ABSTRACT
The aim of this case-control study was to investigate the correlation between the methylation levels of inhibitor of DNA binding 2 (Id2) gene and the incidence of tetralogy of Fallot (TOF) in children. The study included 31 TOF and 32 healthy control children. The methylation status of the Id2 gene was determined with time-of-flight mass spectrometry. Peripheral blood indices were detected, and their correlations with Id2 gene methylation were analyzed. Methylation analysis showed that there was no significant difference in the Id2 gene methylation level between the control and TOF groups (P > 0.05). After controlling the factors of gender, age, height and body weight, the analysis showed that there was no correlation between the methylation levels at each Id2 site and the red blood cells (RBC), hemoglobin (HGB) or hematocrit (HCT) (P > 0.05). However, the methylation levels at the CpG-16 and CpG-18.19 sites of the Id2 gene were negatively correlated with the mean corpuscular hemoglobin (MCH) (r = −0.337, P = 0.009; r = −0.392, P = 0.002) and mean corpuscular hemoglobin concentration (MCHC) (r = −0.363, P = 0.005; r = −0.286, P = 0.028), respectively. Our results suggest that the Id2 methylation might not be associated with the incidence of TOF. These results might contribute to the understanding of the etiology and mechanism of TOF in clinic.
Introduction
Congenital heart disease (CHD) is a common congenital defect in human beings, which ranks the first in the causes of non-infectious death during infancy [Citation1,Citation2]. Tetralogy of Fallot (TOF), a type of CHD, is mainly characterized by pulmonary artery stenosis, ventricular septal defect, aortic overriding, and right ventricular hypertrophy. The mechanism of TOF involves the combination of environmental and genetic factors. Evidence suggests that the mechanism of TOF cannot simply be explained by genetic mutations, but may also imply the accumulation of epigenetic modifications [Citation3–5].
Methylation is a form of epigenetic modification of genomic DNA. Human inhibitor of DNA binding 2 (Id2) gene is located on chromosome 2p25. The Id protein plays a key role in the regulation of various biological processes, including cell differentiation and proliferation, lineage profiling, gene expression, neurogenesis, lymphoid tissue formation and neovascularization [Citation6–8]. Knockout of Id2 in the mouse embryos leads to a variety of cardiac abnormalities, associated with ventricular trabecular damage and ventricular septal defect, obvious outflow tract atresia, and poor endocardial pad maturation. When the Id knockout mouse blastocysts were injected with wild-type embryonic stem cells, the embryonic mortality and cardiac malformations would be reduced [Citation9].
Based on the important role of Id2 in the heart formation process, in this case-control study, the relationship between Id2 and TOF was investigated. The effects of Id2 gene methylation on the incidence of TOF were analyzed and studied. The etiology and mechanism of TOF were also discussed from an epigenetic perspective.
Subjects and methods
Study subjects
Totally, 31 cases of TOF and 32 control subjects, all from Harbin, Heilongjiang, China, were included in this study. For the TOF group, the cases were admitted to the First Affiliated Hospital of Harbin Medical University from January 2013 to December 2014. These children were diagnosed as TOF by ultrasound, cardioangiography or surgical diagnosis. Among these 31 TOF children, there were 23 males and 8 females, with an average age of 16.42 ± 6.25 months (rangeing from 9 to 26 months), average height of 75.42 ± 7.02 cm (ranging from 61 to 88 cm), and average body weight of 9.47 ± 1.64 kg (ranging from 7 to 13 kg).
The control group contained 32 children admitted to the Fourth Affiliated Hospital of Harbin Medical University due to common respiratory tract infection, during the same time period. CHD was excluded for these control subjects based on the physical examination and ultrasound detection. In these 32 control children, there were 23 males and 9 females, with an average age of 17 ± 6.19 months (ranging from 8 to 27 months), average height of 83.63 ± 8.04 cm (ranging from 72 to 100 cm) and average body weight of 12.09 ± 1.96 kg (ranging from 8 to 15 kg). Written informed consent forms were obtained from the subjects’ legal guardians and the study was approved by the Ethics Review Board of the Fourth Affiliated Hospital of Harbin Medical University and the First Affiliated Hospital of Harbin Medical University.
