene [Citation16]. According to the design principle of shRNA [Citation23], we chose three sequences of COX-2 shRNA from the TRC and OriGene GenBank databases for this study. RNAs are usually designed as dsRNA for application, and their length is an important factor affecting the expression of the target gene. In mammalian cells, more than 30 base pairs of exogenous dsRNA can induce the intracellular interferon system, activating RNA-dependent protein kinase (PKR), which has a broad and non-specific effect on inhibition [Citation7,Citation22]. Thus, the length of the designed shRNA in this experiment was no more than 30 nucleotides.
Transformation of COX-2shRNA recombinant vectors
There was a lethal gene, CCDB, located between the two BsaI sites in the empty pYr-1.1-hU6-EGFP vector but not in the COX-2shRNA recombinant vectors. When foreign shRNA can replace the CCDB expression box in ordinary competent DH5a, this plasmid can grow in the corresponding bacteria. COX-2shRNA-1,2,3 vector in the ordinary competent DH5a grew corresponding colonies in this study, indicating that the COX-2-shRNA-1,2,3 sequences were inserted into the vector successfully.
Xho I digestion identification and sequencing analysis of COX-2shRNA recombinant vectors
In this study, compared with the correct sequences, the COX-2shRNA-1,2,3 sequence inserted into the vector identified by Xho I restriction enzyme digestion and sequencing analysis confirmed that the construction of recombinant eukaryotic expression vector was correct ( and and Online Supplement Figures S1–S4).
Identification of the optimal shRNA sequence in vitro
Transfection of Ad-COX-2shRNA
The adenovirus containing the recombinant plasmids was transfected into HSC-T6 cells; the cells emitted green fluorescence upon excitation with blue light under a fluorescence microscope. Ten fields were randomly selected, and the ratio between the positive cells and the total cells was used as an indicator of plasmid transfection efficiency. Green fluorescence was observed for the Ad-COX-2-shRNAs, and Ad-empty vector groups after 24 h, and the transfection efficiency was above 70%, indicating that the transfections were successful. There was no green fluorescence in the blank control group (Online Supplement Figure S5).
COX-2 mRNA expression in HSCs
The HSC-T6 cell lines were infected by Ad-COX-2-shRNAs and Ad-empty vector, respectively. The expression of COX-2 mRNA was analyzed by RT-PCR. After 24 h, the expression levels of COX-2 mRNA were significantly decreased in HSC-T6 cell lines infected by Ad-COX-2-shRNA-1 compared to the Ad-empty vector and blank groups. After 48 and 72 h, the COX-2 mRNA expression levels were significantly suppressed in HSC-T6 cell lines infected by Ad-COX-2-shRNA-1,2,3 compared to the Ad-empty vector and blank groups, and the interference effect of COX-2 shRNA-1 was better than that observed in the Ad-COX-2-shRNA-2,3 groups ().
Figure 3. Time course of COX-2 mRNA expression in HSCs assessed by RT-PCR. M, molecular size marker; Lane 1: COX-2-shRNA-1 group; Lane 2, COX-2 shRNA-2 group; Lane 3, COX-2 shRNA-3 group; Lane 4, empty vector group; Lane 5, blank control group. The three gels show different expression of COX-2 mRNA in HSCs at 24, 48 and 72 h (from left to right). (a) P < 0.05 vs. the Ad-empty vector group; (b) P < 0.05 vs. the blank group.
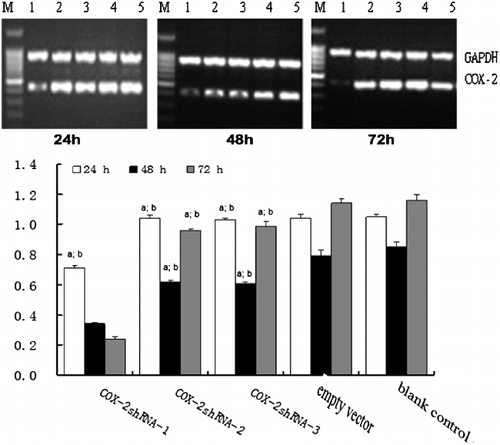
COX-2 protein expression in HSCs
The HSC-T6 cell lines were infected with Ad-COX-2-shRNAs and Ad-empty vector for 48 h. The expression of COX-2 protein was analyzed by Western blot. Compared with the Ad-empty vector group, the expression of COX-2 in the Ad-COX-2-shRNA-1 group was significantly suppressed (P < 0.05), whereas there were no significant differences with the other groups (P > 0.05) ().
