ABSTRACT
To investigate the biology functions of ERF transcriptional factor in Populus simonii × P.nigra, the proteins interacting with ERF were screened by the yeast two-hybrid method. The bait vector harbouring ERF and cDNA library of Populus simonii × P.nigra were constructed. The bait had no self-activation and toxicity to yeast cells in the yeast two-hybrid system. The library titering experiment indicated that it could meet the requirement for the yeast two-hybrid library screen. Five proteins that interacted with ERF were obtained, including galactinol-sucrose galactosyl transferase 2, phosphoinositide-specific phospholipase C family protein, MLP-like protein, senescence-associated protein and an unknown protein. Real-time polymerase chain reaction showed that the expression levels of ERF and five interacting protein genes increased after salt treatment. Gene ontology analysis associated most of the interacting proteins with stress response. These results showed that ERF might share similar function with these proteins and they act together to regulate the salt response of Populus simonii × P.nigra. This study provided experimental evidence for further revealing the biological function of the ERF transcription factor in poplar.
Introduction
With the completion of many plant genome sequencing projects and the development of bioinformatics, a variety of transcription factors (TFs), which are associated with drought, salt, cold, hormone, pathogen response, growth and development, have been isolated from higher plants since Paz-Ares first reported the TF from maize in 1987 [Citation1]. Ethylene-responsive factors (ERF) are one of the largest groups of TFs in plants and are closely related to the response of plants to biotic and abiotic stress [Citation2]. ERF genes have been cloned and analyzed in wheat [Citation3], Arabidopsis thaliana [Citation4], grape [Citation5], Jatropha curcas [Citation6], cotton [Citation7], soybean [Citation8], Hevea brasiliensis [Citation9], tobacco [Citation10], potato [Citation11], maize [Citation12] and poplar [Citation13,Citation14]. Fifty-eight transcription factor families have been found in the poplar genome, encoding 4287 different transcription factors containing 209 AP2/ERF transcription factors [Citation15]. The ERF transcription factor family, which accounts for 9% of the TFs in plants, contains a 58- or 59-amino acid conserved domain. The ERF transcription factors are widely involved in the regulation of plant growth and development and various stress responses [Citation16]. According to the number and characteristics of the AP2/ERF domains, the ERF transcription factors can be divided into three families: AP2, ERF and RAV. The AP2 family contains two AP2/ERF domains, which play a very important role in the regulation of plant growth and development [Citation17]. The RAV family containing one AP2/ERF domain and a B3 domain, plays important roles in ethylene, brassinolide, biotic and abiotic stress responses [Citation18]. The ERF family contains one AP2/ERF domain and is divided into two subfamilies: CBF/DREB subfamily and ERF subfamily. The differences between CBF/DREB and ERF subfamilies are the 14th and 19th amino acid. The 14th and 19th amino acids of DREB are valine and glutamic acid, while ERF is alanine and aspartic acid. DREB and ERF transcription factors are mainly involved in plant response to hormone and environmental stress [Citation19].
ERF transcription factors can interact with other proteins to regulate the expression of downstream target genes involved in development and resistance to abiotic and biotic stress. Qiu et al. [Citation20] constructed a wheat cDNA library and screened proteins interacting with ERF transcription factor W17 by the yeast two-hybrid system and showed that HSP90 and PPR proteins, which were involved in signal transduction and immune processes, interacted with W17 in vivo. ERF4 in Malus xiaojinensis (MxERF4) interacted with MxFIT and MxIRO2, as shown by yeast two-hybrid and bimolecular fluorescence complementation assays (BiFC), and the interactions had a positive regulation effect on MxIRT and MxHA2. When the expression of MxIRT and MxHA2 rose, the active iron content and efflux in M. xiaojinensis roots were increased significantly [Citation21]. The interaction of OsERF115 with OsNF-YB1 in Oryza sativa was confirmed and OsNF-YB1 specifically regulated the transcription of downstream genes during rice endosperm development by forming protein complexes consisting of OsNF-YB1, OsNF-YC and ERF [Citation22]. CitVHA-c4, a kind of V-ATPase in citrus, was observed to interact with CitERF13 using a yeast two-hybrid screen and BiFC. The transcript abundance of CitERF13 was concomitant with CitVHA-c4, and CitERF13 over-expression led to significant citric acid accumulation. ERF-VHA interactions appeared to be involved in citric acid accumulation [Citation23]. A few studies have been performed on the interaction with ERF in plants, but little is known about Populus ERF interaction in response to salt stress.
