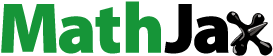
ABSTRACT
Here we report the synthesis, characterization and biological properties of silver nanoparticles (AgNPs) using the extract from a spice blend. AgNPs were prepared using 0.1 mmol/L AgNO3 solution and aqueous extract from a spice blend of garlic, ginger and cayenne pepper. The nanoparticles were characterized using ultraviolet–visible (UV-vis) spectroscopy, scanning and transmission electron microscopy (SEM and TEM), energy dispersive X-ray (EDX), X-ray diffraction (XRD) and Fourier transform infrared spectroscopy (FTIR). The AgNPs formed within an hour of the reaction with colour change from yellow to dark brown and maximum absorbance at 420 nm in the UV-vis spectrum. SEM revealed well dispersed particles with little agglomeration, and the presence of elemental silver was confirmed by EDX. TEM confirmed the formation of poly-dispersed, spherical-shaped NPs with size range of 6–28 nm. FTIR showed that amine, protein, phenolic, aromatic and alkyne groups contributed to AgNPs synthesis, and XRD confirmed their crystalline and face-centred cubic nature. Both Gram-negative and Gram-positive bacteria were susceptible to the spice blend AgNPs with MIC of 0.0625 at 62.5 µg/mL. The AgNPs exhibited greater inhibition on DPPH radical with IC50 < 31.25 µg/mL, while it was 68.75 µg/mL for ABTS. The nanoparticles were not toxic against MDA-MB-231 breast cancer cell lines. These spice blend nanoparticles would support the production of AgNPs with significantly enhanced pharmacokinetic properties and greatly improved therapeutic performance.
Introduction
Synthesis and characterization of nanomaterials is presently an important subject of research. Nanotechnology holds promising application in bio-sensing, biomedical, food, feed and drug delivery, cosmetics and cancer therapy, and many more fields [Citation1]. Silver nanoparticles can and have been synthesized using a variety of chemical and physical methods, involving chemical, photochemical, heat, electrochemical and photochemical reduction [Citation2–5]. Although physical and chemical methods are more popular and widely used for synthesis of nanoparticles, these processes involve the use of several toxic chemicals as reducing agents, leading to environmental toxicity and non-biodegradable products. Thus, there is the urgent need to develop eco-friendly biosynthetic processes that reduce the use of toxic chemicals. Therefore, nanoparticles synthesized from extracts of medicinal plants are currently of great interest. According to Salam et al. [Citation6] this interest is attributed to their wide range of applications, easy synthesis, economic prospects and environmental friendliness.
Nowadays, many materials from medicinal plants, fruits, weeds, mushrooms, herbs and spice, as well as herbal remedies are being used for the synthesis of nanoparticles [Citation7–10].
As reviewed by Viuda-Martos et al. [Citation11], spices and aromatic herbs have been used since antiquity as preservatives, colorants, flavour enhancers and home cures; and have long been the basis of traditional medicine in many countries. Spices have become the subject of study, particularly by the chemical, pharmaceutical and food industries because of their potential use for improving health [Citation11].
Besides its culinary use as a spice, our studies have shown that the spice blend of garlic (Allium sativum), ginger (Zingiber officinale) and cayenne pepper (Capsicum frutescens) has high polyphenolic and other reducing groups, possesses strong antioxidant, hypolipidemic anti-diabetic properties and is not toxic under repeated intake in rats [Citation12–15]. The presence of phenols and other reducing groups as well as the functional properties make the spice blend an ideal platform for biosynthesis of nanoparticles. In this study, to exploit all these beneficial properties together with the advantages of nanotechnology, silver nanoparticles were synthesized from the spice blend. The nanoparticles synthesized were then characterized using appropriate instrumentation and evaluated for their antimicrobial, antioxidant and cytotoxic activities.
Materials and methods
Materials
The fresh spices were purchased from the vegetable market in Alice, Eastern Cape, South Africa. The spices were identified and authenticated with the previously collected herbarium specimens available. The individual spices were cleaned, sliced, dried at 60 °C for 72 h and then pulverised. Equal amount of each spice was weighed (1:1:1, w/w/w) and mixed thoroughly by passing through a coffee grinder to give the spice blend. The spice blend was stored in air-tight bottles at 4 ˚C till needed. Silver nitrate (Sigma–Aldrich, USA) and all other reagents used in this study were of analytical grade. Deionised water was used throughout the experiment.
Preparation of silver nanoparticles
Twenty grams of the spice blend were weighed into a 250 mL conical flask and extracted with 100 mL of deionised water at 60 °C for 10 min in a water bath. The extract was cooled and filtered using Whatman filter paper no. 1 under vacuum. Exactly 15 mL of the prepared extract was added to 45 mL of aqueous AgNO3 (0.1 mol/L solution) at room temperature and stirred continuously with a magnetic stirrer for 15 min. The solution obtained was kept in the dark to prevent auto-oxidation of silver.
