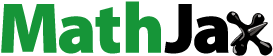
ABSTRACT
Antibodies and antagonists targeting tumour necrosis factor alpha (TNFα) have become a number of the best-selling anti-inflammatory drugs. The nanobody is a single domain antibody derived from the variable domain of a heavy-chain antibody. However, so far, there are still no reports on the recombinant expression of TNFα nanobodies. Here, TNF30, the key anti-TNFα nanobody domain of ozoralizumab, was cloned, expressed in Escherichia coli, and then purified with the Ni2+-chelating affinity chromatography. The western blot results showed that the recombinant TNF30 has strong binding capability with TNFα. Furtermore, the pharmacological and histopathological analysis in the carrageenan-induced mice paw oedema demonstrated that the recombinant nanobodies had potential anti-inflammatory activity in vivo. These works provided a novel approach for the production of TNF30 in E. coli, and might establish a foundation for the industrial production of the drug and the development of more active derivatives against TNFα in the future.
Human tumour necrosis factor alpha (TNFα) is a pleiotropic cytokine, which, on the one hand, participates in haemopoiesis, immunological surveillance and protection from bacterial infection and, on the other hand, plays a role in many diseases, such as rheumatoid arthritis (RA), transplant rejection and tumorigenesis [Citation1,Citation2]. Antibodies and antagonists targeting TNFα have become a number of the best-selling drugs against rheumatoid arthritis and ankylosing spondylitis. In 2014, the total sales of three TNFα antibodies, Humira, Remicade and Enbrel, jumped 7.8% from 2013 to $30.3 billion [Citation3]. Now, Humira has been the most lucrative drug in the world, and it had $18.4 billion of global sales in 2017.
The nanobody is a single domain antibody derived from the variable domain of a heavy-chain antibody [Citation4]. Comparing with the conventional monoclonal antibody, the nanobody has some special advantages. First, the nanobody has a smaller size, which endows it with high penetration capability through the cell membrane [Citation5]. Second, the elongated complementarity-determining region 3 (CDR3) facilitates the nanobody to contact with epitopes which hide into the concave surface of an antigen [Citation4]. Third, the single-strand polypeptide of the nanobody is suitable for obtaining by phage display and production via genetic engineering [Citation6,Citation7]. Because of these characteristics, nanobodies have been widely considered as therapeutic or diagnostic candidates, enzyme inhibitors and protein crystallised helpers, and are gaining increasing attention [Citation4,Citation8,Citation9].
Up to now, there are still no literature reports on the recombinant expression of TNFα nanobodies. Ozoralizumab is a trimeric bispecific nanobody which has completed phase II clinical trials in patients with RA [Citation10]. Ozoralizumab consists of two VHHs directed against TNFα and one albumin-binding VHH [Citation11]. In the present study, TNF30, the key anti-TNFα domain of ozoralizumab, was cloned and expressed in Escherichia coli. Its affinity to TNFα and its bioactivity on inflammation in vivo were also investigated.
Materials and methods
Cloning of TNF30 and construction of recombinant plasmid
DNA encoding TNF30 was optimised according to the codon preference of E. coli and synthesised. To construct the expression plasmid for TNF30, firstly, the gene encoding TNF30 was amplified using polymerase chain reaction (PCR) with primer EC01 (5′-ATCGAAGGTCGTGAAGTTCAACTTGTTGAATCAGG-3′) and EC02 (5′-GCGAGGAGCTCATTATGATGAAACAGTAACAAGAG-3′). A hexahistidine (His6) tag sequence followed by a Factor Xa protease recognition site was obtained by PCR using primer EC03 (5′-AACTTCACGACCTTCGATGCCGCTGCTGTGATGATG-3′) and EC04 (5′-AGCTACATCACTCAGACTTCAGCAACCGCACCTGTG-3′). The products of these two PCR amplifications were then mixed to use as a new template and were amplified with primer EC02 and EC04 to generate a fused gene. The obtained PCR product was digested with NotI and SacI and inserted into pET22b and pET28a plasmids. The recombinant construct was analysed by restriction-enzyme digestion and sequencing to confirm its sequence fidelity, and the positive recombinant plasmids were named as pET22-TNF30 and pET28-TNF30 ().