In this study, interacting proteins were obtained by the yeast two-hybrid method based on the cloning of the ERF gene from Populus simonii × P.nigra, and the expression pattern of ERF and interacting protein genes were analyzed by real-time polymerase chain reaction (PCR). This study provided experimental evidence for studying the salt tolerance mechanism of ERF transcription factor and its application in transgenic research.
Materials and methods
Plant growth and stress treatment
The twigs of Populus simonii × P. nigra were grown in pots containing water at 26 °C /22 °C (day/night) with 75% relative humidity, 16 h photoperiod and 175 μmol/(m2·s) light intensity. Two-month-old seedlings with new roots and leaves were divided into two groups. One group was grown under normal conditions (as described above) as a control and the other one was stressed by 150 mmol/L NaCl solution. One gram of leaves were harvested from three seedlings at 0 and 24 h of treatment for real-time PCR. The leaves were harvested under normal conditions and 150 mmol/L NaCl at 24 h for cDNA library construction. The samples were frozen immediately in liquid nitrogen. The tissues were then stored at −80 °C until use.
Cloning and testing bait for autoactivation and toxicity
The full-length open reading frame (ORF) of the ERF gene from P. simonii × P. nigra (PsnERF) [Citation24] with an EcoR I restriction site at the 5' end and a BamH Isite at the 3' end was obtained by PCR using the following primers: 5'-TTCATTCGAATTCCATGTCGCTATT-3'/5'-CGGGATCCCGTTAACCAGTGGAGGAAGGAC-3'. The fragment, after double restriction enzyme digestion, was inserted into the EcoR I/BamH I sites of the vector pGBKT7. The pGBKT7-PsnERF recombinant vector was transformed into yeast Y2H according to Yeastmaker™ Yeast Transformation System 2 (Clontech, 630439). In order to test the bait for autoactivation and toxicity, pGBKT7-PsnERF and pGBKT7 were transformed into Y2H and then the transformed clones were screened by SD/-Trp, SD/-Trp/x-gal, SD/-Trp/x-gal/AbA media at 30 °C for 3–5 days.
cDNA library construction and yeast two-hybrid assays
The total RNA was extracted from the pooled leaves (control and treatment) by the SDS method [Citation25]. The cDNAs with end sequences homologous to the prey vector pGADT7-Rec using SMART technology, and SmaI-linearized pGADT7-Rec were co-transformed into yeast strain Y187 and plated on SD/Leu agar medium at 30 °C for 3–4 days, according to the Mate & Plate™ Library System (Clontech, 630 490). All colonies were harvested and pooled into YPDA medium (containing 25% glycerol) for library screening. The bait strain (Y2HGold [pGBKT7+Bait]) was hybridized with the library strain according to the Matchmaker Gold Yeast Two-Hybrid System (Clontech) and the zygotes were observed under a microscope (MDX4-T, Mshot) during the yeast two-hybrid process. The colonies were screened by SD/-Trp/-Leu/x-gal/AbA (DDO/X/A) medium at 30 °C for 3–5 days. Then, all the blue colonies that grew on DDO/X/A were transferred onto higher stringency SD/-Ade/-His/-Trp/-Leu/x-gal/AbA (QDO/X/A) agar plates using a pipette tip. A single blue colony from QDO/X/A was picked for restreaking 2–3 times. Positive clones from QDO/X/A were identified by PCR using the specific primers MDF (5'-CTATTCGTGATGAAGATACCCC ACCAAACCC-3') and MDR (5'-GTGAACTTGCGGGGTTTTTTCAGTATCTACGATT-3') and then sequenced. The results were analyzed via BLAST (Basic local alignment search tool; https://blast.ncbi.nlm.nih.gov).
Rescue of the prey plasmid and confirmation of positive interactions
After yeast two-hybrid screening, the prey plasmids from positive clones were rescued using E.Z.N.A.® Yeast Plasmid Kit (Omega). The bait and prey plasmids were co-transformed into Y2H and the genuine interactions were selected by QDO/X/A.
Expression of ERF and interacting protein in response to salt stress
Total RNA of each sample was extracted from leaves of P. simonii × P. nigra under salt stress and normal conditions. One microgram of total RNA was reverse transcribed into cDNA in a 20 µL volume using a PrimeScript™ RT reagent kit with gDNA Eraser (Takara). The cDNAs served as templates in real-time PCR using gene-specific primers (). The Populus actin (EF418792) gene was used as internal control in order to normalize the amount of total RNA in each reaction. Real-time PCR was performed using the SYBR Premix Ex Taq™ (Takara) on a CFX connect real-time system (Bio-RAD). The amplification was performed with the following cycle parameters: 94 °C for 30 s, followed by 40 cycles of 94 °C for 12 s, 53 °C for 30 s, 72 °C for 30 s, and 1 s at 81 °C for plate reading.
