Abstract
Histone deacetylases (HDACs) catalyse the deacetylation of core histones and non-histone proteins. Histone deacetylases work in concert with histone acetyltransferases to modify the structure and function of chromatins, and regulate gene transcription. Histone deacetylases are critical enzymes involved in the regulation of multiple cellular processes, such as plant growth and development, stress responses and gene silencing. Plant HDACs are a supergene family and can be divided into three families, namely RPD3/HDA1, HD2 and SIR2. HD2 specifically occur in plants, not in fungi and animals. In this study, an HD2-type HDAC gene, PtHDT903, was cloned from Populus trichocarpa. Its amino acid sequence, subcellular localization and expression patterns under abiotic stresses were analysed. The results showed that PtHDT903 encodes a hydrophilic and acidic protein consisting of 305 amino acids. The predicted PtHDT903 protein has the conserved domains which found in the other HD2-type HDACs. The PtHDT903 protein was localized in the nucleus. The expression of the PtHDT903 gene was down-regulated by salt stress, whereas it was up-regulated by cold. The results provide valuable information for the further functional study of PtHDT903.
Introduction
Multiple mechanisms regulate gene expression. One of them is epigenetic regulation, which is fast, reversible and inheritable [Citation1]. The epigenetic regulation mechanism includes DNA methylation, histone modifications, small and non-coding RNAs, and chromatin architecture. Among the histone modifications, histone acetylation has been studied much earlier and in greater detail [Citation2]. Histone acetylation is regulated by histone acetyltransferases (HATs) and histone deacetylases (HDACs). HATs catalyse histone acetylation, which is associated with expanded chromatin structure. HDACs catalyse the removal of acetyl groups from chromatin, leading to contact chromatin structure, and thus are considered to be transcription repressors. Both HATs and HDACs are important for the regulation of gene expression. Recently, HDACs attract more and more attention.
HDACs are a supergene family. Based on sequence homology to yeast HDACs, plant HDACs can be classified into three families, namely reduced potassium dependency 3/histone deacetylase 1 (RPD3/HDA1), histone deacetylase 2 (HD2) and silent information regulator 2 (SIR2). RPD3/HDA1 includes a large number of members and the family proteins are Zn2+-dependent [Citation3, Citation4]. HD2 are plant specific and the first HD2 protein was identified in maize embryos [Citation5–10]. SIR2 catalyse NAD+-dependent deacetylation from histones and non-histone proteins [Citation11, Citation12]. The studies in herbaceous plants, including maize, Arabidopsis and rice, show that HDACs play an important role in development, stress responses and gene silencing [Citation13–33]. The aberrant expression of HD2 family genes alters the leaf morphology, flowering and seed development [Citation16, Citation19, Citation34–37]. The over-expression of AtHD2C in Arabidopsis can reduce the transpiration and increase the tolerance of transgenic plants to salt and drought [Citation25]. In rice, the over-expression of HDT701 can enhance the salt and osmotic stress tolerance during the seedling stage [Citation38]. To date, however, the expression and function of HD2 in cold stress is less known.
In plants, the studies on HD2 are just at the beginning. The expression and function of HD2 family genes in woody plants are still not known. In this study, a gene encoding an HD2-type HDAC, PtHDT903, was cloned from Populus trichocarpa. The amino acid sequence, subcellular localization and expression of PtHDT903 under various types of stress were investigated in detail.
Materials and methods
Plant growth and stress treatment
We used 4-week-old seedlings of poplar (P. trichocarpa) grown at 25 °C under the 16-h light/8-h dark conditions. The stress treatments were salt and cold. For salt treatment, we supplemented the seedlings with 200 mmol/L NaCl in vermiculite for 0, 12 and 48 h. For cold treatment, we incubated the seedlings at 4 °C for 0, 1 and 3 days. After cold treatment for 3 days, we allowed the seedlings to recover for 2 days at 25 °C. After salt and cold treatments, we collected the leaves, stems and roots of the seedlings for RNA isolation and gene expression analyses. The presented data are mean values with standard deviation from three independent treatment experiments with nine plants per treatment.
