Abstract
The present study aimed to investigate the protective effect and mechanism of action of astaxanthin (AST) on the oxidative stress injury of adult retinal pigment epithelium-19 (ARPE-19) cells induced by hydrogen peroxide (H2O2). ARPE-19 cells were divided into five groups, including control group, H2O2 model group, AST1 group, AST2 group and AST3 group. MTT was used to determine cell viability. Cell morphology was visualized under an inverted fluorescence microscope. TUNEL assay and flow cytometry was performed to detect cell apoptosis. To examine the levels of ROS, SOD and MDA, we used DCFH-DA, WST-1 and TBA assays. The optimal concentration of AST for increasing cell viability was 40 µg/L. Pretreatment with AST alleviated damages in cell morphology induced by H2O2. Pretreatment with AST had the best protective effect on ARPE-19 cells from oxidative stress injury induced by H2O2. AST treatment had protective effect on ARPE-19 cells against apoptosis. Treatment with AST reduced the apoptotic rate of ARPE-19 cells induced by H2O2. Treatment with AST altered the levels of ROS, SOD and MDA in ARPE-19 cells induced by H2O2. The present study demonstrates that AST protects ARPE-19 cells against oxidative stress injury, probably by inhibiting the production of ROS, elevating the activity of SOD and reducing the content of MDA. Of note, preventive delivery of AST (40 µg/L) has the best effect. The present study also provides a theoretical basis for the prevention and treatment of age-related macular degeneration.
Introduction
Age-related macular degeneration (AMD) is an age-related disease that causes progressive degeneration of retinal macular photoreceptor and retinal pigment epithelium (RPE) [Citation1]. Studies show that oxidative stress may be an important pathogenesis of AMD that involves the targeting of RPE cells, and reduced number and dysfunction of macular RPE cells are considered to be essential for the occurrence of AMD [Citation2,Citation3]. Therefore, improving the antioxidant capacity of RPE cells by treatment or nutritional intervention may provide a way for the therapeutics of AMD.
Under a variety of noxious stimuli, living organisms produce a greater amount of reactive oxygen species (ROS), which overwhelms the oxide-scavenging ability of the body. In this case, dynamic balance is interrupted, and oxidative stress is generated [Citation4]. Superoxide dismutase (SOD) is an important antioxidant enzyme that defenses against superoxide anion (O2–) damage. SOD scavenges O2– and prevents its direct or indirect damages on the body [Citation5]. Malondialdehyde (MDA) is a major metabolite of lipid peroxidation, the content of which indirectly reflects the production levels of free radicals and the oxidative damage levels in body tissues [Citation6]. Apoptosis plays an important role in many pathophysiological processes, such as growth, development and aging and oxidative stress is an important factor that causes apoptosis [Citation7].
Astaxanthin (AST) is considered one of the most powerful antioxidants with multiple biological functions, such as antioxidant effect, anti-inflammation, anti-hypertension, immunity enhancement and anti-tumor effect [Citation8,Citation9]. AST is a chain-breaking antioxidant with special molecular structure and super antioxidant activity [Citation10]. In the present study, we investigate the protective effect of AST on ARPE-19 cells with induced oxidative stress damages, as well as its mechanism of action.
Materials and methods
Cells
ARPE-19 cell line was purchased from Jennio Biotech Co., Ltd (Guangzhou, China) and cultured in RPMI-1640 medium supplemented with 10% fetal bovine serum (Thermo Fisher Scientific, Waltham, MA, USA). ARPE-19 cell model of oxidative stress damage was constructed by incubating the cells with 200 µmol/L hydrogen peroxide (H2O2). The cells were divided into five groups, including control group, H2O2 model group, AST1 group, AST2 group and AST3 group. For the control group, the cells were cultured as described above, without addition of any drug. For the H2O2 model group, the cells were cultured with normal medium containing 200 µmol/L H2O2 for 24 h. For the AST1 group, the cells were incubated with normal medium containing optimal concentration (determined using MTT assay as described later) of AST (Sigma-Aldrich, St. Louis, MO, USA) for 1 h, and then cultured for another 24 h after addition of 200 µmol/L H2O2. For the AST2 group, the cells were incubated with normal medium containing both optimal concentration of AST and 200 µmol/L H2O2 for 24 h. For the AST3 group, the cells were first incubated with normal medium containing 200 µmol/L H2O2 for 1 h, and then cultured for another 24 h after addition of optimal concentration of AST.