Anti-fibrosis effect of recombinant adenovirus vectors in vivo
COX-2 mRNA expression in the liver tissue of rat
The COX-2 mRNA expression in liver tissue was analyzed by RT-PCR. Compared with the normal control group, the COX-2 mRNA expression in the model group was significantly higher (P < 0.05), indicating COX-2 mRNA expression was enhanced in rats liver tissue with hepatic fibrosis. The COX-2 mRNA expression in the Ad-COX-2-shRNA1 group was higher than that in the normal control group and was lower than that in the model group, demonstrating that Ad-COX-2-shRNA successfully silenced COX-2 expression in the liver tissue ( and ).
Figure 5. COX-2 mRNA expression in rat liver tissue assessed by RT-PCR. M, molecular size marker; Lane 1, normal control group; Lane 2, model group; Lane 3, COX-2 shRNA-1 group; Lane 4, empty vector group.
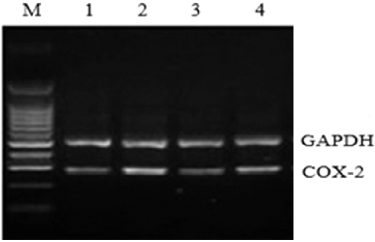
Table 2. COX-2 mRNA expression in liver tissue (x ± S.D.).
COX-2 protein expression in the liver tissue of rat
The COX-2 protein expression in liver tissue was analyzed by immunohistochemistry. Compared with the normal control group, the COX-2 protein expression in the model group was significantly higher (P < 0.05), indicating that the COX-2 protein expression was enhanced in the liver tissue with hepatic fibrosis. The COX-2 protein expression in the COX-2 shRNA-1 group was higher than that in the normal control group and was lower than that in the model group, demonstrating that COX-2 shRNA-1 successfully silenced COX-2 expression in the liver tissue with hepatic fibrosis ( and ).
Figure 6. COX-2 protein expression in liver tissue of SD rats (immunohistochemistry, 400×). Normal control group (A); model group (B); COX-2 shRNA-1 group (C); empty vector group (D).
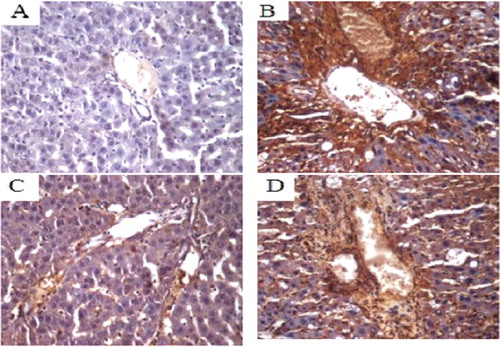
Table 3. COX-2 protein expression in liver tissue (x ± S.D.).
Pathological changes in rat liver tissue
There was no staining of lipid droplets in the normal control group, and the background was blue. In the other groups, there were different degrees of fatty degeneration; lipid droplets formed in liver cells, and the background changed from blue to red. Compared with the model group and the empty vector group, there was a significantly lower number of lipid droplets in the liver tissues of the COX-2 shRNA-1 group and the normal control group ().
Figure 7. Changes in lipid droplets in the liver tissues of the SD rat groups (Sudan IV staining, 400×). Normal control group (A); model group (B); COX-2 shRNA-1 group (C); empty vector group (D).