Cloning and sequence analysis of PtHDT903
Based on the nucleic acid sequence of PtHDT903 in the Chromdb database (http://www.chromdb.org/) and the National Center for Biotechnology Information (NCBI) database, the Open Reading Frame (ORF) of the HDT903 gene in P. trichocarpa was cloned by reverse transcription-polymerase chain reaction (RT-PCR). The cDNAs were synthesized with PrimeScript RT reagent Kit (TaKaRa), using the total RNAs from young seedlings. The specific primers were designed and the primer sequences were as follows: 5′-ATGGAGTTCTGGGGTGTTGA-3′ and 5′-CTATGCAGCACTGTGCTTAG-3′. The molecular weight (MW) and the theoretical isoelectric point (pI) of the PtHDT903 protein were determined using ExPASy (Expert Protein Analysis System) ProtParam software (http://web.expasy.org/protparam/). The PtHDT903 homologous proteins were obtained by blasting NCBI. The homologous proteins include P. euphratica PeHDT1 (XP 011018878), Herrania umbratica HuHDT1 (XP 021289379), Sesamum indicum SiHDT1 (XP 011101488), Jatropha curcas JcHDT1 (XP 012076192), Manihot esculenta MeHDT1 (XP 021610077), Arachis ipaensis AiHDT1 (XP 016208108) and Cajanus cajan CcHDT1 (XP 020211823). The amino acid sequences of PtHDT903 and its homologous proteins were aligned using ClustalX 1.83 [Citation39] and refined with Genedoc [Citation40]. The amino acid sequence identity among the eight HD2 was analysed using DNAMAN 7.0. The neighbour-joining phylogenetic tree was constructed using the MEGA 5 program [Citation41] with a bootstrap analysis of 1000 replicates. The conserved domain of PtHDT903 was determined using the SMART program (http://smart.embl-heidelberg.de/) and by searches in the Conserved Domain Database (CDD, http://www.ncbi.nlm.nih.gov/Structure/cdd/wrpsb.cgi).
Subcellular localization analysis
The ORF fragment of PtHDT903 was fused to the 5′ end of the green fluorescent protein (GFP) reporter gene in the vector pCAMBIA1302. The recombinant plasmid containing the fusion gene (HDA903-GFP) and the vector (GFP) were respectively transformed into the epidermal cells of onion by particle bombardment using PDS-1000/He (BIO-RAD). After incubation in 1/2 Murashige and Skoog (MS) medium at 28 °C for 24 h, GFP fluorescence was observed under a confocal laser-scanning microscope (LSM700; Carl Zeiss).
Gene expression analysis
The total RNAs from leaves, stems and roots of P. trichocarpa seedlings were prepared using Trizol reagent (Invitrogen) and cDNA were obtained using PrimeScript RT reagent Kit (TaKaRa). The real-time PCR was set up using SYBR Premix Ex Taq II Kit (TaKaRa) in a volume of 20 µL. The reaction procedure was as follows: 95 °C for 5 min, followed by 44 amplification cycles at 95 °C for 30 s, 60 °C for 30 s and 72 °C for 30 s. The primers for PtHDT903 were: 5′-CAAAGTTGCTCAACCCGAAT-3′ and 5′-TGGTTGCTTTGCCTCTTCTT-3′. The 18S rRNA (18S) was used as the internal control. For organ-specific expression analysis, the expression level of PtHDT903 gene was calculated by the 2-ΔCt method [Citation42] and presented as 106×2-ΔCt. The expression level of PtHDT903 under salt and cold stresses was determined using 2-ΔΔCt calculations. The transcript level of PtHDT903 without cold and salt treatments was indicated as 1. The transcript levels (n-fold) of PtHDT903 under cold and salt stresses were relative to that without any treatments. The data were statistically analysed using one-way analysis of variance (ANOVA) and Tukey test. The significance level was set to p < 0.05.
Results and discussion
Cloning, sequence and organ specific expression analysis of PtHDT903
In our study, we cloned an HD2-type HDAC gene PtHDT903 from P. trichocarpa (GenBank accession No. EF145859). The ORF of the cloned PtHDT903 is 918 bp in length, encoding a hydrophilic protein consisting of 305 amino acid residues. Its molecular mass is 33.35 kDa, and its isoelectric point is 4.61.
PtHDT903 has the closest relationship in evolution with PtHdT1 from P. euphratica () and their amino acid sequence identity was 97.8%. The amino acid sequences of PtHDT903 and the other seven HD2 proteins were highly conserved (). In the N-terminal region of the predicted PtHDT903 protein, there is an invariable pentapeptide motif (MEFWG) and two conserved amino acid residues, a histidine at position 25 and an aspartate at position 70, which are postulated to be critical for HDAC catalytic activity [Citation43]. The central part of PtHDT903, as in the other HD2 proteins [Citation6, Citation22, Citation35, Citation44], is an extended acidic region enriched in Asp and Glu residues (position 162 to 208). The acidic region might be involved in nucleolar localization or association with basic tails of histones [Citation45]. The C-terminal region of PtHDT903 contains a nuclear localization signal (KKAK) and a C2H2-type zinc finger domain. The single zinc finger might be involved in protein–protein interactions [Citation46]. In addition to the C2H2 zinc finger (position 279 to 302), the predicted PtHDT903 also has two Low Complexity domains, from amino acids 159 to 177 and from 185 to 206 ().