MTT assay
ARPE-19 cells were seeded into 96-well plates and cultured under normal conditions. To identify the optimal concentration of AST, ARPE-19 cell viability was tested using MTT assay after incubation with 10 µg/L, 20 µg/L and 40 µg/L AST for 24 h. Optical density (472 nm) was measured using a reader (IFM200 Pro, Tecan, Zurich, Switzerland) to reflect cell viability. The concentration of AST at which the cells had the highest viability was used as the optimal concentration for further examinations. ARPE-19 cells in control group, H2O2 model group, AST1, AST2 and AST3 were treated accordingly and optical density (472 nm) was obtained after cultivation for 24 h.
Microscopy
After treatments, ARPE-19 cells in each group were visualized under an inverted fluorescence microscope (OLYMPUS BX53, Olympus, Tokyo, Japan). The morphological changes of the cells between groups were evaluated.
Terminal deoxynucleotidyl transferase dUTP nick end labelling (TUNEL) assay
ARPE-19 cells in each group were seeded into 48-well plates, and subjected to fixation, blocking and infiltration. Then, 50 μL TUNEL reaction mixture was added into each well, and the cells were incubated in wet box for 1 h. Then, the nuclei of the cells were stained with 4’,6-diamidino-2-phenylindole (DAPI) for 15 min. Finally, the cells were observed under an inverted fluorescence microscope (BX53; Olympus).
Flow cytometry
ARPE-19 cells were harvested and centrifuged for 10 min before discarding supernatants. The cell pellets were resuspended in 200 μL binding buffer before addition of 10 μL Annexin V-FITC and 10 μL propidium iodide (Solarbio, Beijing, China). Then, the cells were incubated at room temperature in dark for 15 min, followed by addition of 300 μL binding buffer. Within 1 h, the cells were examined using flow cytometry (FACSCantoII, BD Biosciences, San Jose, CA, USA).
Dichloro-dihydro-fluorescein diacetate (DCFH-DA) method
The expression of ROS was tested using DCFH-DA kit (Wanleibio, Shanghai, China). First, 70 µmol/L DCFH-DA was added into the culture medium and incubated at 37 °C for 60 min. After centrifugation for 5 min, the supernatants were discarded, followed by washing with phosphate-buffered saline twice. The cell deposits were collected and examined for fluorescence intensity using a fluorospectrophotometer (F-7000; Hitachi, Tokyo, Japan) at 525 nm.
WST-1 assay
To measure the activity of SOD in ARPE-19 cells, WST-1 assay was used. Cells were seeded in 96-well plates, and divided into control group, blank control group, measurement group and blank measurement group. WST-1 assay was performed according to the manufacturer’s protocol (Beyotime, Shanghai, China). Optical density was measured using a reader (IFM200 Pro, Tecan, Zurich, Switzerland) and the activity of SOD was calculated.
Thiobarbituric acid (TBA) method
To determine the level of MDA, the TBA method was employed. The cells were divided into standard, blank, measurement and control groups. The assay was performed according to the manufacturer’s manual (Nanjing Jiancheng Bioengineering Institute). Optical density was measured using a reader (IFM200 Pro, Tecan, Zurich, Switzerland) and the level of MDA was calculated.
Statistical analysis
The results were analyzed using SPSS 19.0 statistical software (IBM, Armonk, NY, USA). All data were expressed as means ± standard deviations (n = 3). Comparison among multiple groups was performed using single factor analysis of variance, and comparison between two groups was performed with independent samples t-test. Differences with p < 0.05 were considered statistically significant.