After 24 h, the solution containing silver nanoparticles (AgNPs) was centrifuged at 3000 rpm (HERMLE Labortechnick GmbH Z306 Siemensstr 25 D-78564 Wehingen) for 10 min and the resulting pellets were dried in an oven at 100 °C for 24 h. The purified AgNPs were then characterized.
Characterization of synthesized AgNPs
The formation of AgNPs was monitored by visual assessment of the colour changes of the solutions. The reduction of silver was measured periodically at a wavelength range 300–700 nm using an ultraviolet–visible (UV-vis) spectrophotometer (UV-3000 PC, United Kingdom). The UV-vis spectra of AgNPs produced were plotted and recorded as a function of bioreduction time (15 min intervals) at room temperature at a resolution of 0.5 nm.
The shape and morphology of the nanoparticles were determined using scanning electron microscopy (SEM). Briefly, the sample was sonicated for 5 min to make a suspension of AgNPs in distilled water. A drop of the suspension was then placed on double-sided coated carbon stubs, allowed to dry and observed using SEM (JEOL-JSM-6390 LV) at a voltage of 15–20 kV at different magnifications.
Transmission electron microscopy (TEM) analysis of the nanoparticles was done using a transmission electron microscope (Zeiss LIBRA® 120). Pellets of centrifuged AgNPs were sonicated in methanol; a drop of the suspension was placed on a carbon coated copper grid and allowed to dry at room temperature. TEM analysis was performed on a transmission electron microscope operating at 80 kV.
Energy dispersive X-ray (EDX) analysis was also performed for the confirmation of elemental silver. Both SEM and EDX analyses were carried out on the same instrument (JEOL JSM-6390 LVA).
X-ray diffraction (XRD) measurement of the nanoparticles was performed on an x-ray diffractometer (Brucker D8 Advanced) operated at a voltage of 45 kV and a current of 30 mA equipped with a proportional counter Cu Kα radiation (λ = 1.5405 Ǻ, nickel filter).
Fourier transform infrared (FTIR) analysis was used to determine the possible biomolecules responsible for the reduction of silver ions to AgNPs. The sample was analyzed using a JASCO FT-IR 4100 spectrometer. Spectra were collected from 50 scans at a resolution of 4 cm−1 in the transmission mode of 4000–440 cm−1.
Antimicrobial assay
The antibacterial activity of the AgNPs from the spice blend was assessed against two Gram-negative and two Gram-positive bacterial strains. The organisms Streptococcus faecalis (ATCC: 29212), Bacillus cereus (ATCC: 10702), Escherichia coli (ATCC: 25922), and Shigella flexneri (KZN) were chosen primarily on the basis of their importance as opportunistic pathogens of humans and food deterioration and were obtained from the Microbiology Unit of the Medicinal Plants and Economic Development (MPED) Research Centre, Botany Department, University of Fort Hare.
Agar dilution method was used for evaluating the minimum inhibitory concentration (MIC) of the AgNPs against the bacterial strains. Briefly, Mueller–Hinton agar was prepared by autoclaving and allowed to cool to 55 °C. A stock solution of the AgNPs was prepared in dimethylsulfoxide (DMSO). The agar medium containing the nanoparticles at final concentrations of (0.015 625–0.25 mg/mL) was poured into Petri dishes, swirled gently until the agar began to set, and left overnight for solvent evaporation. Microorganisms were streaked in radial pattern on the agar plates; the inoculum of each test strain was standardized at 5 × 105 cfu/mL using McFarland standard as described by the National Committee for Clinical Laboratory Standards (NCCLS) [Citation16]. The plates were incubated under aerobic conditions at 37 °C and examined after 24 h. Each treatment was performed in triplicate and complete suppression of growth at a specific concentration of the nanoparticles was taken as the minimum inhibitory concentration (MIC). Ciprofloxacin was used as positive control in this experiment.
Antioxidant assay
The antioxidant activities of the AgNPs from the spice blend was evaluated using DPPH and ABTS assays.
2,2-Azino-bis (3-ethylbenzthiazoline-6-sulfonic acid (ABTS) radical scavenging assay
The modified method described by Re et al. [Citation17] using micro-titre well plates was adopted for the determination of ABTS-scavenging activity. Briefly, the stock solutions consisting of 7 mmol/L ABTS solution and 2.4 mmol/L potassium persulphate solution were prepared. The working solution was prepared by mixing the two stock solutions in equal proportions (1:1 v/v) and allowing them to react for 12 h at room temperature in the dark. The solution was then diluted by adding 1 mL ABTS+ solution with 60 mL of methanol until an absorbance of 0.708 ± 0.001 units at 734 nm was attained. Then, 100 µL of methanol was added into all the wells with the exception of second (B) and third (C) rows; rutin (200 µL) was added into the third row (C) and 200 µL of the nanoparticles (0.5 mg/mL) prepared in methanol were added in triplicates in the next rows. A two-fold serial dilution yielding concentrations of the AgNPs and standard ranging from 0.01 to 0.5 μg/mL were thus prepared in the wells. The AgNPs (100 μL) and the control were allowed to react with 100 μL of the ABTS+ solution and the absorbance was measured spectrophotometrically at 734 nm after 7 min. The ABTS+-scavenging capacity of the extract was then compared with that of the standards and the percentage inhibition was calculated as:Where Abscontrol is the absorbance of ABTS radical + methanol and Abssample is the absorption of the ABTS + sample (nanoparticles/standards).
