ABSTRACT
In this study, we investigated effects of 3,3′-diindolylmethane (DIM) on alveolar bone resorption in mice with experimentally induced periodontitis. Experimental periodontitis was induced by placing 5.0 silk sutures around the maxillary left second molar for 1 week. Fifty-three SPF, 6–8-week-old, female C57 mice were randomly divided into four groups: (1) healthy mice (control group), (2) mice with experimentally induced periodontitis (Ped group), (3) mice with periodontitis treated with corn oil (OIL-Ped group) and (4) mice with periodontitis treated with DIM dissolved in corn oil (DIM-Ped group). Infiltration of inflammatory cells in the gingival tissue was assessed via histomorphometrical analysis, inflammatory factors were detected by reverse transcription quantitative polymerase chain reaction (RT-qPCR) and changes in the alveolar bone were detected by micro-computed tomography (micro-CT) after treatment for 1 and 4 weeks. After 1 week of treatment, compared with the Ped and OIL-Ped groups, the DIM-Ped group showed decreased infiltration of inflammatory cells, preservation of connective tissue in the papilla and less interproximal and interradicular bone resorption. In the DIM-Ped group, the expression of IL-1β and RANKL decreased significantly (P < 0.05). After 4 weeks of treatment, periodontal inflammation level and alveolar bone absorption were similar among groups. However, in the DIM-Ped group, the expression of IL-1β, IL-6 and RANK significantly decreased (P < 0.05). As a whole, DIM treatment for 1 week can benefit alveolar bone recovery by inhibiting the inflammation of the periodontal tissue and expression of bone resorption-related cytokines.
Introduction
Periodontitis, characterised by inflammatory alveolar bone resorption, is considered to be caused by complex interactions between periodontopathogenic bacteria and the host immune response [Citation1]. Thus, reasonable regulation of inflammatory factors is expected to be an effective treatment for periodontal diseases [Citation2]. 3,3′-Diindolylmethane (DIM) is an oligomer of acidised indole-3-carbinol (I3C) [Citation3]. Recently, it was found that DIM may have remarkable anti-inflammatory effects, including effective remission of experimental colitis [Citation3], encephalomyelitis [Citation4], dermatitis [Citation5] and osteoporosis symptoms in mice [Citation6] and significant down-regulation of the release of inflammation mediators in macrophages [Citation7] and dendritic cells [Citation8]. DIM alleviates steatosis and the progression of nonalcoholic steatohepatitis (NASH) partly by shifting the imbalance of Treg/Th17 cells to Treg dominance, which plays an important role in periodontitis recovery [Citation9]. Furthermore, the regulatory effect of DIM on the RANKL/RANK pathway may offer a new treatment strategy for periodontitis [Citation7].
Given these anti-inflammatory effects, the aim of the present study was to analyse the therapeutic effects of DIM on periodontal inflammation and alveolar bone resorption for the first time.
Materials and methods
Animals
Fifty-three female mice (Mus rattus, C57BL6, 6–8 weeks old and weighing 19–23 g) were randomly assigned to four groups. Periodontitis was experimentally induced in all mice except for those in the control group (n = 5). The remaining groups included an experimental periodontitis group (Ped, n = 16), mice with periodontitis treated with corn oil (Sigma-Aldrich Corporation, St Louis, MO, USA) (OIL-Ped, n = 16) and mice with periodontitis treated with DIM (Sigma-Aldrich Corporation) dissolved in corn oil (DIM-Ped, n = 16). The study protocol was approved by the Institutional Ethics Committee of the Guangzhou Institute of Oral Disease, Stomatology Hospital of Guangzhou Medical University, followed all recommendations of the Animal Research: Reporting In Vivo Experiments (ARRIVE) guidelines for the execution and submission of studies in animals [Citation10] and was conducted in accordance with the recommendations of the National Council for Control of Animal Experimentation. Pairs of mice were housed in cages in a room under a 12-h daylight/darkness cycle at an ambient temperature of 23 °C ± 2 °C with 55% ± 10% humidity.
Model for experimental periodontitis
The mice were anaesthetised using an intraperitoneal injection of sodium pentobarbital (Sigma-Aldrich Corporation) (40 mg/kg body weight). To induce bone loss, a 5-0 silk ligature (JinHuan Medical Co., Ltd., Pudong, Shanghai, China) was ligatured around the maxillary left second molar for 1 week, as described in a previous study [Citation11]. The contralateral molar in each mouse was left unligated to serve as a baseline control for bone height measurements.
