ABSTRACT
The emergence of multidrug-resistant (MDR) microbes has become one of the major threat globally. Infectious diseases are the second leading cause of death, two-third of which are caused by Gram-negative bacteria. The increasing number of multidrug resistant (MDR) microbes is quite alarming and has raised the necessity of development of new antibacterial drugs. Escherichia coli and Klebsiella have been reported among the top most resistance-developing pathogens. Ricinus communis is an important medicinal plant reported to possess antimicrobial phytochemicals such as α-pinene. The hexane treated crude ethanolic extract of R. communis was evaluated against Gram-negative bacteria E. coli and Klebsiella oxytoca. The agar well diffusion assay was used to determine the antibacterial activity. In the present study, we have shown experimentally that leaf extract of R. communis can induce the deterioration of the inner and outer cell membranes of E. coli and K. oxytoca and decrease their viability at a concentration of 50 mg/ml. Transmission electron microscopic results revealed cell membrane damage, cellular disintegration and release of cytoplasmic content, leading to cell death. To our knowledge, this is the first study of the antibacterial activity of R. communis against E. coli and K. oxytoca by Transmission electron microscopy. The ultramicroscopic observations showed that the phytochemical present in the leaf extract of R. communis could penetrate the bacterial cell, causing rupture of cell membranes and hence confirm the cytotoxic and antimicrobial property of R. communis.
Introduction
The emergence of multidrug-resistant (MDR) microbes, evoked by overuse of antibiotics, has led to a dramatic decrease in the efficacy of commonly used major antimicrobial drugs, which has now become a serious problem worldwide. This growing problem has raised the necessity of exploration and development of novel antimicrobials such as natural products, fabricated antibiotics and plant phytochemicals [Citation1,Citation2]. Development of new synthetic compounds for inhibiting various microorganisms has been in progress for the last six decades [Citation3]. There have been a large number of reports about the development of resistance to various antimicrobials which are currently available in the market. There has been a tremendous rise in the incidences of multi-drug resistant pathogens with complex mechanisms to escape the action of antimicrobials [Citation4]. Development of novel antimicrobial compounds is one of the strategies employed by unleashing the hidden potential of natural resources such as medicinal plants [Citation5]. There are several advantages in using herbs or natural products as a source for the developmentof novel antibiotics. Medicinal plants are known to produce very complex compounds which are still beyond the capability of synthetic chemists. Moreover, there is minimal risk of side effects associated with medicinal plants as compared to synthetic drugs, as the edible plants or traditional medicine is reported to have very less or no side effects [Citation6]. Another advantage is their availability and cost-effectiveness [Citation7].
Medicinal plants are known to be valuable resources for the development of novel antimicrobial drugs [Citation8]. Medicinal herbs are better equipped at metabolic bio-engineering of active compounds than conventional laboratory approaches [Citation9]. Several studies are currently focusing on natural medicinal herbs which are regularly used in folkloric medicine and have a high probability of developing as bactericidal or bacteriostatic agents [Citation10].
Infectious diseases caused by bacterial pathogens continues to be one of the major health concern worldwide, affecting millions of people every year [Citation11,Citation12]. During the past 30 years, 37 new human pathogens have been reported to be as disease causing agents in human. Infectious diseases are responsible for high morbidity throughout the world, accounting for about a quarter of deaths around the globe [Citation13].
Escherichia coli and Klebsiella sp. are major Gram-negative bacterial pathogens that are responsible for causing life–threatening human diseases [Citation14–17]. The emergence of new resistant strains of Klebsiella oxytoca and E. coli have revealed virulent growth and enhanced ability to cause disease in a healthyindividual [Citation15]. Antibacterial drugs are used on a large scale to kill bacterial pathogens and safeguard the public health. A series of antimicrobial substances, including quaternary ammonium compounds, antibiotics and metal ions, can target and inhibit bacterial growth and in turn, damage the morphological features of microorganisms. However, various issues such as complexity in chemical synthesis, antibiotic resistance, environmental pollution and cost-effectiveness should be considered before developing new synthetic antimicrobial drugs [Citation2,Citation16,Citation17]. Of late, antimicrobial agents from natural resources, have provided opportunities to tackle some of these issues [Citation8]. Medicinal plants are considered an important source for discovering new antimicrobial drugs. Ricinus communis L. is one of the most widely studied plants due to its tremendous therapeutic potential including anti-microbial activity [Citation18,Citation19].