Results and discussion
The optimal concentration of AST for increasing cell viability is 40 µg/L
To determine the optimal concentration of AST, ARPE-19 cell viability was tested using MTT assay after incubation with 10, 20 and 40µg/L AST for 24 h. The data showed that the viability of cells treated with H2O2 was significantly reduced compared with control (p < 0.05). By contrast, treatment of cells in the H2O2 model group with AST significantly enhanced the cell viability in a dose-dependent manner (p < 0.05), with the effect of 40 µg/L AST being the strongest (). Preliminary experiments showed that concentrations of AST >40 μg/L did not provide better protection (data not shown). The result suggests that the optimal concentration of AST for increasing cell viability is 40 µg/L.
Figure 1. Effect of different AST concentrations on the viability of ARPE-19 cells treated with 200 µmol/L H2O2. Note. MTT assay was performed after treatment with H2O2 for 24 h, following pre-incubation with 10, 20 and 40 µg/L AST for 1 h. Relative cell viability was calculated from the optical density value at 472 nm against that of the control group. #, p < 0.05 compared with control; *, p < 0.05 compared with H2O2 group.
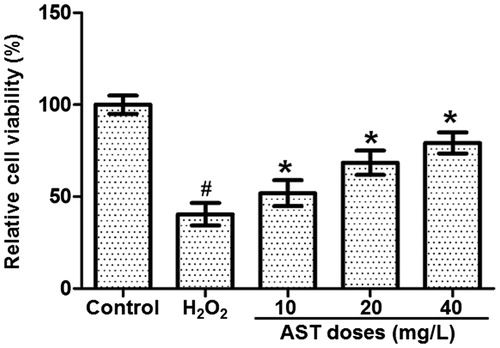
Pretreatment with AST alleviates damages in cell morphology induced by H2O2
To test the effect of AST on the morphology of ARPE-19 cells, the cells in each group were visualized under an inverted fluorescence microscope. ARPE-19 cells in the control group showed even density, stretched shapes and spindle-shaped adherent monolayer growth, but no floating cells. By contrast, the cells in the H2O2 model group were sparse in density, shrunk in shape and decreased in size, and some cells were floating in the culture medium. Treatment with AST improved the morphology of the cells, which was healthier than that in the H2O2 model group. Of note, the morphology of the cells in the AST1 group was improved the most, with the fewest floating cells (). The result indicates that pretreatment with AST alleviates the damages in cell morphology induced by H2O2.
Figure 2. Effect of AST on the morphology of ARPE-19 cells treated with H2O2. Control group, non-treated cells. H2O2 group, cells treated with 200 µmol/L H2O2 for 24 h. AST1 group, cells treated with 40 µg/L AST for 1 h, and then cultured for another 24 h after addition of 200 µmol/L H2O2. AST2 group, cells treated with 40 µg/L AST and 200 µmol/L H2O2 for 24 h. AST3 group, cells first treated with 200 µmol/L H2O2 for 1 h, and then cultured for another 24 h after addition of 40 µg/L AST. Note: Morphological changes under an inverted fluorescence microscope (×200).
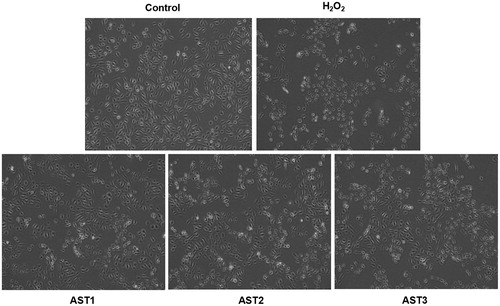
Treatment with AST has the best protective effect on ARPE-19 cells from oxidative stress injury induced by H2O2
To study the effect of AST treatment on the viability of cells pretreated with H2O2, MTT assay was carried out. The data showed that the relative cell viability in the H2O2 model group was significantly lower than that in the control group (p < 0.05). However, treatment with AST significantly enhanced the relative cell viability compared with the H2O2 model group (p < 0.05). Of note, the relative cell viability in the AST2 and the AST3 groups was both significantly lower than that in the AST1 group (p < 0.05), and that in the AST3 group was significantly lower than that in the AST2 group (p < 0.05) (). These results suggest that pretreatment with AST has the best protective effect on ARPE-19 cells from oxidative stress injury induced by H2O2.