Primer design and synthesis
The potential CpG islands in the human Id2 gene were predicted with an online prediction tool (http://www.ebi.ac.uk/Tools/seqstats/emboss_CpGPlot/). The Sequenom®EPi Designer software was used to design the specific primers for the CpG island-rich region in the promoter of the human Id2 gene. The primers were synthesized by Shanghai Saibaisheng Gene Technology Co., Ltd. ().
Table 1. CpG island-rich region in the promoter for specific amplification of human Id2 gene.
Genomic DNA extraction and methylation determination
For each child from the TOF and control groups, 5 mL peripheral venous blood was collected under fasting conditions in the morning, with vacuum blood collection tubes. The blood samples were subjected to ethylenediaminetetraacetic acid (EDTA) anticoagulation, and then stored at −80 °C. Whole genomic DNA was extracted with the DNA Extraction Kit (DP304; Tiangen, Beijing, China), treated with NaHSO3 and normalized to 10–20 ng/L, followed by short centrifugation at low speed.
For polymerase chain reaction (PCR), the 10 μL reaction system consisted of 1 μL PCR buffer, 1 μL deoxyribonucleoside triphosphates (dNTP; 200 μmol/L), 0.2 μL primer each, 0.1 μL PCR enzyme, 1 μL DNA template and 6.5 μL ddH2O. The reaction conditions were as follows: denaturation at 94 °C for 4 min, and then 94 °C for 20 s, 56 °C for 30 s, and 72 °C for 1 min, for a total of 45 cycles, followed by extension at 72 °C for another 63 min. The PCR products were purified with the Shrimp Alkaline PhosPhatase (SAP). The 2 μL reaction system consisted of 1.7 μL RNase-free ddH2O and 0.30 μL SAP, which was subjected to 37 °C for 20 min and 85 °C for 5 min, and then the reaction was stopped at 4 °C. The product obtained by SAP was subsequently subjected to the T-cut reaction/RNaseA digestion. The solution contained 3.21 μL RNase-free ddH2O, 0.89 μL 5 × T7 Polymerase Buffer, 0.22 μL T Cleavage Mix, 0.22 μL DTT (100 mmol/L), 0.40 μL T7 RNA& DNA Polymerase, and 0.06 μL RNase A. The reaction conditions were as follows: 94 °C for 30 s; 94 °C for 5 s, 56 °C for 5 s (40 cycles), 80 °C for 5 s (5 cycles) and then 72 °C for another 3 min. After being desalted, the final product was sequenced by the MALDI-TOF Mass ARRAY system.
Statistical analysis
Data are expressed as mean values with standard deviation (± SD). SPSS 18.0 software was used for statistical analysis. Student's t- and Pearson χ2 tests were used for the group comparison. After controlling the four factors of gender, age, height and body weight, the methylation levels in CpG islands and peripheral blood indices were analyzed with partial correlation analysis. P < 0.05 was considered to indicate statistical significance.
Results and discussion
Comparison of basic characteristics and clinical data between TOF and control groups
The basic characteristics and clinical data of the TOF and control subjects were first investigated and compared. Our results showed that all the children from the TOF and control groups were form the Chinese Han population. There were 23 males and 8 females in the TOF group, while there were 23 males and 9 males in the control group, with no significant difference in gender between these two groups. Moreover, there were no significant differences in the age between these two groups either (17 ± 6.19 and 16.42 ± 6.25 months for the control and TOF groups, respectively). However, significant differences were observed between the two groups in the height (83.63 ± 8.04 and 75.42 ± 7.02 cm for the control and TOF groups, respectively; P < 0.01), body weight (12.09 ± 1.96 and 9.47 ± 1.64 kg for the control and TOF groups, respectively; P < 0.01), red blood cells (RBC) (4.61 ± 0.41 and 5.82 ± 0.94 × 1012/L for the control and TOF groups, respectively; P < 0.01), hemoglobin (HGB) (120.78 ± 10.07 and 149.32 ± 27.13 g/L for the control and TOF groups, respectively; P < 0.01) and hematocrit (HCT) (36.58 ± 2.90% and 44.67 ± 7.18% for the control and TOF groups, respectively; P < 0.01). In addition, no significant differences were observed in the mean corpuscular volume (MCV), mean corpuscular hemoglobin (MCH) or mean corpuscular hemoglobin concentration (MCHC) between the control and TOF groups (). These results indicate hypoxia status and developmental delay in the children from the TOF group.