A major objective in antimicrobial drug discovery is the development of novel compounds with the potential of killing resistant pathogens by various modes of action, which are novel and which could impede the emergence of resistance in the microorganisms [Citation20]. R. communis is a naturally available medicinal plant and the active compounds present in it have shown antimicrobial activity against a large number of bacteria and fungi [Citation18,Citation21].
In the present study, we have used leaf extract of R. communis to assess the antibacterial activity against E. coli and K. oxytoca. R. communis produces various phytochemicals such as ricinoleic acid, rutin, quercetin, pinene, alpha-pinene, ricin, alpha-thujone, gallic acid and gentistic acid. These compounds have been reported to have antibacterial activity against various pathogens [Citation22,Citation23]. Extract of R. communis has shown antibacterial activity against various bacterial pathogens [Citation24]. In this study, we have used hexane treated ethanolic extract of leaf of R. communis and the pathogenic bacteria K. oxytoca and E. coli were used as model bacterial species to study and evaluate its antibacterial activity by using Transmission Electron Microscopy (TEM). Among the various approaches used for studying the antimicrobial activity of the plant, TEM is an imaging-based technique applied to observe structural alterations in the cell morphology [Citation25].
Materials and methods
Plant collection and processing
Leaves of R. communis were collected from KAU Farm, in Jeddah, Saudi Arabia. A sample was deposited to herbarium repositories in the Department of Biology, Faculty of Sciences, King Abdulaziz University, Jeddah, Saudi Arabia. Leaves were properly cleaned with tap water followed by distilled water. Leaves were dried under the shade and then crushed into a fine powder using an electric blender. Dried leaf powder was first extracted by mixing 25 g of plant leaf powder with 100 ml of hexane followed by 100 ml of ethanol in a shaking incubator for 48 h at 25 °C. The mixture was filtered through Whatman No. 1 filter paper. Ethanol was completely removed from the filtrate by using Rotavapor (BUCHI, Switzerland), at 40 °C and under reduced pressure. The dried leaf extract was stored at –20 °C until further use [Citation24].
Bacterial strains
E. coli (ATCC# 25 922), K. oxytoca(ATCC # 700 324) and Staphylococcus aureus (ATCC# 25 923) were procured from the Department of Microbiology, KAU Hospital, Jeddah KSA. Bacterial cultureswere grown in Nutrient broth, overnight, at 37 °C. Bacterial strains were maintained and preserved in nutrient agar slants at 4 °C and 50% glycerol broth at −80 °C.
Antibacterial activity
Agar well diffusion assay determined the antimicrobial activity of the Ricinus leaf extract. In brief, 100 μL of the bacterial suspension was optimized to 0.5 McFarland standards (106 CFU/mL) and plated on the Muller Hinton agar plates by using a spreader. Around 6 mm wells were made on agar plates for applying the plant extract. Different doses of plant extract were added to the wells and incubated for 1 h at room temperature. The plates were further incubated at 30 °C for 24 h. 10% of DMSO was used as negative control. Around 50 µL of penicillin/streptomycin solution (100 μg/mL streptomycin; 100 U/mL penicillin) was used as a positive control. The experiments were repeated in triplicates and zones of inhibition in millimeters was calculated as a measure of antibacterial activity.
Transmission electron microscopy
Bacterial strains were treated with Ricinus leaf extract and were investigated by negative contrast TEM as described by Haschemyer and Meyers [Citation26]. Bacteria were transferred to a 300 mesh carbon-coated copper grid by keeping the grid coated-side-down on a drop of bacterial culture for about 2 min. Excess liquid was removed by touching the grid with a piece of Whatman filter paper, and bacteria were then negatively stained by floating the grid on a drop of 2% (w/v) PTA solution (pH 6.5) for 1 min. Excess liquid was again removed by touching the grid with a piece of Whatman filter paper, and the bacterial preparation was examined by TEM using a JEM-1011 microscope (Jeol, Tokyo, Japan) at 80 KV accelerated voltage [Citation26,Citation27].