Figure 1. Phylogenetic analysis of HD2-type histone deacetylases. Note: The phylogenetic tree is based on amino acid sequences of PtHDT903 and its homologous proteins. The tree was drawn to scale (scale bar, 0.05 amino acid substitutions per site). The eight HD2 proteins are Populus trichocarpa PtHDT903, Populus euphratica PeHDT1, Herrania umbratica HuHDT1, Sesamum indicum SiHDT1, Jatropha curcas JcHDT1, Manihot esculenta MeHDT1, Arachis ipaensis AiHDT1, and Cajanus cajan CcHDT1.
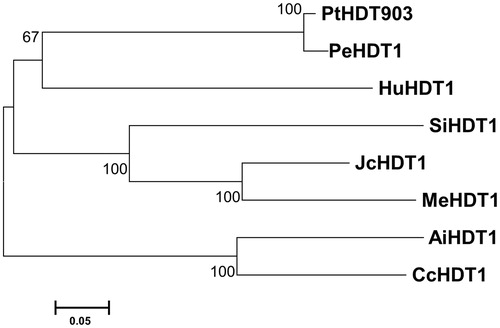
Figure 2. Amino acid sequence alignment of HD2-type histone deacetylases. Note: Amino acid sequences of PtHDT903 and its homologous proteins were aligned. Letters indicate: (a) invariable pentapeptide motif (MEFWG); (b) critical amino acid residues for histone deacetylase catalytic activity; (c) nuclear localization signal (KKAK); (d) conserved C2H2-type zinc finger domain. The extended acidic domain is underlined.
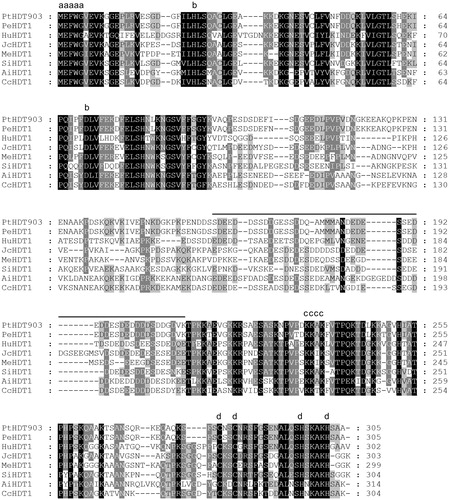
Figure 3. Conserved domains of PtHDT903 (A) and its expression in various organs (B). Note: Grey box represents the low complexity domains; black box represents the C2H2-type zinc finger domain. The mRNA levels of PtHDT903 in leaves, stems and roots were analysed using real-time PCR.
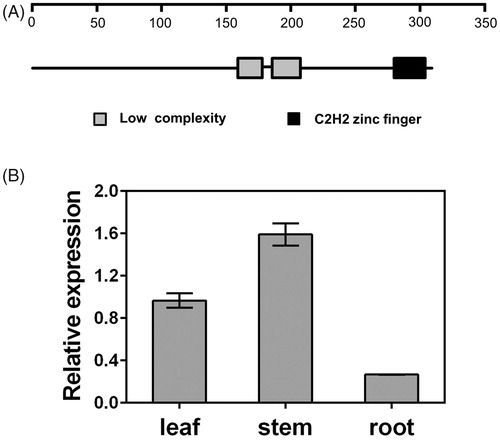
The PtHDT903 gene could be expressed in all the organs examined, but at different levels. Its expression levels in leaves and stems were high, while its expression in roots was relatively low ().
Subcellular localization of PtHDT903
To know the subcellular localization of the PtHDT903 protein, the HDT903-GFP infusion gene was transformed into the epidermal cells of onion. In the onion cells transformed with GFP alone, the green fluorescence was distributed throughout the cells (), while in the cells transformed with HDA903-GFP, the fluorescent signals were only detected in the nucleus (). The data showed that PtHDT903 is localized in the nucleus. In our experiment, the GFP fluorescence could be detected in some cells. The lack of fluorescence in the surrounding cells might be due to the low transformation efficiency of onion cells.