Table 2. Basic characteristics and clinical data of TOF and control subjects.
Id2 gene sequencing and methylation status in TOF and control groups
The Id2 gene sequences and methylation status were next analyzed and compared between the TOF and control groups. For the Id2 gene sequence analysis, the target regions of the gene were determined at http: //genome.ucsc.edu/. The gene sequences of the target region were analyzed at http://www.epidesigner.com/, and the Mass ARRAY reaction amplicon was designed. The position relative to the transcription start site was −1000 to 1000 bp, with a length of 597 bp. The amplicon contained 23 CpG sites, and 13 sites could be detected ().
For the Id2 gene methylation analysis, the methylation levels of the −1000 to 1000 bp flanking region of the Id2 transcription start site from the blood samples were detected with time-of-flight mass spectrometry, covering the 23 CpG sites around the transcription start site (CpG_1 to CpG_23). Considering the different roles of the epigenetic alterations in different CpG sites in the Id2 gene in the pathogenesis of TOF, the 13 detectable CpG sites in all these samples were further subjected to cluster analysis (). Our results showed that the methylation levels for these Id2_CpG sites were from 0 to 0.4, with no significant differences between the control and TOF groups. In these 13 CpG sites, the highest methylation level was observed at the CpG_2 site, followed by the CpG_11 and 22, as well as the CpG_11 and 12 site, whereas the lowest methylation level was noted for the CpG_16 site.
Comparison of Id2 gene methylation levels between TOF and control groups
The methylation levels of each Id2_CpG were then analyzed. In these 23 Id2_CpG sites, 13 sites could be detected, whereas the sites of CpG_1, 4, 6, 8, 9, 10, 13, 14, 15, and 20 could not be detected. The site pairs of CpG_11 and 12, CpG_18 and 19, and CpG_21 and 22 shared the same molecular weights, leading to the same MS data based on the average of the two units (). According to the analysis, there were no significant differences in the methylation levels in each Id2_CpG site between the TOF and control groups (), suggesting that the Id2 gene methylation level might not be related to the incidence of TOF.
Table 3. Comparison of methylation levels at Id2_GpG sites.
Correlation analysis between Id2 gene methylation level and peripheral blood erythrocyte indices
Alterations in the DNA methylation levels induced by changed temporal and spatial factors might result in related diseases. Therefore, after controlling the factors of gender, age, height and body weight, the correlation between the Id2 gene methylation levels and peripheral blood erythrocyte indices (including RBC, HGB, HCT, MCV, MCH and MCHC) were investigated. Our results showed no correlation between the Id2_CpG island methylation levels and the RBC, HGB, HCT or MCV. However, the methylation levels of the CpG-16 and CpG-18.19 sites in the Id2 gene were negatively correlated with MCH (r = −0.337, P = 0.009; r = −0.392, P = 0.002; and ) and MCHC (r = −0.363, P = 0.005; r = −0.286, P = 0.028; and ), respectively. Along with the increased methylation levels at the CpG-16 and CpG-18.19 sites of the Id2 gene, decreased serum contents of MCH and MCHC would be observed.
Figure 4. Correlation between the serum content of MCH and the methylation level at the Id2_CpG_16 site.
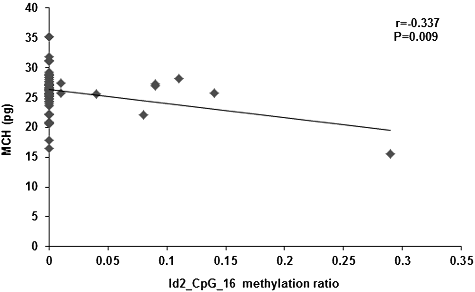
Figure 5. Correlation between the serum content of MCHC and the methylation level at the Id2_CpG_16 site.
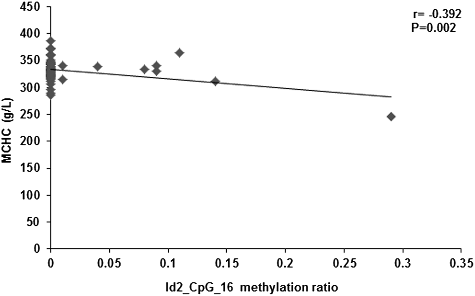
Figure 6. Correlation between the serum content of MCH and the methylation level at Id2_CpG_18.19 site.