Drug treatments
After removing the ligatures, OIL-Ped and DIM-Ped groups were exposed to different treatment procedures. The OIL-Ped group was intraperitoneally injected with 100 μL of corn oil containing 2% dimethyl sulphoxide (DMSO; Sigma-Aldrich Corporation) from the day the ligatures were removed, twice a week until the mice were sacrificed. The DIM-Ped group was intraperitoneally injected with 100 μL of DIM dissolved in corn oil (DMSO, 2%) at a dose of 0. 1 mg/g, as described in a previous study [Citation6], twice a week until the mice were sacrificed.
Animal sacrifice and analyses
At the end of the periodontal treatment period (1 and 4 weeks), half of the mice in each group were sacrificed by neck fracture. The palatal gingival tissues of the lesions were excised and total RNA was extracted for reverse transcription quantitative polymerase chain reaction (RT-qPCR). After dissection of the gingival tissues, block samples were fixed in 4% paraformaldehyde (Beijing Leagene Biotech. Co., Ltd., Haidian District, Beijing, China) for 24 h and stored in 70% ethanol (Fu Yu Chemical Co., Ltd., Hangzhou City, Zhejiang Province, China) until used to evaluate alveolar bone resorption via micro-computed tomography (micro-CT) (SCANCO Medical AG, Brüttisellen, Switzerland) [Citation12]. After micro-CT, the samples were submitted to routine processing for descriptive histological analysis.
Histological analyses
The samples for histological analysis were decalcified in a solution containing 10% ethylenediaminetetraacetic acid (EDTA; Beijing Leagene Biotech. Co., Ltd.) (0.5 mol/L, pH 8.0) for 4–5 weeks (with × 3 solution changes per week) and then embedded in paraffin. Serial sections of 4-mm thickness were obtained in the mesiodistal direction, mounted on slides and stained with haematoxylin and eosin (H&E; Beijing Leagene Biotech. Co., Ltd.). Bone resorption and inflammation are typically assessed in the area between the first and second molar from the cemento-enamel junction to the alveolar bone crest [Citation13,Citation14]. Six fields (100 × 100 μm) from each section were randomly selected for histopathological analysis. The total number of inflammatory cells from the six areas, mainly polymorphonuclear leukocytes, represented the inflammatory status [Citation15]. A masked and calibrated examiner (CRA) blinded to the groups assessed the extent of inflammation in the periodontal tissues.
RT-qPCR assessment of inflammatory cytokines changes
Total RNA from the gingival palatal tissues between the distal aspect of the first molar and mesial aspect of the third molar was extracted using the RNAprep pure kit [DP420; TIANGEN Biotech (Beijing) Co., Ltd., Haidian District, Beijing, China]. Complementary DNA (cDNA) was synthesised by RT of total RNA using a reverse transcription kit (RR047A; Takara, Dalian, Liaoning Province, China). qPCR was performed using a qRT-PCRSystem (CFX96 Touch™ Real-time PCR System; Bio-Rad; Hercules, California, USA). Each 25-μL PCR reaction mix included 2 μL of the RT reaction product, nuclease-free water, master mix (premix Ex Taq 420A; Takara, Dalian, Liaoning Province, China) and forward and reverse primers for mouse cytokine genes (Genecopoeia, Rockville, MD, USA) (). The cycling conditions used for all primers were preoptimised at 95 °C for 30 s and 40 cycles of 95 °C for 5 s and 60 °C for 30 s. Determination of the relative gene expression levels was performed using the cycle threshold method and normalised to the housekeeping gene encoding glyceraldehyde 3-phosphate dehydrogenase (GAPDH). The results are presented as the mean mRNA expression level from duplicate measurements normalised to that of the internal control GAPDH and expressed as the fold change compared with levels determined in cDNA samples prepared from healthy control gingival tissues.
Table 1. Inventoried primers for target genes.
Micro-CT measurement of alveolar bone loss
After sacrificing the animals, the maxilla samples from each group were carefully harvested, fixed in 4% paraformaldehyde for 24 h and stored in 70% ethanol at room temperature until scanned using a micro-CT system. The X-ray generator was operated at 59 kV and a beam current at 100 μA with a 0.5-mm aluminium filter at an image resolution of 9.93 μm. The images were reconstructed using Data Viewer v.1.4.3.1 (SkyScan; Kartuizersweg, Kontich, Belgium) in all three spatial dimensions, and then all the images were orientated and saved in sagittal slices (2000·2000). Data of ROI were analysed using a CT-Analyser v1.10.1.0 (SkyScan; Kartuizersweg, Kontich, Belgium), as described by Zhang et al. [Citation16]. The parameters analysed for each sample included bone volume fraction (BVF), trabecular number (Tb. N), trabecular bone pattern factor (Tb. Pf), trabecular thickness (Tb. Th), trabecular separation (Tb. Sp) and structure model index (SMI). Measurements of linear bone loss were made along the axis of each root surface of all molar teeth. The total alveolar bone loss was obtained by subtracting the values of the right maxilla (unligated control) from the sum of the recordings from the buccal tooth surfaces, in millimetres (mm) [Citation17].