1,1-diphenyl–2–picrylhydrazyl (DPPH) radical scavenging assay
To assess the scavenging ability on DPPH radicals, the method described by Zou et al. [Citation18] using micro-well titre plates was used. Briefly, a stock solution of 0.135 mmol/L DPPH radical was prepared in methanol. Then, 100 µL of methanol was added into all the wells with the exception of second (B) and third (C) rows; rutin (200 µL) was added into the third row and 200 µL of the nanoparticles (0.5 mg/mL) prepared in methanol were added in triplicates in the next rows. A two-fold serial dilution yielding concentrations of the AgNPs and standards ranging from 0.01 to 0.5 mg/mL were thus prepared in the wells. The reaction mixture was then vortexed thoroughly and left in the dark at room temperature for 30 min after which the absorbance was measured in a spectrophotometer at 517 nm. The DPPH radical scavenging ability of the nanoparticles was calculated as:Where Abscontrol is the absorbance of DPPH + methanol and Abssample is the absorbance of DPPH + sample (nanoparticles/standards).
Cell culture and cytotoxicity assay
MDA-MB-231 breast cancer cells (ATCC HTB-26) were maintained in culture in phenol-red free L-15 medium supplemented with 10% (v/v) heat-inactivated FCS, 1 mmol/L L-glutamine, 100 U/mL penicillin, 100 μg/mL streptomycin and 12.5 μg/mL amphotericin (PSA) at 37 °C in a humidified incubator. The cytotoxicity of the AgNPs was assessed using the MTT Cell Proliferation Assay (Roche). Briefly, cells were seeded at a density of 6000 cells per well into 96-well plates and incubated overnight, followed by treatment with a range of concentrations (0.16, 0.8, 4, 20, 100 and 500 μg/mL) of the AgNPs or DMSO vehicle control (0.02 % v/v DMSO) for 96 h. Thereafter, 2.5 µL of WST-1 Cell Proliferation Reagent was added per well and the absorbance at 450 nm after 8 h recorded using a Synergy Mx spectrophotometer (BioTek). The experiment was carried out in triplicate and the half maximal inhibitory concentration (IC50) was calculated from a dose response curve (log concentration vs absorbance at 450 nm) using non-linear regression with GraphPad Prism 4 (GraphPad Inc.).
Statistical analysis
Results were expressed as mean values with standard deviation (±SD) of three replicates and were subjected to analysis of variance (ANOVA) using Minitab release version 12, Windows 95. Significant levels were tested at P < 0.05.
Results and discussion
In the present study, AgNPs synthesized from a spice blend of garlic, ginger and cayenne pepper were characterized and their antibacterial and antioxidant activities and preliminary toxicity were investigated. Currently, many spices and herbal extracts have been used for the synthesis of silver, copper, iron and gold nanoparticles [Citation9,Citation19]. We used this spice blend of garlic, ginger and cayenne pepper for production of AgNPs because they contain high amount of polyphenols and other reducing groups with biological properties appropriate for the efficient production of AgNPs.
Synthesis and characterisation of AgNPs
Addition of the spice extract to aqueous silver nitrate solution resulted in changes of colour of the mixture from faint yellow to colloidal brown, indicating AgNP formation (Supplementary Figure S1). According to Nasiri and Nasiri [Citation20], the first indicator of bio-reduction of Ag ions to AgNPs is evidenced by the characteristic change in the colour of the plant extract. This occurs as a result of alteration in surface plasmon resonance (SPR) when positive ions (Ag+) are transformed into nano-forms [Citation21].
The UV-Vis spectrum of AgNPs () was recorded from the reaction medium as a function of time at intervals of 0, 15, 30, 45 and 60 min. The AgNPs from the spice blend gave absorbance peaks ranging between 375–420 nm. The intensity of the absorbance increased with time, indicating increase in the amount of AgNPs formed. The UV absorption spectrometric analysis showed a strong resonance centered at 420 nm at 60 min. The UV-vis spectra also revealed that the AgNPs formed rapidly within 60 min and remained stable even after 24 h.
Figure 1. Ultraviolet–visible (UV-vis) spectra of silver nanoparticles as a function of time at intervals of 15, 30, 45 and 60 min.