Expression and identification of recombinant proteins
The recombinant plasmids were transformed into E. coli Rosetta (DE3) for expression. A single colony containing the recombinant plasmid was cultured in medium containing antibiotics (50 μg/mL ampicillin for pET22b and 30 μg/mL kanamycin for pET28a) at 37 °C. When the cultures reached optical density (OD600) about 0.6, isopropy-beta-D-thiogalactopyranoside (IPTG) was added to the broth for induction of the recombinant protein. After the induction, the bacterial cells were lysed by sonication in phosphate buffer; and then the supernatant obtained after centrifugation (13,000 × g, 30 min) was collected as the soluble protein sample. The protein was detected by 15% sodium dodecyl sulphate-polyacrylamide gel electrophoresis (SDS-PAGE) at 80 V for 15 min and then at 120 V for 120 min. Furthermore, in order to confirm the correctness of the obtained protein, the recombinant products were detected by Western blot using mouse anti-His6 (1:5000, Santa Cruz, USA) antibodies, and then incubated with IRDye™-800 conjugated anti-mouse secondary antibodies (Li-COR Biosciences, USA). The specific proteins were visualised by Odyssey Infrared Imaging System (Gene Company, China). In addition, to find the optimal conditions for the protein expression, we tested the effects of different media (High-salt LB, Low-salt LB, LB+Glycerol, TB, SB, SOB, SOC, 2 × YC) on the yield of recombinant products. Besides, the concentration of inducer IPTG, the incubation time and the temperature were optimised with response surface analysis using the Design-Expert 8.5 software.
Purification of recombinant TNF30
The recombinant protein was separated using affinity chromatography with Ni-NAT agarose resin (GE Healthcare life science). For cleavage of the His6 tag, the purified fusion protein was incubated with Factor Xa cleavage buffer (50 mmol/L Tris-HCl, 150 mmol/L NaCl, and 1 mmol/L CaCl2, pH 7.5) plus 80 U of Factor Xa protease for 16 h at 23 °C. Subsequently, to further purify the excised TNF30, the cleaved protein was incubated with the Ni2+-chelating resin for 0.5 h to allow the His6 tag to bind with the resin. The TNF30 was then collected as supernatant after centrifugation (1,000 × g, 1 min).
Affinity analysis
In order to detect the affinity of the recombinant TNF30 with TNFα, Western blot analysis was performed. Briefly, 4 μg TNFα was loaded on SDS-PAGE. Then samples were transferred to nitrocellulose membranes and blocked for 60 min at room temperature in 5% skim milk (w/v). The membranes were incubated with 10 μg/mL His6-fused TNF30 or products of strains harbouring the pET28a empty vector for 60 min at 37 °C, immunoblotted with mouse anti-His6 (1:5000, Santa Cruz, USA) antibodies overnight at 4 °C, and then incubated with IRDye™-800 conjugated anti-mouse antibodies (Li-COR Biosciences, USA) for 2 h at room temperature. The specific proteins were visualised by Odyssey Infrared Imaging System (Gene Company, China).
Animals and carrageenan-induced paw oedema
In order to detect the inhibitory effects on inflammation of the recombinant TNF30 in vivo, paw edema was induced by subcutaneous injection of 25 μL of 1% (suspension in saline) lambda carrageenan into the right hind paw of male ICR mice (20–25 g). TNF30 (30 or 60 mg/kg), aspirin (200 mg/kg) or phosphate buffered saline (PBS) were intraperitoneally administered 30 min before the carrageenan injection. The paw diameter (D) of mice was measured by calipers, before and after injection of carrageenan at different time intervals (1, 2, 3, 4, 5 and 6 h). The oedema rate was calculated as follows:
After 6 h of carrageenan treatment, the mice were sacrificed by neck dislocation, and the tissue of the paws was removed and fixed in alfac solution. Each sample was embedded in paraffin wax, sectioned at 4 μm and stained with hematoxylin and eosin. A representative area was selected for qualitative light microscopy analysis of the inflammatory cellular response with a 20 × objective. Three slices from each animal were analysed and a minimum of three animals for each treatment were taken. All the procedures performed in the animal experiments were in accordance with the ethical standards and were approved by the College Academic Committee.