Data analysis
Statistical analysis was performed using GraphPad Prism software (GraphPad Software, Inc., San Diego, CA, USA). The results obtained for all parameters among the different groups were comparatively evaluated in each period using one-way analysis of variance (ANOVA) and the unpaired t-test. A probability (P) value of < 0.05 was considered statistically significant.
Results and discussion
Histological analysis
The results from the descriptive histological analysis between the first and second molars for all groups in every period are presented in . The molars of the control group had normal morphology ((A)). However, the mice subjected to ligature-induced periodontitis experienced accentuation of inflammatory cells ((I)), fibre disorganisation, cemento-enamel attachment loss, apical migration of the junctional epithelium, cementum demineralisation and severe bone resorption ((B, C, D, F, G and H)). After 1 week of treatment, compared with the other groups, the reduction in the inflammatory infiltrate was more pronounced in the DIM-Ped group (P < 0.01) ((I)), coupled with preserved collagen fibres, remineralisation of the cementum (the arrow shows regenerated periodontal fibres) and a significant decrease in bone loss ((B, C and D)). After 4 weeks of treatment, gingival inflammation and alveolar bone loss were markedly decreased in all ligatured groups ((B–F, C–G and D–H)). Nevertheless, the DIM-Ped group showed morphological characteristics very similar to those of the other groups ((F, G, H and I)).
Figure 1. Histological views (H&E stain, magnification × 100) of the sections of the distal root aspect of the first maxillary molars in the control, Ped, OIL-Ped and DIM-Ped groups at 1 week (A, B, C and D) and at 4 weeks (E, F, G and H). Statistical analysis for periodontal tissue inflammation (I).
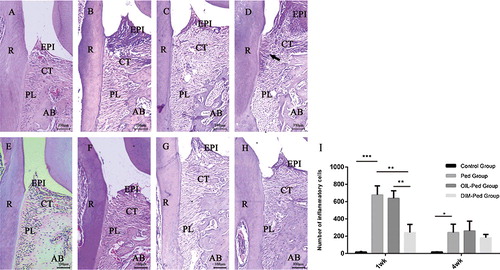
Quantitative analyses of mRNA expression of inflammatory cytokines
After 1 week of treatment, mRNA expression of RANK, RANKL, IL-1β and IL-6 was more pronounced in the ligature model. In the DIM-Ped group, the expression levels of IL-1β and RANKL were significantly decreased (P < 0.05). After 4 weeks of treatment, the expression levels of RANK, RANKL, IL-1β and IL-6 tended to increase in the ligature model. In the DIM-Ped group, the expression levels of RANK, IL-1β and IL-6 decreased significantly (P < 0.05). However, there were no significant differences among the other ligatured groups at each time point ().
Micro-CT three-dimensional (3D) and two-dimensional (2D) observations
The 2D and 3D sagittal micro-CT views of each group showed obvious alveolar bone loss in the ligature models after 1 week of treatment. There was less alveolar bone resorption in the DIM-Ped group than in the Ped and OIL-Ped groups. After 4 weeks of treatment, the alveolar bone loss in the ligature models became mild, while there was less alveolar bone resorption in the DIM-Ped group than in the other two groups ().
Figure 3. Bidimensional sagittal micro-CT views of the maxillary molars of different animals from each group at 1 (A–D) and 4 weeks (E–H). Three-dimensional sagittal micro-CT views of the maxillary molars of different animals from each group at 1 (I–L) and 4 weeks (M–P). Control group (A, E, I and M); Ped group (B, F, J and N); OIL-Ped group (C, G, K and O) and DIM-Ped group (D, H, L and P).