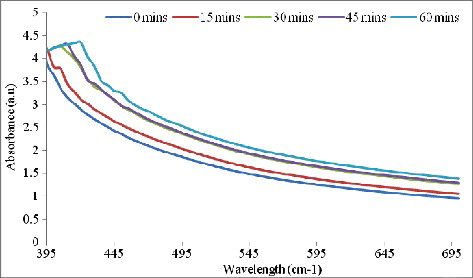
The characteristic peak at 420 nm observed in the UV-vis spectrum further confirms the biosynthesis of AgNPs. According to Singhal et al. [Citation22] and Selvi et al. [Citation23], the broad absorption band at 420 nm is typical of AgNPs and is attributed to excitation of surface plasmon resonance. This single surface Plasmon resonance peak indicates that the particles were spherical with a wide range of size distribution [Citation24]. In addition, SPR peak in the range of 410–450 nm has been reported by other authors as indicative of AgNP biosynthesis.
Scanning electron microscopy of AgNPs
SEM analysis of the AgNPs revealed the formation of highly crystalline well dispersed AgNPs (), which were spherical in shape, fairly agglomerated and of varying sizes. The present work is in agreement with earlier work done on silver nanoparticles synthesized from extracts of each of the spices that made up the spice blend, i.e garlic, ginger and cayenne pepper [Citation25]. The explanation for the agglomeration and varying sizes is again consistent with our earlier report [Citation25] in which we posited among other reasons that AgNPs have the tendency to agglomerate due to their high surface tension and energy.
Energy dispersive X-ray analysis of AgNPs
EDX further confirmed the formation of AgNPs from the spice blend, as there was strong signal in the silver region confirming the formation of AgNPs (). From the EDX spectra, the weight percentage of silver was 66.63%.
The EDX spectrum confirmed the synthesis of AgNPs and indicates that silver is the principal ingredient element. Metallic AgNPs generally show a typically strong signal peak at 3 keV, owing to surface plasmon resonance [Citation26]. Similar results have been reported earlier for AgNPs from garlic, ginger and cayenne pepper individually [Citation25] and with other plant extracts such as Artemisia nilagirica leaf, Artocarpus heterophyllus seed and Boerhaavia diffusa extracts [Citation27, 28 , 29Citation[28]].
Transmission electron microscopy analysis of AgNPs
TEM is often used to provide further insight into the morphology and particle size distribution profile of AgNPs. A representative TEM image of AgNPs of the spice blend is shown in . TEM revealed that the AgNPs have spherical shape with average size range of 6–28 nm. The data obtained showed distinct poly-dispersed, spherical-shaped NPs with small sizes without significant agglomeration. Such small size provides nanoparticles with large surface area, which subsequently enhances their reactivity and catalytic properties in various applications [Citation30]. Earlier TEM studies of NPs synthesized from garlic, ginger and cayenne pepper individually, showed similar TEM characteristics as observed in the present study [Citation25]. Similarities in the shapes of nanoparticles could be attributed to having the same type of bio-reductive and capping agents. Several studies have reported a wide range in the sizes (5–100 nm) of AgNPs and morphologies (spherical, nanorods, hexagonal, etc.) using various plant extracts (e.g. soluble starch, coffee and green tea, fungal spices and other biological extracts [Citation9,Citation18,Citation31–35], but none from a blend of spices which we now report in this study.
Fourier transform infrared microscopic analysis of AgNPs
Fourier Transform Infrared (FTIR) spectroscopic analysis identified the potential functional groups of bio-molecules in the spice blend extract responsible for reducing and capping the bio-reduced AgNPs. The FTIR analysis revealed different stretches of bonds at different peaks for each of the spices ().
The nanoparticles from the spice blend showed peaks at 3456, 3016, 2970, 2946 and 2574 cm−1 haracteristic of O–H alcohol stretch and N–H amide stretch; C–H stretch, O–H acid stretch and carboxylic acid OH with strong intensity. Other peaks at 2134, 1738, 1435, 1365, 1228, 1216, 1206 and 1092 were assigned to the C≡C (alkyne stretch), C꞊O carbonyl stretch, C≡C (aromatic stretch), N–O nitro stretch, C–O acid and ether stretch; while the peaks 899 and 775 were assigned to the C–H bending.
Ethylene groups (=C–H) capable of acting as reducing agents, have been detected by FTIR at around 1092 nm [Citation36]. The absorption peaks at 1737 and 2970 cm−1 point to a possible interaction between proteins and AgNPs; The peak at 1373 cm−1 belongs to an amide bond stretch which could be from the carbonyl group of a protein as reported by Ali et al. [Citation37]; and the peak at 3456 cm−1 may be due to –OH groups present in alcohols and phenols [Citation38]. The absorption peaks at around 3456 and 1738 cm−1 are attributed to the hydroxyl group and binding of C=O functional group with AgNPs [Citation23]. It may therefore be speculated that these bio-molecules are involved in the reduction, capping and stabilization of the produced nanoparticles.