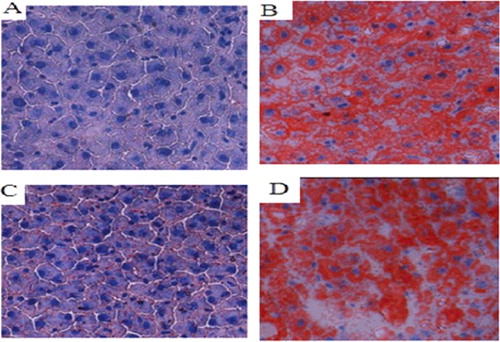
In all groups but in the normal control, HE staining revealed different degrees of edema, hepatocyte steatosis, inflammatory cell infiltration and hyperplasia of collagen fibers in liver tissue. Compared with the model group and the empty vector group, the degrees of liver fibrous hyperplasia in the COX-2 shRNA-1 group and normal control group were significantly lower, with small amounts of liver steatosis, small amounts of fibrous tissue, fibrous tissue hyperplasia and complete lobular architecture ().
Figure 8. Liver tissues of each SD rat group (HE staining 200×). Normal control group (A); model group (B); COX-2 shRNA-1 group (C); empty vector group (D).
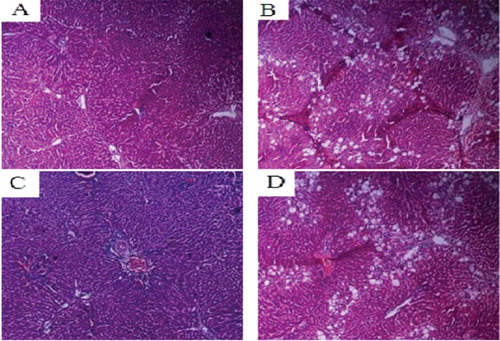
In addition to HE staining, we also used Masson staining to analyze the damage severity of liver fibrosis. Compared with the normal control group, the other groups all had different degrees of collagen fiber hyperplasia, and even false flocculus. Compared with the model group and the empty vector group, the collagen fiber hyperplasia in the COX-2 shRNA-1 group was reduced, and false flocculus was not formed ().
Degree of liver fibrosis score and collagen area analysis
Compared with the normal control group, the other groups exhibited increased liver fibrosis of different degrees and increased collagen areas of different amounts, with significant differences (P < 0.01). Compared with the normal control group, the degree of liver fibrosis in the COX- 2 shRNA-1 group was relatively high, and the values in the model group and the empty vector group were lower ().
Table 4. Degree of liver fibrosis score and collagen area analysis (x ± S.D.).
As a new treatment method, RNAi technology has gradually become the hot spot of research [Citation5]. Our study is the first to report that COX-2 shRNA1 could be used to treat experimental hepatic fibrosis. We found that the severity of hepatic fibrosis was alleviated and the liver texture became softer in the treated SD rats. It is suggested that the anti-fibrosis function of COX-2 shRNA might bring new hope for future therapy.
Conclusions
This study demonstrated the successful construction of COX-2 shRNA eukaryotic expression vectors. COX-2 shRNA-1 inhibited the expression of COX-2 in liver tissue and ameliorated liver fibrosis in SD rats. Our findings may lead to new therapeutic methods in the research on COX-2 gene and anti-fibrosis activities.
supp_data_1431569.pdf
Download PDF (562.2 KB)Acknowledgements
We are grateful to physicians and technicians who contribute to this study.
Disclosure statement
The authors declare no conflicts of interest.
Additional information
Funding
References
- Motino O, Agra N, Brea Contreras R, et al. Cyclooxygenase-2 expression in hepatocytes attenuates non- alcoholic steatohepatitis and liver fibrosis in mice. Biochim Biophys Acta. 2016;1862:1710–1723.
- Chang HK, Chang EY, Ryu S, et al. Cyclooxygenase-2 inhibitor reduces hepatic stiffness in pediatric chronic liver disease patients following kasai portoenterostomy. Yonsei Med J. 2016;57:893–899.
- Kim J, Lee J, Shin CM, et al. Risk of gastrointestinal bleeding and cardiovascular events due to NSAIDs in the diabetic elderly population. BMJ Open Diabetes Res Care. 2015;3:e000133.
- Nie QH, Zhu CL, Zhang YF, et al. Inhibitory effect of antisense oligonucleotide targeting timp-2 on immune-induced liver fibrosis. Dig Dis Sci. 2010;55:1286–1295.