Table 1. Real-time PCR primer sequences.
Three independent biological replications and three experimental technical replications were performed for each treatment. The mean of the nine replications was used for relative expression quantitation. Gene expression levels were calculated by the 2−△△Ct method [Citation26], defined as: Ct = (Ct-target−Ct-control)2–(Ct-target− Ct-control)1.
Results and discussion
Confirmation of bait toxicity and autoactivation
The vectors of pGBKT7 and pGBKT7-PsnERF were transformed into yeast strain Y2H respectively and the transformants were screened on SD/-Trp medium. The results showed that the colonies containing pGBKT7 and pGBKT7-PsnERF grew well on SD/-Trp medium. This demonstrated that the bait ERF was not toxic when expressed in yeast. The Y2H yeasts containing pGBKT7-PsnERF could grow well on SD/-Trp and SD/-Trp/x-gal media but the colonies could not turn blue on SD/-Trp/x-gal medium (A and B). The transformant could not grow on SD/-Trp/x-gal/AbA medium (C). That indicated that the bait ERF did not autonomously activate the reporter genes in Y2H in the absence of prey protein, and was suitable as bait for screening the cDNA library.
Construction of cDNA library and yeast two-hybrid screening
The titer of this original cDNA library was 1.56 × 107 cfu/mL. During the yeast two-hybrid process, zygotes resembling a clover leaf were observed under a microscope. A zygote had a typical 3-lobed structure which represented the two haploid parental cells and the budding diploid cell (). A total of 719 colonies were obtained on DDO/X/A medium and 156 colonies were selected on QDO/X/A medium (). Twenty-two positive colonies were determined by PCR. The PCR products were sequenced and analyzed using BlastX. The 22 positive clones determined by PCR represented 13 unique proteins (). Thirteen prey vectors were rescued and co-transformed with bait pGBKT7-ERF into Y2H, respectively. After screening on QDO/X/A medium, there were five positive genuine proteins (). The interacting proteins were galactinol-sucrose galactosyl transferase 2, phosphoinositide-specific phospholipase C family protein, MLP-like protein 328, senescence-associated protein and an unknown protein via BLAST ().
Table 2. Proteins interacting with PsnERF.
Expression analysis of ERF and interacting protein gene in response to salt stress
The expression patterns of ERF and five interacting protein genes were analyzed by real-time PCR. The results showed that the expression levels of ERF and the five interacting protein genes were all increased after salt treatment. In conditions of salt stress, the expression levels of ERF and 7–1 were significantly higher than those under normal conditions (P < 0.05). The expression levels of L1, 2–1 and 4–1 were extremely higher than those under normal conditions (P < 0.01). The expression patterns of interacting protein genes were similar to that of the ERF gene (). These results indicated that ERF and interacting proteins might act together to regulate the salt response of P. simonii × P. nigra, and the five interacting proteins might play important roles in salt tolerance.
Figure 6. Expression levels of ERF and interacting protein genes in leaves of Populus simonii × P.nigra under salt stress.