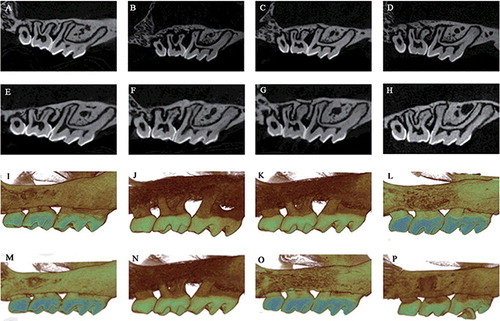
Linear alveolar bone loss
After 1 week of treatment, linear alveolar bone loss was lower in the DIM-Ped group than in the Ped group (P < 0.05), while there was no difference between the Ped and OIL-Ped groups (P > 0.05). After 4 weeks of treatment, there was no difference between the experimental groups (P > 0.05) ().
Volumetric alveolar bone loss
After 1 week of treatment, BVF and Tb. N of the ligature model decreased compared with those of the control group (P < 0.05). Among the ligature model groups, these parameters decreased significantly in the DIM-Ped group (P < 0.05), whereas there was no difference between the Ped and OIL-Ped groups (P > 0.05). After 4 weeks of treatment, there was no difference in BVF and Tb. N between the experimental groups (P > 0.05). Lastly, there were no significant differences in Tb. Pf, Tb. Th, Tb. Sp and SMI among the groups at all time points (P > 0.05) ().
Figure 5. Comparison of micro-CT bone micro parameters measured in a selected ROI of the maxillary first molars in all experimental groups at 1 and 4 weeks. BVF, bone volume fraction; Tb. Th, trabecular thickness; Tb. Sp, trabecular separation; Tb. N, trabecular number; Tb. Pf, trabecular bone pattern factor; SMI, structure model index.
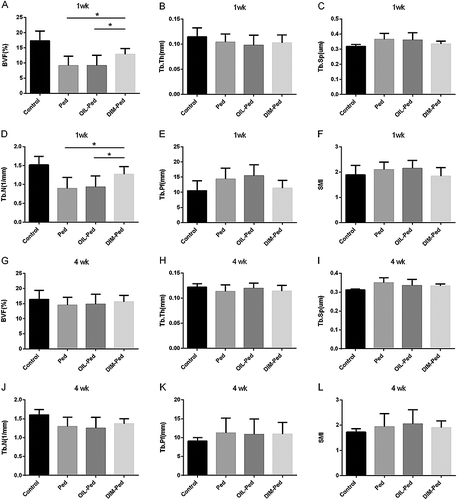
Putative mechanism of action and therapeutic potential
In the present study, a well-established model of ligature-induced periodontitis in mice was used, which is a reliable technique for disease induction [Citation12], that is frequently used to identify pathogenic factors and determine the efficacy of drugs [Citation18,Citation19]. Analysis was performed by histological staining and micro-CT. Neutrophil recruitment is critical to cause periodontal tissue damage by stimulating local inflammatory mediator production and activating osteoclastogenesis in a RANKL-dependent manner [Citation20]. The histomorphometrical analysis showed significantly less infiltration of inflammatory cells in the DIM-Ped group compared with that in the Ped and OIL-Ped groups after 1 week of treatment, coupled with decreased periodontal ligament breakdown, cemento-enamel loss and alveolar resorption. Therefore, the anti-inflammatory effect of DIM was obvious. In addition, there was a significant reduction in alveolar bone loss by DIM. Further analysis of volumetric alveolar bone parameters confirmed the protective effect of DIM, as BVF and Tb. N increased significantly. Although there were no significant differences among the other parameters, Tb. Th tended to increase, whereas SMI, Tb. Pf and Tb. Sp tended to decrease. These results indicated that the use of DIM reduced alveolar bone loss in 1 week. After 4 weeks of treatment, gingival inflammation and alveolar bone loss were relieved to a similar extent in all ligatured groups, which indicated marked self-repair in 4 weeks, masking the efficacy of DIM.
The effect of DIM on periodontal connective tissues and alveolar bone suggests that DIM is an effective agent for periodontitis treatment, which can partly be explained by its effect on RANK, RANKL, OPG, IL-6 and IL-1β in the gingival tissue [Citation12]. RANKL plays a crucial role in the differentiation and activation of osteoclasts by binding to its cognate receptor RANK, whereas OPG can bind to RANKL competitively, thereby inhibiting osteoclast function [Citation21]. Crotti et al. [Citation22] reported an increased RANKL/OPG ratio in periodontitis. Liu et al. [Citation23] further pointed out that the more serious the periodontal symptoms are, the higher the RANKL/OPG ratio is. Inhibiting the expression of RANKL in T cells could significantly decrease alveolar bone resorption [Citation24]. Thus, alveolar bone resorption is likely to be inhibited if the expression of RANKL can be suppressed.