Results and discussion
Leaf extract of Ricinus was evaluated for its antimicrobial activity by agar well diffusion method. Leaf extract at 2 mg/mL dose showed significant antimicrobial activity against E.coli with a zone of inhibition of 18 mm in size (). Leaf extract also exhibited significant antimicrobial activity against K. oxytoca with the zone of inhibition of 17 mm in size (). However, against the Gram-positive bacterium S. aureus, Ricinus leaf extract exhibited low anti-bacterial activity as seen in . Penicillin/streptomycin, used as a positive control, resulted in a zone of inhibition of 22 mm against both the bacterial pathogens. In treatment with only DMSO solvent control, no zone of inhibition was detected. Varying doses of the Ricinus leaf extract (i.e. 2–100 mg/mL) were tested against bacterial pathogens using the broth dilution method and Minimum inhibitory concentration (MIC) was determined. Bacterial growth was completely inhibited at the dose of 90 mg/mL (MIC value) and above for K. oxytoca as well as for E. coli (data not shown).
Table 1. Determination of antibacterial activity of Ricinus leaf extract against various pathogens.
Effect of Ricinus leaf extract on bacterial strains morphology was also investigated by negative contrast Transmission electron microscopy. After the exposure of E. coli and K. oxytoca cells with leaf extract of R. communis, many protrusions at outer membrane along with lysed membranes were seen. The TEM images revealed the formation of blebs on the bacterial surface. There was a clear indication of cell membrane damage, cellular disintegration and release of cytoplasmic content, leading to cell death. Treatment of E.coli cells with the leaf extract of R. communis resulted in morphological changes including cell membrane damage and release of cellular content as seen under the microscope ().
Figure 1. Transmission electron micrograph depicting the activity of Ricinusleaf extract on E.coli cell. (A) Control, untreated E. coli cell; (B,C) E.coli cells treated with 50 mg/mL of Ricinus leaf extract.
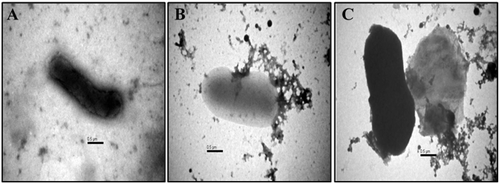
The antimicrobial activity of leaf extract against K. oxytoca was confirmed after observation of Electron microscopic images obtained after overnight incubation of Klebsiella with Ricinus leaf extract. The cells after treatment were observed with characteristic shape with detectable shrinkage and irregular shape. Klebsiella cells have shown aberrant morphology accompanied by cracking and rupturing and release of cytoplasmic content ().
Figure 2. Transmission electron micrograph depicting the activity of Ricinus leaf extract on Klebsiella oxytoca. (A) Control Klebsiella cells; (B,C) Klebsiella cells treated with 50 mg/mL of Ricinus leaf extract
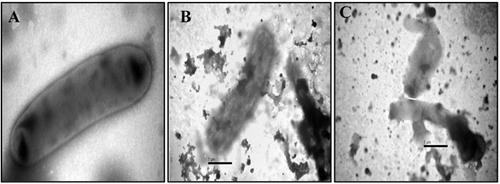
The phytochemicals present in R. communis are known to possess antibacterial activity. Morphological variations in the organization of bacterial cell wall may influence the efficacy of the phytochemicals found in the Ricinus leaf extract. Gram-negative bacteria consist of a thin peptidoglycan layer (2–3 nm) in between cytoplasmic membrane and the outer membrane when compared to Gram-positive bacteria which is devoid of the outer membrane but contains a peptidoglycan layer of 30 nm thickness. This thicker wall may act as a barrier protecting the cell from the entry of phytochemicals into the cytoplasm. The bacterial strain may also regulate the bioavailability of the phytochemicals present in the Ricinus leaf extract by modification or inactivation.
As observed in TEM micrographs, Ricinus leaf extract promotes detachment of outer cell membrane and spilling off the cytoplasmic content. Moreover, the cell wall is severely damaged and shows undulations, indicating a loss of integrity, and the aggregated cytosolic material is seen on the cell surface. The cytoplasmic membrane of E.coli cells was irregular and separated from the outer cell membrane after the overnight treatment and release of the intracellular contents was observed. Treatment of Klebsiella cells with Ricinus leaf extract, resulted in lysis of outer cell membrane with the formation of protrusions as noted in the TEM images. Moreover, the cellular contents were leaked completely from the cytoplasm.