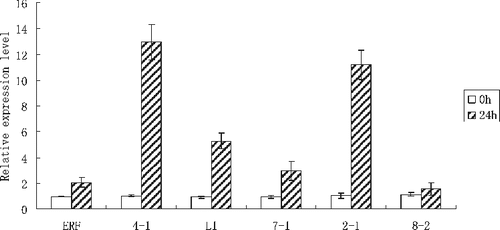
The function of interacting proteins with ERF was analyzed by gene ontology (GO) (). Galactinol-sucrose galactosyl transferase, encoded by L1, participates in the raffinose catabolic process; this reaction and pathway results in the breakdown of raffinose [Citation27]. Galactinol-sucrose galactosyl transferase responds to karrikin and oxidative stress. It was suggested that galactinol and raffinose possibly scavenge hydroxyl radicals as a novel function to protect plant cells from oxidative damage caused by methylviologen (MV) treatment, salinity or chilling [Citation28]. The interacting protein 2–1 is a senescence-associated protein and is associated with mitochondrial respiratory demand and dysfunction [Citation29]. The 2–1 protein was localized in the mitochondrion and was predicted to have a function of mitochondrial RNA polymerase, which was inferred from genetic interaction (IGI) of gene ontology. Phosphoinositide-specific phospholipase C family protein (PLC), encoded by 7–1, was predicted to play an important role in signal transduction processes, mediating the cellular actions of a variety of hormones and growth factors. For example, PLC2 is involved in auxin biosynthesis and signaling, thus modulating the development of both male and female gametophytes in Arabidopsis [Citation30]. The stress-induced Ca2+ signaling, which is essential for controlling Na+ accumulation in leaf blades, is dependent on phospholipase C (PLC) in Oryza sativa, thus establishing whole plant salt tolerance [Citation31]. The 8–2 gene encoded MLP-like protein 328 (major latex protein, MLP) which was predicted to be responsive to biotic stimulus, phenylpropanoid, zinc ion, etc. GhMLP28, a defense-related major latex protein from upland cotton, interacts with ethylene response factor 6 (GhERF6). The distribution of GhMLP28 in the nucleus is dependent on the presence of GhERF6. GhMLP28 acts as a positive regulator of GhERF6 and the two proteins might contribute to protection against Verticillium dahliae infection in cotton plants [Citation32]. It was also reported that MLP was associated with vegetative to reproductive phase transition of meristem [Citation33].
Table 3. Gene ontology analysis on proteins interacting with ERF.
All the results indicated that most of the interacting proteins with ERF were related with response to stress and these provided a new approach to understand the regulatory mechanism of ERF transcript factor under stress conditions. GST pull-down and co-immunoprecipitation (Co-IP) assays will be applied to validate the interaction with ERF in further studies.
Conclusions
In this work, we successfully obtained five proteins interacting with ERF transcription factor from P. simonii × P.nigra. Most of the proteins were associated with stress response. This study provided useful information in revealing the function of ERF transcript factor in response to salt stress.
Disclosure statement
No potential conflict of interest was reported by the authors.
Additional information
Funding
References
- Paz-Ares J, Ghosal D, Wienand U, et al. The regulatory c1 locus of Zea mays encodes a protein with homology to myb proto-oncogene products and with structural similarities to transcriptional activators. EMBO. 1987;6(12):3553–3558.
- Junya M, Kazuo S, Kazuko YS. AP2/ERF family transcription factors in plant abiotic stress responses. BBA-Gene Regul Mech. 2012;1819:86–96.
- Zhang L. Functional analysis of salt-responsive gene TaERF5 in wheat [M.Ag. thesis]. Beijing: Chinese Academy of Agricultural Sciences; 2013.
- Bui LT, Giuntoli B, Kosmacz M, et al. Constitutively expressed ERF-VII transcription factors redundantly activate the core anaerobic response in Arabidopsis thaliana. Plant Sci. 2015;236:37–43.
- Zhuang J, Peng RH, Cheng ZM, et al. Genome-wide analysis of the putative AP2/ERF family genes in Vitis vinifera. Sci Hortic-Amsterdam. 2009;123:73–81.
- Wang XH, Han HY, Yan J, et al. A new AP2/ERF transcription factor from the oil plant Jatropha curcas confers salt and drought tolerance to transgenic tobacco. Appl Biochem Biotech. 2015;176:582–597.
- Liu K. Gene cloning and characterization of B3 and B1 subgroup transcription factors of ERF family from Gossypium barbadense L. [D.Sc.thesis]. Beijing: Chinese Academy of Agricultural Sciences; 2011.
- Sun X. Functional identification and promoter separation of stress-resistent related transcription factor genes, GmDREB3, from soybean (Glycine max L.) [M.Ag. thesis]. Urumchi: Xinjiang Agriculture University; 2007.
- Duan C, Argout X, Gébelin V, et al. Identification of the hevea brasiliensis AP2/ERF super family by RNA sequencing. BMC Genomics. 2013;14:30–52.
- Sears MT, Zhang HB, Rushton PJ, et al. NtERF32: a non-NIC2 locus AP2/ERF transcription factor required in jasmonate- inducible nicotine biosynthesis in tobacco. Plant Mol Biol. 2014;84:49–66.
- Charfeddine M, Saıdi MN, Charfeddine S, et al. Genome-wide analysis and expression profiling of the ERF transcription factor family in potato (Solanum tuberosum L.). Mol Biotechnol. 2015;57:348–358.
- Du HW, Huang M, Zhang ZX, et al. Genome-wide analysis of the AP2/ERF gene family in maize water logging stress response. Euphytica. 2014;198:115–126.