Figure 4. Sub-cellular localization of PtHDT903 in epidermal onion cells transformed with the vector containing the GFP reporter gene (A–C) and the HDT903-GFP infusion gene (D–F). Note: The photographs were taken in the dark field for green fluorescence (A and D), under bright light for cell morphology (B and E) and in combination (C and F). Scale bars: 100 µm.
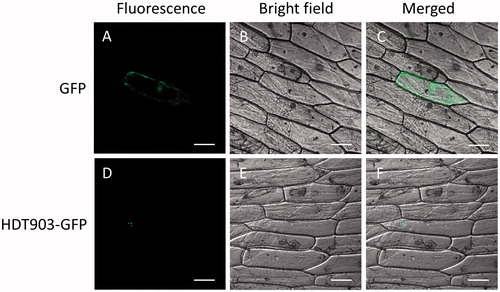
Expression patterns of PtHDT903 under abiotic stresses
We analysed the expression patterns of the PtHDT903 gene under salt and cold stresses. The results showed that the expression of PtHDT903 was regulated by both salt and cold treatment, but the expression patterns were different under both stresses. Salt stress treatment with 200 mmol/L NaCl for 48 h resulted in down-regulation of the PtHDT903 expression in leaves and stems, but not in roots (). After such a treatment, the expression levels of PtHDT903 in the leaves and stems were 84.7 and 78.7% down-regulated, respectively. This result was consistent with the findings in rice and Arabidopsis. In rice, the expression level of OsHDT701 steadily decreased after 300 mmol/L NaCl treatment for 1 and 3 h [Citation38]. In Arabidopsis, the expression levels of the four genes in the HD2 family, HD2A, HD2B, HD2C and HD2D, were significantly down-regulated after 250 mmol/L NaCl treatment for 6 h [Citation47].
Figure 5. Expression patterns of PtHDT903 under salt (A–C) and cold (D–F) stress in leaves (A and D), stems (B and E) and roots (C and F). Note: Real-time PCR analysis. Data represent mean values with standard deviations (±SD) of three independent experiments. *p < 0.05, **p < 0.01, ***p < 0.001 and ****p < 0.0001, significant difference between the treated and control samples.
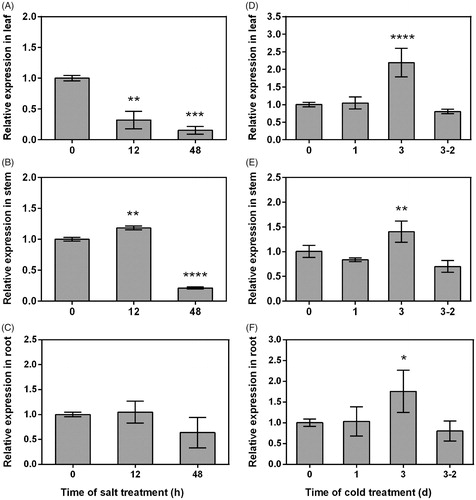
In plants, the expression of HD2 family genes under cold stresses is not well understood and few data on their expression are available. The data available in rice show that the expression of the OsHDT701 gene was not altered after cold (4 °C) treatment for 1 day [Citation21]. In our study, the expression of PtHDT903 was not altered after cold (4 °C) treatment for 1 day, but was significantly induced after long-term cold treatment (3 days). After cold treatment for 3 days, the PtHDT903 expression in the leaves, stems and roots was 2.2-, 1.4- and 1.8-fold up-regulated, respectively. After recovery for 2 days following cold treatment for 3 days, the expression of PtHDT903 in the leaves, stems and roots was almost the same as that before cold treatment. Taken together, the expression of PtHDT903 in poplar seedlings was repressed by salt, but was induced by cold. The different responses of PtHDT903 to salt and cold stimuli suggest that the up-stream regulators or down-stream target genes of PtHDT903 might be different under salt and cold stress conditions.
Conclusions
In our work, an HD2-type HDAC gene PtHDT903 was cloned from P. trichocarpa. The PtHDT903 protein was predicted to contain three conserved domains. The PtHDT903 protein is exclusively localized in the nucleus. The expression of PtHDT903 gene is responsive to abiotic stresses. Its expression was repressed by salt, but was induced by cold. Our findings provide valuable information for further functional study of PtHDT903, which might be potentially applied for the cultivation of stress-tolerant poplar varieties.
Disclosure statement
No potential conflict of interest was reported by the authors.