Destruction of the Gram-negative bacterial cell membrane as observed in TEM analysis would be one of the probable modes of action for the phytochemicals present in Ricinus leaf extract. The release of intracellular material, which was caused by the damage of the membrane, ultimately leads to cell death [Citation28]. It has been claimed that the application and use of antibacterial agents that are responsible for disruption of the membrane bilayer is a more efficient approach for controlling the growth of bacterial pathogens. Since these agents have the greater advantage of being active against dormant bacteria (with some slow or even no growth), they are of great interest. Such metabolically inactive organisms will be able to survive at high doses of various other classical antibiotics and long-term treatment is therefore required for drug efficacy [Citation29,Citation30].
R. communis is known to produce various diverse compounds such as steroids, alkaloids, flavonoids, glycoside and saponins, which are responsible for the therapeutic benefits such as antimicrobial, anti-cancer, anti-inflammatory, etc. Various phytochemicals present in Ricinus are ricinoleic acid, rutin, quercetin, pinene, alpha-thujone and gallic acid. Most of the studies with regards to antimicrobial activity have been conducted using crude extract of Ricinus leaves. Recently, one study by Masoko and Nemudzivhadi (2015) had demonstrated the isolation of stigmasterol and β-sitosterols from the leaves of R. communis with antibacterial activity [Citation21]. In order to develop a new antimicrobial compound, we would perform the purification of various phytochemicals and identify the compound with potent antimicrobial activity in our future studies.
Conclusions
Transmission electron microscopy confirmed antimicrobial activity of R. communis against bacterial pathogens after observing the structural changes due to the action of phytochemicals present in the leaf extract. Electron micrographs of E. coli and K. oxytoca showed dramatic alterations in cell morphology after exposure to Ricinus leaf extracts. In both pathogens, cell wall disruptions were observed, which resulted in the release of intracellular material and ultimately, leading to empty and flaccid cells. The cellular damage seen on TEM examination indicated the lethal effects of Ricinus leaf extracts on the pathogens. For the first time, the antibacterial activity of Ricinus leaf extract against E. coli and K. oxytoca was confirmed by using TEM micrographs. By isolating and purifying the specific phytochemical responsible for antimicrobial activity and analyzing its mode of action by the molecular study can lead to the discovery of novel antimicrobial compound from R. communis.
Acknowledgements
This study was technically and financially supported by the Deanship of Scientific Research (DSR), King Abdulaziz University, Jeddah under Grant number 62–130–35-HiCi.
Disclosure statement
The authors declare that there are no conflicts of interests.
Additional information
Funding
References
- Cragg GM, Newman DJ. Natural products: a continuing source of novel drug leads. Biochim Biophys Acta. 2013;1830(6):3670–3695.
- Ventola CL. The antibiotic resistance crisis: Part 1: causes and threats. Pharm Ther. 2015;40(4):277–283.
- Bérdy J. Thoughts and facts about antibiotics: where we are now and where we are heading. J Antibiot. 2012;65(8):441.
- Davies J, Davies D. Origins and evolution of antibiotic resistance. Microbiol Mol Biol Rev. 2010;74(3):417–433.
- Dias DA, Urban S, Roessner U. A historical overview of natural products in drug discovery. Metabolites. 2012;2(2):303–336.
- Ekor M. The growing use of herbal medicines: issues relating to adverse reactions and challenges in monitoring safety. Front Pharmacol. 2014;4:177.
- Balouiri M, Sadiki M, Ibnsouda SK. Methods for in vitro evaluating anti- microbial activity: a review. J Pharmaceutical Anal. 2016;6(2):71–79.
- Cowan MM. Plant products as antimicrobial agents. Clin Microbiol Rev. 1999;12(4):564–582.
- Pan SY, Zhou SF, Gao SH, et al. New perspectives on how to discover drugs from herbal medicines: CAM's outstanding contribution to modern therapeutics. Evid Based Complement Alternat Med. 2013;2013: 627375 . doi:10.1155/2013/627375.
- Verma S, Singh SP. Current and future status of herbal medicines. Vet World. 2008;1(11):347–350.