Related research on the impact of DIM on RANKL found that DIM suppressed PGE2-stimulated expression of RANKL in fibroblast-like cells and osteoblasts [Citation25]. In vivo, DIM increases the bone mass by suppressing osteoclastic bone resorption in ovariectomy-induced osteoporotic mice [Citation6]. In the present study, mice treated with DIM for 1 week also had a significant inhibitory effect on the high expression of RANKL in the gums, whereas DIM treatment had a weak effect on the expression of OPG at 1 and 4 weeks, which showed that the main factors affecting the OPG content in mice were autoimmune regulation and body repair. Taken together, these results show that DIM can reduce the RANKL/OPG ratio, thereby inhibiting the alveolar bone absorption. After 4 weeks of treatment, the expression of RANK was significantly decreased in the DIM-Ped group. When both RANK and RANKL are significantly inhibited by DIM, the loss of alveolar bone can be relieved.
Among various inflammatory cytokines, IL-1β and IL-6 play important roles in the expression of differentiation factors and activation of osteoclasts, which results in CT degradation and bone resorption [Citation26]. A previous study reported that DIM can reduce the expression of IL-1β and IL-6 to delay prostate tumour growth and angiogenesis [Citation27]. In vitro, the effects of DIM on IL-1β and IL-6 were consistent [Citation7,Citation8]. In line with these previous results, in the present study, the expression levels of IL-1β and IL-6 in the gingiva tissue were reduced in the DIM-Ped group at 1 and 4 weeks. The observed reduction in IL-1β further confirms the reduction of leukocyte migration in the treated mice because IL-1β can induce the regulation of adhesion molecules on leukocytes and endothelial cells and stimulate the production of chemokines necessary to recruit circulating leukocytes [Citation12]. The downregulation of IL-1β and IL-6 might be one of the possible anti-inflammatory activities of DIM in periodontitis.
In terms of clinical importance, compared with most preventive experiments for ligature-induced periodontitis, our therapeutic experiment is more convincing because it is closer to the clinical treatment of periodontitis. Our study first demonstrated that DIM can effectively inhibit alveolar bone absorption after 1 week of treatment, furthering the research regarding periodontitis drug therapy. Thus, individuals with periodontitis should be advised to eat cruciferous vegetables that contain I3C to support periodontal disease treatment. However, the optimal dosage, mode of administration and duration of use, along with the regulatory role of DIM in the RANKL/RANK pathway and its upstream and downstream regulation in vitro and in vivo, require further analysis.
Conclusions
To the best of the our knowledge, this study is the first to reveal that DIM can inhibit inflammation of the periodontal tissue and expression of inflammatory factors IL-1β, IL-6 and RANKL in 1 week, which promoted alveolar bone recovery in the experimental periodontitis mouse model. These results suggest that DIM may be a potential host-modulatory agent in adjunctive therapy for periodontal diseases.
Acknowledgements
We are grateful for the technical support from Guangzhou Medical University-Affiliated Stomatology Hospital.
Disclosure statement
The authors report no conflicts of interest related to this study.
Additional information
Funding
References
- Kara A, Akman S, Ozkanlar S, et al. Immune modulatory and antioxidant effects of melatonin in experimental periodontitis in rats. Free Radic Biol Med. 2013;55(1):21–26.
- Morand DN, Davideau JL, Clauss F, et al. Cytokines during periodontal wound healing: potential application for new therapeutic approach. Oral Dis. 2017;23(3):300–311.
- Kim YH, Kwon HS, Kim DH, et al. 3,3′-diindolylmethane attenuates colonic inflammation and tumorigenesis in mice. Inflammatory Bowel Dis. 2009;15(8):1164–1173.
- Rouse M, Rao R, Nagarkatti M, et al. 3,3′-diindolylmethane ameliorates experimental autoimmune encephalomyelitis by promoting cell cycle arrest and apoptosis in activated T cells through microRNA signaling pathways. J Pharmacol Exp Ther. 2014;350(2):341–352.
- Kim EJ, Park H, Kim J, et al. 3,3′-diindolylmethane suppresses 12-O-tetradecanoylphorbol-13-acetate-induced inflammation and tumor promotion in mouse skin via the downregulation of inflammatory mediators. Mol Carcinog. 2010;49(7):672–683.
- Yu TY, Pang WJ, Yang GS. 3,3′-Diindolylmethane increases bone mass by suppressing osteoclastic bone resorption in mice. J Pharmacol Sci. 2015;127(1):75–82.