X-ray diffraction analysis of AgNPs
XRD gives information about the crystalline nature and phase of NPs. It also provides a rough idea about the particle size through the Debye Scherrer equation [Citation30]. The dry powder XRD pattern of the AgNPs synthesized from the spice blend () showed that all diffraction peaks corresponded to the characteristic crystalline Face Centred Cubic (FCC) silver lines compared with the standard powder diffraction card of Joint Committee on Powder Diffraction Standards (JCPDS), silver file No. 04–0783.
Strong Bragg reflections which correspond to the reflections of silver are shown for the spice blend nanoparticles. The powder diffraction pattern and Miller indices (h k l) to each peak are assigned and shown in . Three peaks at 2θ values of (64.71, 77.68, 81.87) corresponding to (220), (311) and (222) planes of silver were observed for the spice blend.
Table 1. Powder diffraction pattern and Miller indices (h k l) of the spice blend nanoparticles.
The Bragg reflections confirmed the face-centred cubic nature of metallic silver and the crystalline structure of the nanoparticles. No diffraction peaks from other species could be detected, which indicates that the obtained sample is pure. The X-ray diffraction results clearly showed that the AgNPs formed through the reduction of Ag+ ions by the spice blend extract are crystalline in nature. Similar observations with biosynthesized nanoparticles have been reported [Citation39,Citation40].
In addition to this, the Bragg reflections seem to suggest that the three spice components exhibited equipotent dominance in determining the properties of the spice blend nanoparticles although more dominance could be attributed to ginger and garlic since they both occupy the same space group [Citation25]. Further, the crystalline nature and the particle sizes of the spice blend nanoparticles seem to be a combination of the properties of the individual spices.
Antibacterial activities of AgNPs
Many antimicrobial agents from various sources such as silver ions and inorganic sources have been used and are still been used as antimicrobial agents in various industries. However, these chemical antimicrobial agents are limited by multidrug resistance. Therefore, efforts have focused on alternative ways to overcome the microbial drug resistance; hence the evaluation of biosynthesized AgNPs. In this study, the antibacterial activity of the spice blend AgNPs was evaluated against two Gram-positive and two Gram-negative bacterial species. The biosynthesized Ag-NPs were effective against Streptococcus faecalis, Bacillus cereus, Escherichia coli and Shigella flexneri (), with a notable MIC at 6.25 µg/mL for the four organisms.
Figure 6. Minimum inhibitory concentration (MIC, µg/mL) of silver nanoparticles from the spice blend against bacterial isolates.
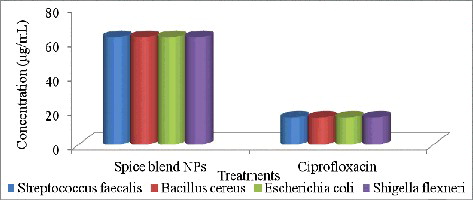
The spice AgNPs showed very profound antibacterial activity against both Gram-positive and Gram-negative bacteria at very low concentration. Gram-positive and Gram-negative bacteria have differences in their membrane structure, the most distinctive of which is the thick peptidoglycan cell membrane layer in Gram-positive bacteria and a double lipopolysacharide (LPS) cell membrane layer in Gram-negative bacteria. According to Sondi and Salopek-Sondi [Citation40] and Gogoi et al. [Citation41], Gram-negative bacteria are more susceptible to AgNPs because the positive charges of Ag+ interact with the negatively charged LPS of the cell membrane with greater affinity when compared to Gram-positive, resulting in holes in the cell membrane, thus disabling their function. However, even though the relatively thick and continuous peptidoglycan cell wall in Gram positive bacteria could restrict the entry of bare AgNPs, interactions of teichoic acid (which spans the peptidoglycan layer) with the bioreductive capping agents (phenol, proteins, esters, etc) of the AgNPs may facilitate their possible entry in Gram positive bacterial species [Citation42].
The results indicate that the spice blend AgNPs exhibited equipotent broad spectrum antibacterial action on both Gram-positive and Gram-negative bacteria tested. Some researchers have reported similar broad spectrum antibacterial action of green-synthesized AgNPs [Citation42–46].
Although several mechanisms have been proposed for the antibacterial action of AgNPs, the shape and size of nanoparticles are important determinants of antimicrobial activity; as smaller sized AgNPs with spherical shape have been reported to exhibit good antibacterial efficacy [Citation41–43,Citation47–49]. The results from this study correlated well with these reports, as the AgNPs produced from the spice blend extract were spherical, small in diameter (6–28 nm) and exhibited high antibacterial activity. Therefore, AgNPs produced from this spice blend may potentially be utilized for the economical production of AgNPs for many food and pharmaceutical applications.
Antioxidant activity of AgNPs
The antioxidant activity of synthesized AgNPs was evaluated by DPPH and ABTS radical scavenging assays, while rutin was used as a standard. The AgNPs exhibited potential free-radical-scavenging activity against both radicals in a dose-dependent manner as shown in . The AgNPs demonstrated very good ability to scavenge free radicals, especially DPPH and ABTS (IC50 < 31.2 and 68.75 µg/mL, respectively), comparable to rutin, a well known standard antioxidant. The AgNPs showed a markedly higher inhibition against DPPH radicals compared to ABTS.