Figure 3. Effect of AST treatment on the viability of ARPE-19 cells treated with H2O2. Note: MTT assay. Optical density was measured at 472 nm, and the relative cell viability of each group was calculated from the optical density value against that of the control group. #, p < 0.05 compared with control; *, p < 0.05 compared with H2O2 model group; $, p < 0.05 compared with AST1 group; &, p < 0.05 compared with AST2 group.
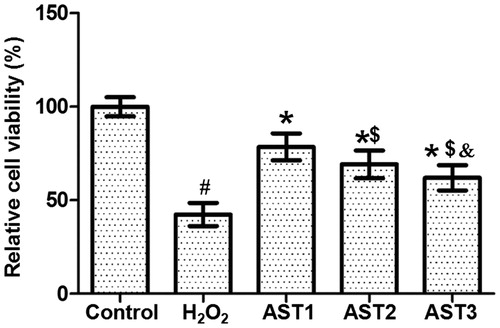
AST treatment has protective effect on ARPE-19 cells against apoptosis
To investigate how AST affects the apoptosis of ARPE-19 cells, TUNEL assay was performed. The images showed that cells in the H2O2 model group had aggravated chromatin condensation, obvious karyopyknosis and increased number of apoptotic bodies. However, AST treatment alleviated the condition of apoptosis. The number of apoptotic cells in the AST1 group was the smallest, and the protective effect in the AST2 group was better than that in the AST3 group (). The result indicates that AST treatment has protective effect on ARPE-19 cells against apoptosis.
Figure 4. Effect of AST on the apoptotic staining of ARPE-19 cells induced by H2O2. Note: TUNEL assay was used to investigate the apoptosis of the cells. Apoptotic cells are shown in green colour under the inverted fluorescence microscope (×200). Then, the images of cells stained by TUNEL assay and DAPI were merged, and the intensity was quantified and expressed as histograms. #, p < 0.05 compared with control; *, p < 0.05 compared with H2O2 model group; $, p < 0.05 compared with AST1 group; &, p < 0.05 compared with AST2 group.
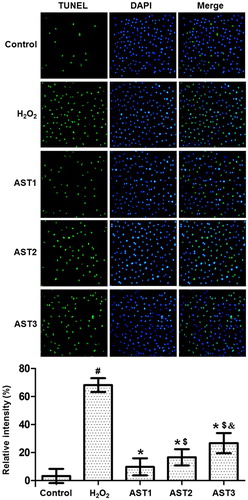
Treatment with AST reduces the apoptotic rate of ARPE-19 cells induced by H2O2
To determine the apoptotic rate of cells in each group, flow cytometry was employed. The apoptotic rate in the H2O2 model group was significantly higher than that in the control group (p < 0.05). Treatment with AST reduced the elevated apoptotic rate induced by H2O2 (p < 0.05). Of note, the apoptotic rate in the AST1 group was the lowest, and that in the AST3 group was significantly higher than that in AST2 (p < 0.05) (). The result suggested that treatment with AST reduced the apoptotic rate of ARPE-19 cells induced by H2O2.
Figure 5. Effect of AST on the apoptotic rate of ARPE-19 cells induced by H2O2. (a) Flow cytometry analysis of ARPE-19 cells in each group. (b) Quantification of apoptotic rate of each group. Note: Data are expressed as mean values with standard deviations (±SD). #, p < 0.05 compared with control; *, p < 0.05 compared with H2O2 model group; $, p < 0.05 compared with AST1 group; &, p < 0.05 compared with AST2 group.