- Xue L, Pestka JJ, Li M, et al. 3,3′-Diindolylmethane stimulates murine immune function in vitro and in vivo. J Nutr Biochem. 2008;19(5):336–344.
- Benson JM, Shepherd DM. Dietary ligands of the aryl hydrocarbon receptor induce anti-inflammatory and immunoregulatory effects on murine dendritic cells. Toxicol Sci. 2011;124(2):327–338.
- Liu Y, She W, Wang F, et al. 3,3′-diindolylmethane alleviates steatosis and the progression of NASH partly through shifting the imbalance of Treg/Th17 cells to Treg dominance. Int Immunopharmacol. 2014;23(2):489–498.
- Kilkenny C, Browne WJ, Cuthill IC, et al. Improving bioscience research reporting: the ARRIVE guidelines for reporting animal research. Osteoarthr Cartil. 2012;20(4):256–260.
- Abe T, Hajishengallis G. Optimization of the ligature-induced periodontitis model in mice. J Immunol Methods. 2013;394(1-2):49–54.
- de Molon RS, de Avila ED, Boas Nogueira AV, et al. Evaluation of the host response in various models of induced periodontal disease in mice. J Periodontol. 2014;85(3):465–477.
- Bainbridge B, Verma RK, Eastman C, et al. Role of porphyromonas gingivalis phosphoserine phosphatase enzyme SerB in Inflammation, immune response, and induction of alveolar bone resorption in rats. Infect Immun. 2010;78(11):4560–4569.
- Leitão RF, Ribeiro RA, Chaves HV, et al. Nitric oxide synthase inhibition prevents alveolar bone resorption in experimental periodontitis in rats. J Periodontol. 2005;76(6):956–963.
- Dong Y, Huihui Z, Li C. Piperine inhibit inflammation, alveolar bone loss and collagen fibers breakdown in a rat periodontitis model. J Periodont Res. 2015;50(6):758–765.
- Zhang W, Ju J, Rigney T, et al. Porphyromonas gingivalis infection increases osteoclastic bone resorption and osteoblastic bone formation in a periodontitis mouse model. BMC Oral Health. 2014;14(1):89–97.
- Carvalho RS. Effect of venlafaxine on bone loss associated with ligature-induced periodontitis in Wistar rats. J Negat Results Biomed. 2010;9(1):1–8.
- Arabaci T, Kermen E, zkanlar S, et al. Therapeutic effects of melatonin on alveolar bone resorption after experimental periodontitis in rats. A Biochemical and Immunohistochemical Study. J Periodontol. 2015;86(7):874–881.
- Li CH, Amar S. Morphometric, histomorphometric, and microcomputed tomographic analysis of periodontal inflammatory lesions in a murine model. J Periodontol. 2007;78(6):1120–1128.
- Chakravarti A, Raquil MA, Tessier P, et al. Surface RANKL of Toll-like receptor 4-stimulated human neutrophils activates osteoclastic bone resorption. Blood. 2009;114(8):1633–1644.
- Yamashita T, Takahashi N, Udagawa N. New roles of osteoblasts involved in osteoclast differentiation. World J Orthop. 2012;3(11):175–181.
- Crotti T, Smith MD, Hirsch R, et al. Receptor activator NF κB ligand (RANKL) and osteoprotegerin (OPG) protein expression in periodontitis. J Periodontal Res. 2003;38(4):380–387.
- Liu D, Xu JK, Figliomeni L, et al. Expression of RANKL and OPG mRNA in periodontal disease: possible involvement in bone destruction. Int J Mol Med. 2003;11(1):17–21.
- Mahamed DA, Marleau A, Alnaeeli M, et al. G(−) anaerobes-reactive CD4+ T-cells trigger RANKL-mediated enhanced alveolar bone loss in diabetic NOD mice. Diabetes. 2005;54(54):1477–1486.
- Dong L, Xia S, Gao F, et al. 3,3′-Diindolylmethane attenuates experimental arthritis and osteoclastogenesis. Biochem Pharmacol. 2010;79(79):715–721.
- Cochran DL. Inflammation and bone loss in periodontal disease. J Periodontol. 2008;79(8 Suppl):1569–1576.
- Kim EK, Kim YS, Milner JA, et al. Indole-3-carbinol and 3,3′-diindolylmethane modulate androgen's effect on C-C chemokine ligand 2 and monocyte attraction to prostate cancer cells. Cancer Prev Res. 2013;6(6):519–529.