The activity against DPPH and ABTS confirmed the significant antioxidant potential of the spice blend AgNPs. The observed free-radical-scavenging potential of AgNPs compared to Rutin might be attributed to the different types of functional groups from the spice blend that were responsible for the reduction and capping of the AgNPs. This suggests the possibility of this spice blend AgNPs as alternative antioxidant in the therapy of free-radical related diseases. Several authors have reported similar high antioxidant activities of AgNPs against ABTS and DPPH [Citation44–47,Citation50–53].
Spices contain a range of biologically active components, such as polyphenols, proteins, alkaloids, etc., which can donate hydrogen to free radicals and thus break the chain reaction of free radicals at the first initiation step [Citation48]. The results obtained indicated that the antioxidant activity of the AgNPs was enhanced by these bio-components.
Cytotoxicity assay
The results of the preliminary cytotoxicity assay of the samples against the MDA-MB-231 cell line revealed that the spice blend AgNPs were not toxic under the conditions of the assay. The anti-cancer agent paclitaxel gave an IC50 80 ng/mL against the MDA-MB-231 cell line (data not shown). This is an indication that the spice blend AgNPs may not be suitable for cancer therapy but could be quite useful for other applications.
Conclusions
We report herein for the first time the successful biosynthesis and biological activities of AgNPs from a blend of three spices—garlic, ginger and cayenne pepper (1:1:1; w/w/w), and silver nitrate solution. The AgNPs displayed high antibacterial and antioxidant activities against selected bacterial pathogens and free radicals, respectively. These suggest the possible use of the spice blend AgNPs in food packaging materials and also as disinfectant and cleaning agents. Further, the antioxidant activity of the AgNPs revealed their potential to protect against oxidative and free-radical processes. However, the nanoparticles did not exhibit any cytotoxic activity against MDA-MB-231 cancer cells, which suggests that the spice blend AgNPs may be non-toxic to normal cells.
Ethics statement
This article does not contain any studies with human participants or animals performed by any of the authors.
Supplemental_Data.pdf
Download PDF (336.3 KB)Disclosure statement
The authors declare that they have no conflict of interest.
Additional information
Funding
References
- Ahmed S, Ahmad M, Swami BL, et al. A review on plants extract mediated synthesis of silver nanoparticles for antimicrobial applications: a green expertise. J Adv Res. 2016;7(1):17–28.
- Mallick K, Witcomb MJ, Scurrell MS. Self-assembly of silver nanoparticles in a polymer solvent: formation of a nanochain through nanoscale soldering. Mater Chem Phys. 2005;90:221–224.
- Yu DG. Formation of colloidal silver nanoparticles stabilized by Na+-poly (-glutamic acid) silver nitrate complex via chemical reduction process. Colloids Surf B. 2007;59:171–178.
- Iravani S, Korbekandi H, Mirmohammadi SV, et al. Synthesis of silver nanoparticles: chemical, physical and biological methods. Res Pharm Sci. 2014;9(6):385–406.
- Mohanta YK, Panda SK, Jayabalan R, et al. Antimicrobial, antioxidant and cytotoxic activity of silver nanoparticles synthesized by leaf extract of Erythrina suberosa (Roxb.). Frontier Mol Biosci. 2017 [cited 2017 Jul 17];4:14. DOI:10.3389/fmolb.2017.00014.
- Salam HA, Kamaraj RPM, Jagadeeswaran P, et al. Plants: green route for nanoparticle synthesis. Int Res J Biol Sci. 2012;1(5):85–90.
- Ankamwar B, Damle C, Ahmad A, et al. Biosynthesis of gold and silver nanoparticles using Emblica officinalis fruit extract, their phase transfer and transmetallation in an organic solution. J Nanosci Nanotechnol. 2005;5(10):1665–1671.
- Ramachandran R, Krishnaraj C, Stacey LH, et al. Plant extract synthesized silver nanoparticles: An ongoing source of novel biocompatible materials. Ind Crop Prod. 2015;70:356–373.
- Geetha AR, George E, Srinivasan A, et al. Optimization of green synthesis of silver nanoparticles from leaf extracts of Pimenta dioica (Allspice). Scientific World J. 2013 [cited 2017 Jul 17];2013:362890. DOI:10.1155/2013/362890.
- Park Y. New paradigm shift for the green synthesis of antibacterial silver nanoparticles utilizing plant extracts. Toxicol Res. 2014;30(3):169–178.
- Viuda-Martos M, Ruiz-Navajas Y, Fernández-López J, et al. Spices as functional foods. Crit Rev Food Sci Nutr. 2011;51(1):13–28.
- Otunola GA, Afolayan AJ. Evaluation of the polyphenolic contents and antioxidant properties of aqueous extracts of garlic, ginger, cayenne pepper and their mixture. J Appl Bot Food Qual. 2013;86(1):66–70.