Additional information
Funding
References
- Boyko A, Kovalchuk I. Genome instability and epigenetic modification–heritable responses to environmental stress? Curr Opin Plant Biol. 2011;14:260–266.
- Allfrey VG, Faulkner R, Mirsky AE. Acetylation and methylation of histones and their possible role in the regulation of RNA synthesis. Proc Natl Acad Sci USA. 1964;51:786–794.
- Finnin MS, Donigian JR, Cohen A, et al. Structures of a histone deacetylase homologue bound to the TSA and SAHA inhibitors. Nature. 1999;401:188–193.
- Marmorstein R. Structure of histone deacetylases: insights into substrate recognition and catalysis. Structure. 2001;9:1127–1133.
- Brosch G, Lusser A, Goralik-Schramel M, et al. Purification and characterization of a high molecular weight histone deacetylase complex (HD2) of maize embryos. Biochemistry. 1996;35:15907–15914.
- Lusser A, Brosch G, Loidl A, et al. Identification of maize histone deacetylase HD2 as an acidic nucleolar phosphoprotein. Science. 1997;277:88–91.
- Farhi J, Tian G, Fang H, et al. Histone deacetylase HD2D is involved in regulating plant development and flowering time in Arabidopsis. Plant Signal Behav. 2017;12:e1300742. doi:10.1080/15592324.2017.1300742
- Kuang JF, Chen JY, Luo M, et al. Histone deacetylase HD2 interacts with ERF1 and is involved in longan fruit senescence. J Exp Bot. 2012;63:441–454.
- Grandperret V, Nicolas-Frances V, Wendehenne D, et al. Type-II histone deacetylases: elusive plant nuclear signal transducers. Plant Cell Environ. 2014;37:1259–1269.
- Liu X, Yang S, Zhao M, et al. Transcriptional repression by histone deacetylases in plants. Mol Plant. 2014;7:764–772.
- Grubisha O, Smith BC, Denu JM. Small molecule regulation of Sir2 protein deacetylases. FEBS J. 2005;272:4607–4616.
- Denu JM. The Sir 2 family of protein deacetylases. Curr Opin Chem Biol. 2005;9:431–440.
- Ma X, Lv S, Zhang C, et al. Histone deacetylases and their functions in plants. Plant Cell Rep. 2013;32:465–478.
- Chinnusamy V, Zhu JK. Epigenetic regulation of stress responses in plants. Curr Opin Plant Biol. 2009;12:133–139.
- Chen ZJ, Tian L. Roles of dynamic and reversible histone acetylation in plant development and polyploidy. Biochim Biophys Acta. 2007;1769:295–307.
- Zhou C, Labbe H, Sridha S, et al. Expression and function of HD2-type histone deacetylases in Arabidopsis development. Plant J. 2004;38:715–724.
- Rossi V, Locatelli S, Varotto S, et al. Maize histone deacetylase hda101 is involved in plant development, gene transcription, and sequence-specific modulation of histone modification of genes and repeats. Plant Cell. 2007;19:1145–1162.
- Hollender C, Liu Z. Histone deacetylase genes in Arabidopsis development. J Integr Plant Biol. 2008;50:875–885.
- Hu Y, Qin F, Huang L, et al. Rice histone deacetylase genes display specific expression patterns and developmental functions. Biochem Biophys Res Commun. 2009;388:266–271.
- Varotto S, Locatelli S, Canova S, et al. Expression profile and cellular localization of maize Rpd3-type histone deacetylases during plant development. Plant Physiol. 2003;133:606–617.
- Fu W, Wu K, Duan J. Sequence and expression analysis of histone deacetylases in rice. Biochem Biophys Res Commun. 2007;356:843–850.
- Demetriou K, Kapazoglou A, Tondelli A, et al. Epigenetic chromatin modifiers in barley: I. Cloning, mapping and expression analysis of the plant specific HD2 family of histone deacetylases from barley, during seed development and after hormonal treatment. Physiol Plant. 2009;136:358–368.
- Chen LT, Luo M, Wang YY, et al. Involvement of Arabidopsis histone deacetylase HDA6 in ABA and salt stress response. J Exp Bot. 2010;61:3345–3353.
- Chen LT, Wu K. Role of histone deacetylases HDA6 and HDA19 in ABA and abiotic stress response. Plant Signal Behav. 2010;5:1318–1320.
- Sridha S, Wu K. Identification of AtHD2C as a novel regulator of abscisic acid responses in Arabidopsis. Plant J. 2006;46:124–133.