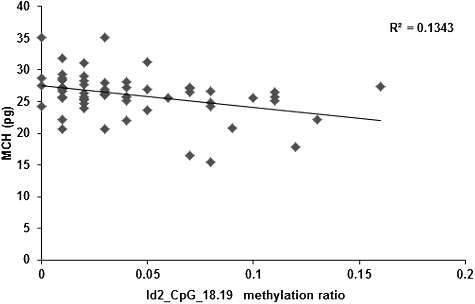
Figure 7. Correlation between the serum content of MCHC and the methylation level at Id2_CpG_18.19 site.
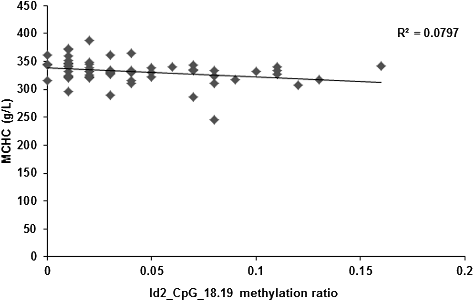
In the present case-control study, the correlation between the Id2 gene methylation and the incidence of TOF was investigated because human Id2 protein belongs to the helix-loop-helix (HLH) family, which inhibits the HLH transcriptional regulators through the HLH domain, and may play a negative regulatory role in the cell differentiation process [Citation10,Citation11]. Studies have reported that the knockout of the Id gene in the mouse embryos would lead to serious heart malformations at as early as E9.5 days, in which the embryonic and heart sizes were reduced by 40%–60%, with changed atrioventricular separation and no ventricular trabecular development [Citation9]. Without the expression of Id proteins, myocardial dysplasia occurred in mouse embryos, suggesting that Id may be involved in the molecular signaling processes between the cardiac muscle, endocardium/epicardium, and endocardial pad during the cardiac development [Citation9].
In the development of chicken hearts, Id2 is reportedly expressed in the cardiac neural crest, second heart field (SHF) outflow/inflow tracts and anterior parasympathetic nerves [Citation12]. Id2 participates in various processes during the heart development, including myogenesis, neurogenesis, cell growth and cell differentiation [Citation13–15]. Removal of Id2 from the cardiac neural crest results in structural defects in the outflow tract (such as the common arterial trunk), and a significant loss would be observed in the Id2 expression in the outflow tract. DNA methylation up-regulates the gene transcription, thus modulating the expression levels of the encoded proteins, which could be transmitted to the next generation through the DNA semiconservative replication [Citation16].
Almost 70%–80% of the mammalian CpG islands are methylated [Citation16–18], in which the CpG islands located in the promoter regions have great influences on the activities of related genes. In this study, the CpG island methylation levels within the −1000 to 1000 bp flanking region of the Id2 transcription start site were analyzed with mass spectrometry. Our results showed that there were no significant differences in the Id2 gene methylation levels between the TOF cases and normal control subjects. These findings suggest that, the cardiac structure development may not involve the altered genetic functions due to the recombination or transposition of Id2. Therefore, we speculate that the Id2 gene methylation level may not be associated with the pathogenesis of TOF, and further in-depth studies are still needed to address this issue concerning other factors.
DNA methylation could be influenced by various factors, including age, gender, height, body weight and environment [Citation19–21]. In this study, the related factors was controlled prior to assessment of the correlation between the methylation levels of each Id2 sites and the RBC, HGB, HCT, MCV, MCH, and MCHC. Our results showed that there was no significant correlation between each CpG site methylation level in the Id2 gene and RBC, HGB, HCT or MCV. However, the methylation levels at the CpG_16 and CpG_18.19 sites were negatively correlated with MCH and MCHC, suggesting that the lower methylation levels at the CpG_16 and CpG_18.19 sites were associated with higher levels of MCH and MCHC. Based on these findings, we hypothesize that the methylation levels of Id2_CpG_16 and CpG_18.19 sites could influence the MCH and MCHC level in peripheral blood in children suffering from TOF.