- Otunola GA, Oloyede HOB, Oladiji AT, et al. Selected spices and their combination modulate hypercholesterolemia-induced oxidative stress in experimental rats. Biol Res. 2014 [cited 2017 July 17];47:5. DOI:10.1186/0717-6287-47-5.
- Otunola GA, Afolayan AJ. Assessment of the oral safety profile of the aqueous extract from a blend of three medicinal spices in Wistar rats. Trop J Pharm Res. 2017;16(1):91–99.
- Otunola GA, Afolayan AJ. Antidiabetic effect of combined spices of Allium sativum , Zingiber officinale and Capsicum frutescens in alloxan-induced diabetic rats. Frontier Life Sci. 2015;8(4):314–323.
- National Committee for Clinical Laboratory Standards (NCCLS). Methods for dilution antimicrobial susceptibility tests for bacteria that grow aerobically. Approved Standard. NCCLS Document M7-A6. 6th ed. Wayne: PA: NCCLS; 2003.
- Re R, Pellegini N, Proteggente A, et al. Antioxidant activity: applying an improved ABTS radical cation decolorization assay. Free Radic Biol Med. 1999;26:1231–1237.
- Zou Y, Chang SK, Gu Y, et al. Antioxidant activity and phenolic compositions of lentil (Lens culinaris var. Morton) extract and its fractions. J Agric Food Chem. 2011;59:2268–2276.
- Fatima M, Zaidi N-us-SS, Afzal DAF. In vitro antiviral activity of Cinnamomum cassia and its nanoparticles against H7N3 influenza A virus. J Microbiol Biotechnol. 2016;26(1):151–159.
- Nasiri S, Nasiri S. Biosynthesis of silver nanoparticles using Carum carvi extract and its inhibitory effect on growth of Candida albicans. Avicenna J Med Biochem. 2016 [cited 2017 Jul 17];4(2):e37504. DOI:10.17795/ajmb-37504.
- Mittal AK, Chisti Y, Banerjee UC. Synthesis of metallic nanoparticles using plant extracts. Biotechnol Adv. 2013;31(2):346–356.
- Singhal G, Bhavesh R, Kasariya K, et al. Biosynthesis of silver nanoparticles using Ocimum sanctum (Tulsi) leaf extract and screening its antimicrobial activity. J Nanoparticle Res. 2011;13:2981–2988.
- Selvi BCG, Madhavan J, Santhanam A. Cytotoxic effect of silver nanoparticles synthesized from Padina tetrastromatica on breast cancer cell line. Adv Nat Sci Nanosci Nanotechnol. 2016 [cited 2017 July 1];7:035015. DOI:10.1088/2043-6262/7/3/035015.
- Raza MA, Kanwal Z, Rauf A, et al. Size- and shape-dependent antibacterial studies of silver nanoparticles synthesized by wet chemical routes. Nanomaterial. 2016 [cited 2017 July 17];6:74. DOI:10.3390/nano6040074.
- Otunola GA, Afolayan AJ, Ajayi EO, et al. Characterization, antibacterial and antioxidant properties of silver nanoparticles synthesized from aqueous extracts of Allium sativum , Zingiber officinale and Capsicum frutensces. Pharmacogn Mag. 2017;13(50):201–208.
- Das J, Das MP, Velusamy P. Sesbania grandiflora leaf extract mediated green synthesis of antibacterial silver nanoparticles against selected human pathogens. Spectrochim Acta Part A. 2013;104:265–270.
- Jagtap U, Bapat VA. Green synthesis of silver nanoparticles using Artocarpus heterophyllus Lam. seed extract and its antibacterial activity. Ind Crops Prod. 2013;46:132–137.
- VijayKumar PPN, Pammi SVN, Kollu P, et al. Green synthesis and characterization of silver nanoparticles using Boerhaavia diffusa plant extract and their anti-bacterial activity. Ind Crop Prod. 2014;52:562–566.
- Khan I, Saeed K, Khan I. Nanoparticles: properties, applications and toxicities. Arabian J Chem. 2017 [cited 2017 July 17] Forthcoming 2018. DOI:10.1016/j.arabjc2017.05.011.
- Yakout SM, Mostafa AA. A novel green synthesis of silver nanoparticles using soluble starch and its antibacterial activity. Int J Clin Exp Med. 2015;8(13):3538–3544.
- Cardeño Calle L, Londoño López ME. Green synthesis of silver nanoparticles using green coffee bean extract. In: Torres I, Bustamante J, Sierra D, editors. VII Latin American Congress on Biomedical Engineering CLAIB 2016; Bucaramanga, Santander, Colombia; 26–28 Oct 2016; Singapore: Springer; 2017. (IFMBE Proceedings, vol 60).
- Karade VC, Waifalkar PP, Dongle TD, et al. Greener synthesis of magnetite nanoparticles using green tea extracts and their magnetic properties. Mater Res Express. 2017;4(9):096102. DOI:10.1088/2053-1591/9892f.