- Zhu J, Jeong JC, Zhu Y, et al. Involvement of Arabidopsis HOS15 in histone deacetylation and cold tolerance. Proc Natl Acad Sci USA. 2008;105:4945–4950.
- To TK, Nakaminami K, Kim JM, et al. Arabidopsis HDA6 is required for freezing tolerance. Biochem Biophys Res Commun. 2011;406:414–419.
- Wu K, Zhang L, Zhou C, et al. HDA6 is required for jasmonate response, senescence and flowering in Arabidopsis. J Exp Bot. 2008;59:225–234.
- Zhou C, Zhang L, Duan J, et al. HISTONE DEACETYLASE19 is involved in jasmonic acid and ethylene signaling of pathogen response in Arabidopsis. Plant Cell. 2005;17:1196–1204.
- Kim KC, Lai Z, Fan B, et al. Arabidopsis WRKY38 and WRKY62 transcription factors interact with histone deacetylase 19 in basal defense. Plant Cell. 2008;20:2357–2371.
- Hu Y, Zhang L, Zhao L, et al. Trichostatin A selectively suppresses the cold-induced transcription of the ZmDREB1 gene in maize. PLoS One. 2011;6:e22132. doi:10.1371/journal.pone.0022132
- Guo JE, Hu Z, Guo X, et al. Molecular characterization of nine tissue-specific or stress-responsive genes of histone deacetylase in tomato (Solanum lycopersicum). J Plant Growth Regul. 2017;36:566–577.
- Hartl M, Füszl M, Boersema PJ, et al. Lysine acetylome profiling uncovers novel histone deacetylase substrate proteins in Arabidopsis. Mol Syst Biol. 2017;13(10):949. doi:10.15252/msb.20177819
- Li C, Huang L, Xu C, et al. Altered levels of histone deacetylase OsHDT1 affect differential gene expression patterns in hybrid rice. PLoS One. 2011;6:e21789. doi:10.1371/journal.pone.0021789
- Wu K, Tian L, Malik K, et al. Functional analysis of HD2 histone deacetylase homologues in Arabidopsis thaliana. Plant J. 2000;22:19–27.
- Han Z, Yu H, Zhao Z, et al. AtHD2D gene plays a role in plant growth, development, and response to abiotic stresses in Arabidopsis thaliana. Front Plant Sci. 2016;7:310. doi:10.3389/fpls.2016.00310
- Colville A, Alhattab R, Hu M, et al. Role of HD2 genes in seed germination and early seedling growth in Arabidopsis. Plant Cell Rep. 2011;30:1969–1979.
- Zhao J, Zhang J, Zhang W, et al. Expression and functional analysis of the plant-specific histone deacetylase HDT701 in rice. Front Plant Sci. 2014;5:764. doi:10.3389/fpls.2014.00764
- Thompson JD, Gibson TJ, Plewniak F, et al. The CLUSTAL_X windows interface: flexible strategies for multiple sequence alignment aided by quality analysis tools. Nucleic Acids Res. 1997;25:4876–4882.
- Nicholas KB, Nicholas HB, Jr. GeneDoc: a tool for editing and annotating multiple sequence alignments. Software distributed by the authors; 1997.
- Tamura K, Peterson D, Peterson N, et al. MEGA5: molecular evolutionary genetics analysis using maximum likelihood, evolutionary distance, and maximum parsimony methods. Mol Biol Evol. 2011;28:2731–2739.
- Livak KJ, Schmittgen TD. Analysis of relative gene expression data using real-time quantitative PCR and the 2(-Delta Delta C(T)) Method. Methods. 2001;25:402–408.
- Aravind L, Koonin EV. Second family of histone deacetylases. Science. 1998;280:1167. doi:10.1126/science.280.5367.1167a
- Bourque S, Dutartre A, Hammoudi V, et al. Type-2 histone deacetylases as new regulators of elicitor-induced cell death in plants. New Phytol. 2011;192:127–139.
- Philpott A, Leno GH. Nucleoplasmin remodels sperm chromatin in Xenopus egg extracts. Cell. 1992;69:759–767.
- Dangl M, Brosch G, Haas H, et al. Comparative analysis of HD2 type histone deacetylases in higher plants. Planta. 2001;213:280–285.
- Luo M, Wang YY, Liu X, et al. HD2C interacts with HDA6 and is involved in ABA and salt stress response in Arabidopsis. J Exp Bot. 2012;63:3297–3306.