Statistical analysis
Data are presented as mean values with standard deviation (±SD). The statistically significant differences were calculated by Student's t-test. Statistical analysis was performed using GraphPad Prism version 5.00 (GraphPad, San Diego, CA, USA).
Results and discussion
Over the last decade, the therapeutic market for monoclonal antibodies (mcAb) has grown exponentially. In 2016, 5 of the top 10 best-selling drugs in the world were mcAbs [Citation12]. Currently, there are five Food and Drug Administration–approved anti-TNFα antibody drugs, adalimumab (Humira), infliximab (Remicade), etanercept (Enbrel), certolizumab (Cimzia) and golimumab (Simponi), representing more than $20 billion in sales [Citation13]. Ozoralizumab is a novel antibody drug consisting of two anti-TNFα nanobodies and one albumin-binding VHH [Citation11]. Previous studies had confirmed that ozoralizumab was superior to infliximab and can decrease clinical scores and reverse the pathology in the transgenic mouse polyarthritis model, and met both the primary and secondary endpoints without any reports of severe adverse events (SAE) in the Phase I/II clinical study [Citation14]. TNF30 is the key anti-TNFα nanobody domain of ozoralizumab and contains 115 amino acids. However, to the best of our knowledge, studies that focus on the genetic engineering of TNF30 have not been published so far. In the present study, TNF30 was first recombinantly expressed in E. coli.
Construction of the recombinant plasmids
To construct the recombinant plasmids, the coding sequence of His6-fused TNF30 was amplified by overlapping PCR, and then inserted into a pET22b or a pET28a vector (Supplemental Figure S1). Previous studies show that the position of the histidine tag could sometimes affect the expression of and the immune response to recombinant antibodies or antigens [Citation15,Citation16]. Here, the tag was introduced into the N terminus of TNF30, and the fused protein could be efficiently expressed, purified and detected by anti-hexahistidine antibodies. Furthermore, since hexahistidine has strong immunogenicity, which might cause side effects in the in vivo pharmacological studies, we used Xa protease to cleave the tag. Xa protease is an important serine endopeptidase of the coagulation cascade and it cleaves after the arginine residue in its preferred cleavage site Ile-Glu/Asp-Gly-Arg (IEGR or IDGR) [Citation17]. The results from the restriction digestion showed that the target fragments had been successfully ligated with the vector, and the DNA sequencing results also validated that the His6 tag and TNF30 were connected by a Factor Xa protease recognition site, and combined into one open reading frame, suggesting that the recombinant plasmids were constructed successfully.
Inductive expression and identification of the recombinant protein in E. coli
The recombinant plasmids pET22-TNF30 and pET28-TNF30 were transformed into E. coli Rosetta (DE3) for overexpression. After the primary screening, two high-expression positive clones harbouring pET22-TNF30 and pET28-tnf were obtained and named R213 and R803, respectively. As shown in A, after being inducted with 1 mmol/L IPTG, both R213 and R803 exhibited significant expression of His6-fused TNF30, and the Western blot results showed that both these recombinant products could be detected by the anti-His6 antibodies (B), suggesting that His6-fused TNF30 was expressed in E. coli Rosetta (DE3) successfully. In addition, since the yield of R803 seemed higher than that of R213 (B), R803 was chosen as the expression strain in the following studies. Furthermore, the results showed that the TNF30 could be expressed in a soluble form in both the cytoplasm and the periplasm. Since the cytoplasmic space is larger than the periplasmic space, the yield in the cytoplasm is higher than that in the periplasm, suggesting that disulphide bonds might not be essential for TNF30. Similiarly, many other previous studies have also reported that activated nanobodies could be expressed well in the cytoplasm [Citation18–20].
In addition, to find the optimal culture medium for the expression, the effects of eight media on the yield of recombinant products were tested. As shown in , the expression of TNF30 in different media was: SB > TB > 2 × YT > Low-salt LB > LB+Glycerol > SOB > SOC; therefore, the SB medium was chosen as the optimal medium for the recombinant expression of TNF30. Furthermore, the analysis also found that the optimal fermentation conditions were induction with 0.1 mmol/L IPTG at 22 °C for 14 h. Under these conditions, the yield of recombinant TNF30 was 20.2 mg/L (Supplemental Figure S2). Here, our data showed that the low temperature, low concentration of inducer and long incubation time could be more helpful in the fermentation of the recombinant TNF30. This is very similar to the study of Sina et al. [Citation21]. They found that low concentrations of lactose and incubation at 10 °C could promote the soluble expression of anti-TNF-α scFv. The reason may be that low inductions and low temperatures reduce the synthesis rate of the protein, allowing enough time for folding. Under the optimised conditions, the production of the recombinant TNF30 antibodies reached 20.2 mg/L, and this yield was consistent with many studies focused on the recombinant expression of antibodies in E. coli [Citation18,19,21,22].