- Xue B, He D, Gao S, et al. Biosynthesis of silver nanoparticles by the fungus Arthroderma fulvum and its antifungal activity against genera of Candida, Aspergillus and Fusarium. Intern J Nanomed. 2016;11:1899–1906.
- Jyoti K, Baunthiyal M, Singh A. Characterization of silver nanoparticles synthesized using Urtica dioica Linn. leaves and their synergistic effects with antibiotics. J Radiat Res Appl Sci. 2016;9:217–227.
- Li J, Zhu J, Liu X. Ultrafine silver nanoparticles obtained from ethylene glycol at room temperature: catalyzed by tungstate ions. Dalton Transact. 2014;43(1):132–137.
- Macdonald IDG, Smith WE. Orientation of cytochrome C adsorbed on a citrate-reduced silver colloid surface. Langmuir. 1996 ;12(3):706–713.
- Ali DM, Sasikala M, Gunasekaran M, et al. Biosynthesis and characterization of silver nanoparticles using marine Cyanobacterium oscillatoria Willei NTDM01. Digest J Nanomat Biostructures. 2011;6:385–390.
- Udayasoorian C, Kumar RV, Jayabalakrishnan M. Extracellular synthesis of silver nanoparticles using leaf extract of Cassia auriculata. Dig J Nanomater Biostruct. 2011;6(1):279–283.
- Ponarulselvam S, Panneerselvam C, Murugan K, et al. Synthesis of silver nanoparticles using leaves of Catharanthus roseus Linn. G. Don and their antiplasmodial activities. Asian Pacific J Trop Biomed. 2012;2(7):574–580.
- Sondi I, Salopek-Sondi B. Silver nanoparticles as antimicrobial agent: a case study on E. coli as a model for Gram-negative bacteria. J Colloid Interface Sci. 2004;275(1):177–182.
- Gogoi SK, Gopinath P, Paul A, et al. Green fluorescent protein-expressing Escherichia coli as a model system for investigating the antimicrobial activities of silver nanoparticles. Langmuir. 2006;22(22):9322–9328.
- Jain N, Bhargava A, Rathi M, et al. Removal of protein capping enhances the antibacterial efficiency of biosynthesized silver nanoparticles. PLoS ONE. 2015 [cited 2017 July 17];10(7):e0134337. DOI:10.1371/journal.pone.0134337.
- Zhang Y, Yang D, Kong Y, et al. Synergetic antibacterial effects of silver nanoparticles of Aloe vera prepared via a green method. Nano Biomed Eng. 2010;2:252–257.
- Gaikwad S, Bhosale A. Green synthesis of silver nanoparticles using Aspergillus niger and its efficacy against human pathogens. Eur J Exper Biol. 2012;2(5):1654–1658.
- Quinteros MA, Aiassa Martínez IM, Dalmasso PR, et al. Silver nanoparticles: biosynthesis using an ATCC reference strain of Pseudomonas aeruginosa and activity as broad spectrum clinical antibacterial agents. Intern J Biomaterial. 2016 [cited 2017 July 17];2016:5971047. DOI:10.1155/2016/5971047.
- Benakashani F, Allafchian AR, Jalali SAH. Biosynthesis of silver nanoparticles using Capparis spinosa L. leaf extract and their antibacterial activity. Karbala Intern J Modern Sci. 2016;2(4):251–258.
- Morones JR, Elechiguerra JL, Camacho A, et al. The bactericidal effect of silver nanoparticles. Nanotechnol. 2005;16(10):2346–2353.
- Krishnaraj C, Jagan E, Rajasekar S, et al. Synthesis of silver nanoparticles using Acalypha indica leaf extracts and its antibacterial activity against water borne pathogens. Colloid Surface B Biointer. 2010;76(1):50–56.
- Uddin G, Rauf A, Arfan M, et al. Preliminary phytochemical screening and antioxidant activity of Bergenia ciliate. Middle East J Sci Res. 2012;11(8):1140–1142.
- Phull A-R, Abbas Q, Ali A, et al. Antioxidant, cytotoxic and antimicrobial activities of green synthesized silver nanoparticles from crude extract of Bergenia ciliate. Future J Pharm Sci. 2016;2(1):31–36.
- Reddy NJ, Nagoor Vali D, Rani M, et al. Evaluation of antioxidant, antibacterial and cytotoxic effects of green synthesized silver nanoparticles by Piper longum fruit. Mater Sci Eng C Mater Biol Appl. 2014;34:115–122.
- Nagaich U, Gulati N, Chauhan S. Antioxidant and antibacterial potential of silver nanoparticles: Biogenic synthesis utilizing apple extract. J Pharm. 2016 [cited 2917 July 17];2016:7141523. DOI:10.1155/2016/7141523.
- Khalil MMH, Ismail EH, El-Magdoub F. Biosynthesis of Au nanoparticles using olive leaf extract: 1st Nano Updates. Arabian J Chem. 2012;5(4):431–437.