In addition, for the TOF children, the aorta receives the venous blood from the right ventricle, which, together with the reduced blood flow for gas change due to pulmonary artery stenosis, always causes hypoxia. The long-term hypoxia would further delay the development of the TOF children (such as height and body weight). In this case, the blood levels of RBC, HGB and HCT will be increased to alleviate the hypoxia status, which might happen in various hypoxia conditions, such as in people with lung diseases and who live at high altitude [Citation22,Citation23]. The blood levels of both RBC and HGB in the TOF group are higher than the control group, suggesting that TOF represents a hypoxic heart disease. More red blood cells and higher content of hemoglobin are associated with greater maximum oxygen uptake in the body, which could alleviate the hypoxic status. Id2 inhibits the cell differentiation and promotes the cell proliferation, and it can promote the development of red blood cells [Citation24,Citation25]. The down-regulated Id2 expression inhibits the growth and maturation of red blood cells, while over-expressed Id2 may accelerate the development of red blood cells [Citation26]. The mechanism may be related to the promotion of PU.1 and GATA-1 functions. In fact, the normal expression of PU.1 and GATA-1 is a necessary condition for the hematopoietic development [Citation27]. Based on these findings, we hypothesize that along with the declined methylation levels at the Id2 CpG_16 and CpG_18.19 sites, the expression of Id2 is increased, which promotes the development of the erythrocyte system, increasing the hemoglobin level and leading to the negative correlation between the Id2 methylation level and MCH/MCHC contents.
There have been studies concerning the relationship between the single nucleotide polymorphisms of EPAS1/HIF2A and EGLN1/ PHD2 genes and the blood cell indices in hypoxic diseases [Citation28,Citation29], which however have not involved Id2 methylation. Due to the limitations from the ethics concerns, only blood samples were collected from the child subjects. In fact, because of the tissue specificity of gene methylation, it would be more conceivable if the myocardial tissue samples could be used. In addition, no significant differences were observed between these two groups, which might be associated with the limited sample size and/or experimental method. Therefore, further in-depth studies with a larger cohort size and optimized detection methods are still need to investigate the mechanism and pathogenesis of TOF in future.
Conclusions
Our results suggest that Id2 gene methylation might not be associated with the incidence of TOF. However, the methylation levels of the Id2 CpG_16 and CpG_18.19 sites were negatively related to MCH and MCHC contents, which could be influenced by the methylation level of these two Id2 sites in peripheral blood in children suffering from TOF. These results might contribute to the understanding of the etiology and mechanism of TOF in clinic.
Disclosures statement
All authors declare no financial competing interests.
All authors declare no non-financial competing interests.
Acknowledgements
This research was supported by the Natural Science Foundation of Heilongjiang Province under grant number H201412 and the Postdoctoral Fund of Heilongjiang Province under grant number 2013LBH-Z13152.
Additional information
Funding
References
- Blue GM, Kirk EP, Sholler GF, et al. Congenital heart disease: current knowledge about causes and inheritance. Med J Aust. 2012;197(3):155–159.
- Matsuda H, Ichikawa H, Ueno T, et al. Heart transplantation for adults with congenital heart disease: current status and future prospects. Gen Thorac Cardiovasc Surg. 2017;65(6):309–320.
- Sheng W, Qian Y, Wang H, et al. DNA methylation status of NKX2-5, GATA4 and HAND1 in patients with tetralogy of fallot. BMC Med Genomics. 2013;6:46.
- Grunert M, Dorn C, Cui H, et al. Comparative DNA methylation and gene expression analysis identifies novel genes for structural congenital heart diseases. Cardiovasc Res. 2016;112(1):464–477.
- Sheng W, Chen L, Wang H, et al. CpG island shore methylation of ZFPM2 is identified in tetralogy of fallot samples. Pediatr Res. 2016;80(1): 151–158.
- Kiewitz SD, Cabrele C. Synthesis and conformational properties of protein fragments based on the Id family of DNA-binding and cell-differentiation inhibitors. Biopolymers. 2005;80(6):762–774.
- Zebedee Z, Hara E. Id proteins in cell cycle control and cellular senescence. Oncogene. 2001;20(58):8317–8325.