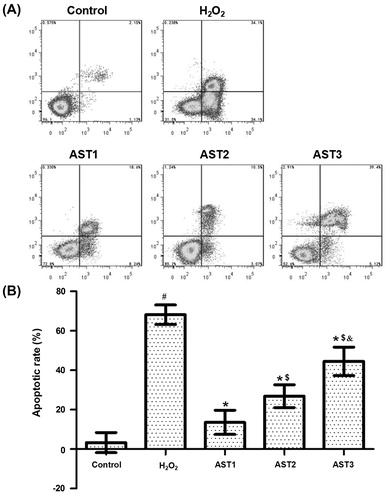
Treatment with AST alters the levels of ROS, SOD and MDA in ARPE-19 cells induced by H2O2
To examine the levels of ROS, SOD and MDA, DCFH-DA, WST-1 and TBA assays were used. The data showed that intracellular ROS level inH2O2 model group was significantly higher than that in the control group, and treatment with AST significantly reduced the ROS level induced by H2O2, with that in the AST1 group being the lowest (p < 0.05) ( and ). In addition, the level of SOD in the H2O2 model group was significantly lower than that in the control group, and treatment with AST significantly enhanced the SOD level, with that in the AST1 group being the highest (p < 0.05) (). Moreover, the content of MDA in the H2O2 model group was significantly higher than that in the control group, while treatment with AST significantly decreased the content of MDA, with that in AST1 being the lowest (p < 0.05) (). These results indicate that treatment with AST altered the levels of ROS, SOD and MDA in ARPE-19 cells induced by H2O2.
Comparative analysis
AMD is a common macular degeneration of the fundus that causes irreversible decline or loss of vision in patients [Citation11,Citation12]. Due to high metabolic rate, high oxygen consumption and long-term exposure to light, RPE cells are easily damaged by oxidative stress. Studies show that RPE damage induced by oxidative stress is a key factor for AMD pathogenesis [Citation13,Citation14]. The antioxidant studied here is one of the most powerful antioxidants known in nature. It directly scavenges oxygen free radicals and has strong antioxidant activities. The effect of AST is stronger than that of zeaxanthin, lutein, β-carotene and vitamin E, etc. [Citation15]. Especially, the antioxidant activity of AST is stronger than NAC [Citation16]. Hosaka et al. [Citation17] report that AST can quickly remove hydroxyl radicals, and the antioxidant activity of AST is stronger than that of ascorbic acid or vitamin E.
In the present study, we determined that the optimal concentration of AST that has the best protective effect on ARPE-19 cells is 40 µg/L, being consistent with a previous study [Citation18]. Here, we only studied its effect on this specific type of cells. However, the antioxidant effect of AST on other cell types still needs further studies in the future. A previous study demonstrates that 20 µg/L AST has better protective effect [Citation19], but preliminary experiments of the present study showed that 40 µg/L AST had the best protective effect against ARPE-19 cell injury induced by H2O2. MTT assay also showed that AST protected ARPE-19 cells against oxidative stress injury induced by H2O2, and preventive delivery of AST could have the best effect. TUNEL assay demonstrated that AST treatment alleviated the apoptosis of ARPE-19 cells induced by H2O2, and flow cytometry showed that AST decreased the apoptotic rate of the cells. Of note, preventive delivery of AST had the best effect compared to the other two delivery methods. Moreover, AST reduced the production of ROS, decreased the content of MDA and enhanced the activity of SOD [Citation20–22], suggesting that AST inhibited oxidative stress induced by H2O2, being consistent with a previous report [Citation23]. The present study found that 40 µg/L of AST provided the best protective effect on ARPE-19 cells. In the end, AST may be used as potential agent for the prevention and treatment of AMD [Citation19,Citation24,Citation25]. Oxidative stress may be an important factor on expression of AMD in ARPE-19 cells [Citation26–28].