Purification of the recombinant protein
After the IPTG induction, the bacteria were supersonically disintegrated and the recombinant His6-fused TNF30 was purified by Ni2+ affinity chromatography. As shown in , when the imidazole concentration increased to 130 mmol/L, the target protein was well eluted, whereas there is no corresponding band in the eluted products of the strains harbouring the pET28a empty vector.
Figure 3. Purification of the recombinant His6-tagged TNF30. Lane M: protein maker (Protein Pre-stained Marker, Thermos); Lane1: supernatant of the recombinant cell lysate; Lane 2: flow-through; Lanes 3–8: gradient elution with imidazole at concentration of 20, 40, 70, 130, 250 and 500 mmol/L, respectively; Lane9: cell lysate supernatant of E. coli Rosetta (DE3) harbouring a pET28a empty plasmid.
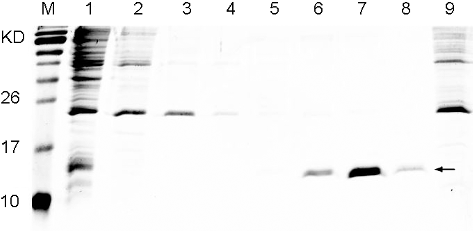
Binding affinity of recombinant TNF30 with TNFα
To confirm whether the recombinant TNF30 had proper affinity to its antigen, TNFα was electrophoresed and then transferred onto nitrocellulose membranes. Western blot was performed using the recombinant TNF30 as the first antibody, and then immunoblotting with mouse anti-His6 antibodies, followed by dye-labeled anti-mouse antibodies. As shown in , compared with the negative control, a significant band was seen when TNFα was immunobotted with the recombinant TNF30, suggesting that the obtained nanobodies have strong TNFα-binding capability.
Anti-inflammation activity of TNF30 in vivo
Finally, in order to determine whether the recombinant TNF30 had desired anti-inflammation activity, a carrageenan-induced paw oedema experiment was conducted. The His6-fused TNF30 was incubated with Factor Xa protease to cleave the His6 tag, and the excised TNF30 was purified by Ni2+-chelating resin again. The purified TNF30 was then intraperitoneally administered to mice 30 min before the carrageenan injection. As seen in , after the carrageenan injection, the paw volume increased till 4 h and kept swelling within 6 h. Compared to the vehicle treated control, both the recombinant TNF30 and the positive control drug, aspirin, showed a remarkable inhibitory effect in carrageenan-induced paw oedema (P<0.05).
Table 1. Anti-inflammation activity of TNF30 in the carrageenan-induced mice paw oedema model (X±SD; n = 8).
Further, histopathological analysis was performed after 6 h of carrageenan injection. shows representative photos of the paw tissues with different treatments. Compared with the non-treated mice, the mice injected with carrageenan but without drug therapy showed significant oedema of the real cortex, hyperplasia of the blood vessels, and more lymphocyte and neutrophils infiltration. However, when the mice were treated by the recombinant TNF30 before the carrageenan injection, the degree of oedema and hyperplasia were markedly decreased, and the infiltrating lymphocytes and neutrophils were also reduced, these effects exhibiting a dose-dependent manner. The results suggested that the recombinant TNF30 had potential anti-inflammatory activity in vivo.
Conclusions
This study presented an efficient recombinant expression system of anti-TNFα nanobody TNF30, laying a foundation for the industrial development of the drug. In addition, subsequent mutation and evolution studies on the obtained TNF30 gene using error-prone PCR, DNA shuffling and other technologies could also produce some more active derivatives against TNFα.
Supplemental Material
Download PDF (538.9 KB)Acknowledgments
This study was financially supported by Tianjin Science and Technology Support Program under grant number 14CZDSY0005.