- Norton JD. ID helix-loop-helix proteins in cell growth, differentiation and tumorigenesis. J Cell Sci. 2000;113(Pt 22):3897–3905.
- Fraidenraich D, Stillwell E, Romero E, et al. Rescue of cardiac defects in Id knockout embryos by injection of embryonic stem cells. Science. 2004;306(5694):247–252.
- Benezra R, Davis RL, Lockshon D, et al. The protein Id: a negative regulator of helix-loop-helix DNA binding proteins. Cell. 1990;61(1):49–59.
- Massari ME, Murre C. Helix-loop-helix proteins: regulators of transcription in eucaryotic organisms. Mol Cell Biol. 2000;20(2):429–440.
- Martinsen BJ, Frasier AJ, Baker CV, et al. Cardiac neural crest ablation alters Id2 gene expression in the developing heart. Dev Biol. 2004;272(1):176–190.
- Jongbloed MR, Vicente-Steijn R, Douglas YL, et al. Expression of Id2 in the second heart field and cardiac defects in Id2 knock-out mice. Dev Dyn. 2011;240(11):2561–2577.
- Duffield GE, Watson NP, Mantani A, et al. A role for Id2 in regulating photic entrainment of the mammalian circadian system. Curr Biol. 2009;19(4):297–304.
- Jen Y, Manova K, Benezra R. Expression patterns of Id1, Id2, and Id3 are highly related but distinct from that of Id4 during mouse embryogenesis. Dev Dyn. 1996;207(3):235–252.
- Caiafa P, Zampieri M. DNA methylation and chromatin structure: the puzzling CpG islands. J Cell Biochem. 2005;94(2): 257–265.
- Colyer HA, Armstrong RN, Sharpe DJ, et al. Detection and analysis of DNA methylation by pyrosequencing. Methods Mol Biol. 2012;863:281–292.
- Takahashi Y, Wu J, Suzuki K, et al. Integration of CpG-free DNA induces de novo methylation of CpG islands in pluripotent stem cells. Science. 2017;356(6337):503–508.
- Geng Y, Gao R, Chen X, et al. Folate deficiency impairs decidualization and alters methylation patterns of the genome in mice. Mol Hum Reprod. 2015;21(11):844–856.
- Choi SW, Tammen SA, Liu Z, et al. A lifelong exposure to a Western-style diet, but not aging, alters global DNA methylation in mouse colon. Nutr Res Pract. 2015;9(4):358–363.
- Grosser C, Wagner N, Grothaus K, et al. Altering TET dioxygenase levels within physiological range affects DNA methylation dynamics of HEK293 cells. Epigenetics. 2015;10(9):819–833.
- Ding K, de Andrade M, Manolio TA, et al. Genetic variants that confer resistance to malaria are associated with red blood cell traits in African-Americans: an electronic medical record-based genome-wide association study. G3 (Bethesda). 2013;3(7):1061–1068.
- Liu B, Wang Z, Lu J. Response to chronic intermittent hypoxia in blood system of Mandarin vole (Lasiopodomys mandarinus). Comp Biochem Physiol A Mol Integr Physiol. 2010;156(4):469–474.
- D'Cruz LM, Stradner MH, Yang CY, et al. E and Id proteins influence invariant NKT cell sublineage differentiation and proliferation. J Immunol. 2014;192(5):2227–2236.
- Li H, Ji M, Klarmann KD, et al. Repression of Id2 expression by Gfi-1 is required for B-cell and myeloid development. Blood. 2010;116(7):1060–1069.
- Yokota Y, Mori S, Narumi O, et al. In vivo function of a differentiation inhibitor, Id2. IUBMB Life. 2001;51(4):207–214.
- Ji M, Li H, Suh HC, et al. Id2 intrinsically regulates lymphoid and erythroid development via interaction with different target proteins. Blood. 2008;112(4):1068–1077.
- Ronen R, Zhou D, Bafna V, et al. The genetic basis of chronic mountain sickness. Physiology (Bethesda). 2014;29(6):403–412.
- Beall CM, Cavalleri GL, Deng L, et al. Natural selection on EPAS1 (HIF2alpha) associated with low hemoglobin concentration in Tibetan highlanders. Proc Natl Acad Sci USA. 2010;107(25):11459–11464.