- Tatem AJ, Rogers DJ, Hay SI. Global transport networks and infectious disease spread. Adv Parasitol. 2006;62:293–343.
- Frieden T. Antibiotic resistance threats in the United States. Atlanta (GA): Centers for Disease Control and Prevention (CDC); 2013 [cited 2017 Aug 07]. p. 2–50; Available from: https://www.cdc.gov/drugresistance/threat-report-2013/index.html
- Saker L, Lee K, Cannito B, et al. Globalization and infectious diseases: a review of the linkages. Geneva: World Health Organization; 2004. (Special Topics in Social, Economic and Behavioural (SEB) Research; 3:1–67).
- Podschun R, Ullmann U. Klebsiella sp. as nosocomial pathogens: epidemiology, taxonomy, typing methods, and pathogenicity factors. Clin Microbiol Rev. 1998;11(4):589–603.
- Joainig MM, Gorkiewicz G, Leitner E, et al. Cytotoxic effects of Klebsiella oxytoca strains isolated from patients with antibiotic-associated hemorrhagic colitis or other diseases caused by infections and from healthy subjects. J Clin Microbiol. 2010;48(3):817–824.
- Levy SB, Marshall B. Antibacterial resistance worldwide: causes, challenges, and responses. Nat Med. 2004;10(12):S122–129.
- Rattan A, Raj VS, Saini KS. Recent advances in the discovery and development of new drugs against Gram-negative pathogens. In: Atta-ur-Rahman, editor. Frontiers in clinical drug research. Vol.1, Anti-infectives. UK: University of Cambridge; 2014. p. 237–262.
- Naz R, Bano A. Antimicrobial potential of Ricinus communis leaf extracts in different solvents against pathogenic bacterial and fungal strains. Asian Pac J Trop Biomed. 2012;2(12):944–947.
- Jena J, Gupta AK. Ricinus communis Linn: a phytopharmacological review. Int J Pharm Pharm Sci. 2012;4(4):25–29.
- Rabanal FA, Grau-Campistany X, Vila-Farres J, et al. A bioinspired peptide scaffold with high antibiotic activity and low in vivo toxicity. Sci Rep. 2015 [cited 2017 Aug 07];5:10558. DOI:10.1038/srep10558
- Masoko P, Nemudzivhadi V. Isolation and chemical structural characterization of the mixture of two related phytosterols from Ricinus communis L. (Euphorbiaceae) leaves. Eu J Med Plants. 2015;10(2):1–11.
- Dhar P, Chan P, Cohen DT, et al. Synthesis, antimicrobial evaluation, and structure-activity relationship of α-pinene derivatives. J Agric Food Chem. 2014;62(16):3548–3552.
- Claude TJ. Chemical composition, antibacterial and antifungal activity of the essential oil of Pinus patula growing in rwanda. Am J Biomed Life Sci. 2014;2(3):55.
- Alamri SA, Moustafa MF. Antimicrobial properties of 3 medicinal plants from Saudi Arabia against some clinical isolates of bacteria. Saudi Med J. 2012;33(3):272–277.
- de Jonge N, Ross FM. Electron microscopy of specimens in liquid. Nat Nano. 2011;6(11):695–704.
- Haschemyer RH, Meyers RJ. Negative staining. In: Hayat MA, editor. Principles and techniques of Electron Microscopy. Vol. 2, Biological Applications. New York: Van Nostrand Reinhold; 1972. p. 101–143.
- Ohi M, Li Y, Cheng Y, et al. Negative staining and image classification - powerful tools in modern electron microscopy. Biol Proced Online. 2004;6:23–34.
- Li H, Chen Q, Zhao J, et al. Enhancing the antimicrobial activity of natural extraction using the synthetic ultrasmall metal nanoparticles. Sci Rep. 2015 [cited 2017 Aug 07];5:11033. DOI:10.1038/srep11033
- Hurdle JG, O' Neill AL, Chopra I, et al. Targeting bacterial membrane function: an underexploited mechanism for treating persistent infections. Nature Rev Microbiol. 2011;9(1):62–75.
- Agnihotri S, Mukherji S, Mukherji S. Size-controlled silver nanoparticles synthesized over the range 5–100 nm using the same protocol and their antibacterial efficacy. RSC Adv. 2014;4(8):3974–3983.