- Trupiano D, YordanovY, Regan S, et al. Identification, characterization of an AP2/ERF transcription factor that promotes adventitious, lateral root formation in Populus. Planta. 2013;238:271–282.
- Zhuang J, Cai B, Peng RH, et al. Genome-wide analysis of the AP2/ERF gene family in Populus trichocarpa. BBRC. 2008;371:468–474.
- Zhu QH, Guo AY, Gao G, et al. DPTF: a database of poplar transcription factors. Bioinformatics. 2007;23(10):1307–1308.
- Zhang JY, Wang QJ, Guo ZR. Progress on plant AP2/ERF transcript factor. Hereditas. 2012;34(7): 835–847.
- Jofuku KD, Omidyar PK, Gee Z, et al. Control of seed mass and seed yield by the floral homeotic gene APETALA2. PNAS. 2005;102:3117–3122.
- Hu YX, Wang YX, Liu XF, et al. Arabidopsis RAV1 is down-regulated by brassinosteroid and may act as a negative regulator during plant development. Cell Res. 2004;14(1):8–15.
- Yang Y, Duan X, Ding X, et al. A novel AP2/ERF family transcription factor from Glycine soja, GsERF71, is a DNA binding protein that positively regulates alkaline stress tolerance in Arabidopsis. Plant Mol Biol. 2017;94(4–5):509–530.
- Qiu ZG, Xu ZS, Zheng TH, et al. Screening and identification of proteins interacting with ERF transcription factor W17 in wheat. Acta Agron Sinica. 2011;37:803–810.
- Liu W. Functional research of ethylene response factor ERF4/ ERF72 involved in iron deficiency response of apple rootstocks [D.Ag. thesis]. Beijing: China Agriculture University; 2017.
- Xu JJ, Zhang XF, Xue HW. Rice aleurone layer specific OsNF-YB1 regulates grain filling and endosperm development by interacting with an ERF transcription factor. J Exp Bot. 2016;67:6399–6411.
- Li S, Yin X, Xie X, et al. The Citrus transcription factor, CitERF13, regulates citric acid accumulation via a protein-protein interaction with the vacuolar proton pump, CitVHA-c4. Sci Rep. 2016 [cited 2017 Aug 31];6:20151. DOI: 10.1038/srep20151.
- Yao W, Wang S, Zhou B, et al. Transgenic poplar overexpressing the endogenous transcription factor ERF76 gene improves salinity tolerance. Tree Physiol. 2016;36(7):896–908.
- Wang L. Differential expression and function analysis of genes in Populus simonii×P.nigra under NaCl stress [D.Ag. thesis]. Harbin: Northeast Forestry University; 2010.
- Livak KJ, Schmittgen TD. Analysis of relative gene expression data using real- time quantitative PCR and the 2(-Delta Delta C(T)) Method. Methods. 2001;25(4):402–408.
- Gangola MP, Jaiswal S, Kannan U, et al. Galactinol synthase enzyme activity influences raffinose family oligosaccharides (RFO) accumulation in developing chickpea (Cicer arietinum L.) seeds. Phytochemistry. 2016;125:88–98.
- Nishizawa A, Yabuta Y, Shigeoka S. Galactinol and raffinose constitute a novel function to protect plants from oxidative damage. Plant Physiol. 2008;147:1251–1263.
- Bonawitz ND, Chatenay-Lapointe M, Wearn CM, et al. Expression of the rDNA-encoded mitochondrial protein Tar1p is stringently controlled and responds differentially to mitochondrial respiratory demand and dysfunction. Curr Genet. 2008;54(2):83–94.
- Li L, He Y, Wang Y, et al. Arabidopsis PLC2 is involved in auxin-modulated reproductive development. Plant J. 2015;84:504–515.
- Li L, Wang F, Yan P, et al. A phosphoinositide -specific phospholipase C pathway elicits stress-induced Ca2+ signals and confers salt tolerance to rice. New Phytol. 2017;214(3):1172–1187.
- Yang CL, Liang S, Wang HY, et al. Cotton major latex protein 28 functions as a positive regulator of the ethylene responsive factor 6 in defense against Verticillium dahliae. Mol Plant. 2015;8(3):399–411.
- Litholdo CG, Parker BL, Eamens AL, et al. Proteomic identification of putative microRNA394 target genes in Arabidopsis thaliana identifies MLP family members critical for normal development. Mol Cell Proteomics. 2016;15(6):2033–2047.