Disclosure statement
No potential conflict of interest was reported by the authors.
Additional information
Funding
References
- Aggarwal BB. Signalling pathways of the TNF superfamily: a double-edged sword. Nat Rev Immunol. 2003;3:745–756.
- Ihnatko R, Kubes M. TNF signaling: early events and phosphorylation. Gen Physiol Biophys. 2007;26:159–167.
- Walsh G. Biopharmaceutical benchmarks 2014. Nat Biotechnol. 2014;32:992–1000.
- Muyldermans S. Nanobodies: natural single-domain antibodies. Annu Rev Biochem. 2013;82:775–797.
- Kijanka M, Dorresteijn B, Oliveira S, et al. Nanobody-based cancer therapy of solid tumors. Nanomedicine. 2015;10:161–174.
- Zhao A, Tohidkia MR, Siegel DL, et al. Phage antibody display libraries: a powerful antibody discovery platform for immunotherapy. Crit Rev Biotechnol. 2016;36:276–289.
- Zamani M, Berenjian A, Hemmati S, et al. Cloning, expression, and purification of a synthetic human growth hormone in Escherichia coli using response surface methodology. Mol Biotechnol. 2015;57:241–250.
- Desmyter A, Spinelli S, Roussel A, et al. Camelid nanobodies: killing two birds with one stone. Curr Opin Struct Biol. 2015;32C:1–8.
- Lauwereys M, Arbabi Ghahroudi M, Desmyter A, et al. Potent enzyme inhibitors derived from dromedary heavy-chain antibodies. EMBO J. 1998;17:3512–3520.
- Willrich MA, Murray DL, Snyder MR. Tumor necrosis factor inhibitors: clinical utility in autoimmune diseases. Transl Res. 2015;165:270–282.
- Krah S, Schroter C, Zielonka S, et al. Single-domain antibodies for biomedical applications. Immunopharmacol Immunotoxicol. 2016;38:21–28.
- Strohl WR. Current progress in innovative engineered antibodies. Protein Cell. 2018;9:86–120.
- Martin BC, Thomas LW, Dann FJ. Apremilast for the treatment of psoriatic arthritis. Dermatol Online J. 2017;23:1–12.
- Steeland S, Vandenbroucke RE, Libert C. Nanobodies as therapeutics: big opportunities for small antibodies. Drug Discov Today. 2016;21:1076–1113.
- del Mar Garcia-Suarez M, Villaverde R, Gonzalez-Rodriguez I, et al. Oriented immobilization of anti-pneumolysin tagged recombinant antibody fragments. Curr Microbiol. 2009;59:81–87.
- Krupka M, Masek J, Barkocziova L, et al. The position of His-Tag in recombinant OspC and application of various adjuvants affects the intensity and quality of specific antibody response after immunization of experimental mice. PLoS One. 2016 [cited 2017 Nov 28];11:e0148497. 10.1371/journal.pone.0148497.
- Jenny RJ, Mann KG, Lundblad RL. A critical review of the methods for cleavage of fusion proteins with thrombin and factor Xa. Protein Expr Purif. 2003;31:1–11.
- Djender S, Schneider A, Beugnet A, et al. Bacterial cytoplasm as an effective cell compartment for producing functional VHH-based affinity reagents and Camelidae IgG-like recombinant antibodies. Microb Cell Fact. 2014[cited 2017 Nov 28];13:140. 10.1186/s12934-014-0140-1.
- de Marco A. Co-expression and co-purification of antigen-antibody complexes in bacterial cytoplasm and periplasm. Methods Mol Biol. 2014;1129:125–135.
- Billen B, Vincke C, Hansen R, et al. Cytoplasmic versus periplasmic expression of site-specifically and bioorthogonally functionalized nanobodies using expressed protein ligation. Protein Expr Purif. 2017;133:25–34.
- Sina M, Farajzadeh D, Dastmalchi S. Effects of environmental factors on soluble expression of a humanized anti-TNF-alpha scFv antibody in Escherichia coli. Adv Pharm Bull. 2015;5:455–461.
- Kipriyanov SM, Moldenhauer G, Little M. High level production of soluble single chain antibodies in small-scale Escherichia coli cultures. J Immunol Methods. 1997;200:69–77.