- Robinson PM, Blalock TD, Yuan R, et al. Hammerhead ribozyme-mediated knockdown of mRNA for fibrotic growth factors: transforming growth factor-beta 1 and connective tissue growth factor. Methods Mol Biol. 2012;820:117–132.
- Gonzalez-Rodriguez A, Valverde AM. RNA interference as a therapeutic strategy for the treatment of liver diseases. Curr Pharm Des. 2015;21:4574–4586.
- Lam JK, Chow MY, Zhang Y, et al. siRNA versus miRNA as therapeutics for gene silencing. Mol Ther Nucleic Acids. 2015;4:e252.
- Deng Y, Wang CC, Choy KW, et al. Therapeutic potentials of gene silencing by RNA interference: principles, challenges, and new strategies. Gene. 2014;538:217–227.
- Presloid JB, Novella IS. RNA viruses and RNAi: quasispecies implications for viral escape. Viruses. 2015;7:3226–3240.
- Kola VS, Renuka P, Madhav MS, et al. Key enzymes and proteins of crop insects as candidate for rnai based gene silencing. Front Physiol. 2015;6:119.
- Mansoori B, Sandoghchian Shotor-bani S, Baradaran B. RNA interference and its role in cancer therapy. Adv Pharm Bull. 2014;4:313–321.
- Dyawanapelly S, Ghodke SB, Vishwanathan R, et al. RNA interference-based therapeutics: molecular platforms for infectious diseases. J Biomed Nanotechnol. 2014;10:1998–2037.
- Sioud M. RNA interference: mechanisms, technical challenges, and therapeutic opportunities. Methods Mol Biol. 2015;1218:1–15.
- Ipsaro JJ, Joshua-Tor L. From guide to target: molecular insights into eukaryotic RNA-interference machinery. Nat Struct Mol Biol. 2015;22:20–28.
- Borel F, Kay MA, Mueller C. Recombinant AAV as a platform for translating the therapeutic potential of RNA interference. Mol Ther. 2014;22:692–701.
- Holen T, Amarzguioui M, Wiiger MT, et al. Positional effects of short interfering RNAs targeting the human coagulation trigger tissue factor. Nucleic Acids Res. 2002;30:1757–1766.
- Yamada T, Peng CG, Matsuda S, et al. Versatile site-specific conjugation of small molecules to siRNA using click chemistry. J Org Chem. 2011;76:1198–1211.
- El-Sagheer AH, Brown T. New strategy for the synthesis of chemically modified RNA constructs exemplified by hairpin and hammerhead ribozymes. Proc Natl Acad Sci USA. 2010;107:15329–15334.
- Li G, Li XP, Jiang L, et al. [Silencing of cox-2 in nasopharyngeal carcinoma cells with a shRNAmir lentivirus vector]. Nan Fang Yi Ke Da Xue Xue Bao. 2009;29:1111–1114. Chinese.
- Yu Y, Zhang S, Kong W. [Construction and identification of eukaryotic plasmid pGC-silencer-U6/Neo/GFP/ABCG2]. Lin Chung Er Bi Yan Hou Tou Jing Wai Ke Za Zhi. 2010;24:795–798. Chinese.
- Zhang MX, Wu J, Xin M, et al. Construction and identification of eukaryotic expression plasmid expressing siRNA targeting Rac1 and detection of its specific downregulation effect. Sichuan Da Xue Xue Bao Yi Xue Ban. 2009;40:969–972.
- Zhou X, Yang XF. Progress of targeting transforming growth factor-β1 small interfering RNA in liver fibrosis. Chin Med Sci J. 2014;29(4):231–235.
- Dow LE, Premsrirut PK, Zuber J, et al. A pipeline for the generation of shRNA transgenic mice. Nat Protoc. 2012;7:374–393.
- Coward WR, Watts K, Feghali-Bostwick CA, et al. Defective histone acetylation is responsible for the diminished expression of cyclooxygenase 2 in idiopathic pulmonary fibrosis. Mol Cell Biol. 2009;29:4325–4339.
- Pang L, Nie M, Corbett L, et al. Protein kinase C-ϵ mediates bradykinin-induced cyclooxygenase-2 expression in human airway smooth muscle cells. FASEB J. 2002;16:1435–1437.