Conclusions
In summary, AST protects ARPE-19 cells against oxidative stress injury induced by H2O2, probably by inhibiting the production of ROS, elevating the activity of SOD and reducing the content of MDA. Of note, preventive delivery of AST (40 µg/L) has the best effect. The present study also provides a novel idea for the prevention and treatment of AMD. However, it is still unclear whether an inducer of SOD can cooperate with AST treatment, and this may be the subject of future study.
Disclosure statement
No potential conflict of interest was reported by the authors.
Additional information
Funding
References
- Liang FQ, Godley BF. Oxidative stress-induced mitochondrial DNA damage in human retinal pigment epithelial cells: a possible mechanism for RPE aging and age-related macular degeneration. Exp Eye Res. 2003;76(4):397–403.
- Kawada H, Blessing K, Kiyota T, et al. Effects of multifunctional antioxidants on mitochondrial dysfunction and amyloid-beta metal dyshomeostasis. J Alzheimers Dis. 2015;44(1):297–307.
- Awh CC, Hawken S, Zanke BW. Treatment response to antioxidants and zinc based on CFH and ARMS2 genetic risk allele number in the Age-Related Eye Disease Study. Ophthalmology. 2015;122(1):162–169.
- Jeung IC, Jee D, Rho CR, et al. Melissa Officinalis L. Extracts protect human retinal pigment epithelial cells against oxidative stress-induced apoptosis. Int J Med Sci. 2016;13(2):139–146.
- Yan T, Bi H, Wang Y. Wogonin modulates hydroperoxide-induced apoptosis via PI3K/Akt pathway in retinal pigment epithelium cells. Diagn Pathol. 2014;9:154. DOI:10.1186/s13000-014-0154-3
- Maboudou P, Mathieu D, Bachelet H, et al. Detection of oxidative stress. Interest of GC-MS for malondialdehyde and formaldehyde monitoring. Biomed Chromatogr. 2002;16(3):199–202.
- Buttke TM, Sandstrom PA. Oxidative stress as a mediator of apoptosis. Immunol Today. 1994;15(1):7–10.
- Zhang XS, Zhang X, Zhou ML, et al. Amelioration of oxidative stress and protection against early brain injury by astaxanthin after experimental subarachnoid hemorrhage. J Neurosurg. 2014;121(1):42–54.
- Qiu X, Fu K, Zhao X, et al. Protective effects of astaxanthin against ischemia/reperfusion induced renal injury in mice. J Transl Med. 2015;13:28. DOI:10.1186/s12967-015-0388-1
- Xu L, Zhu J, Yin W, et al. Astaxanthin improves cognitive deficits from oxidative stress, nitric oxide synthase and inflammation through upregulation of PI3K/Akt in diabetes rat. Int J Clin Exp Pathol. 2015;8(6):6083–6094.
- Gammone MA, Riccioni G, D'Orazio N. Marine carotenoids against oxidative stress: effects on human health. Mar Drugs. 2015;13(10):6226–6246.
- Mates JM, Segura JA, Alonso FJ, et al. Oxidative stress in apoptosis and cancer: an update. Arch Toxicol. 2012;86(11):1649–1665.
- Junttila MR, Li SP, Westermarck J. Phosphatase-mediated crosstalk between MAPK signaling pathways in the regulation of cell survival. FASEB J. 2008;22(4):954–965.
- Regnier P, Bastias J, Rodriguez-Ruiz V, et al. Astaxanthin from Haematococcus pluvialis prevents oxidative stress on human endothelial cells without toxicity. Mar Drugs. 2015;13(5):2857–2874.
- Xu G, Huang Y, Luo Y. Progress of production process, pharmacological activity and application research of astaxanthin. Strait Pharm J. 2015;27:8–12.
- Tokarz P, Kaarniranta K, Blasiak J. Role of antioxidant enzymes and small molecular weight antioxidants in the pathogenesis of age-related macular degeneration (AMD). Biogerontology. 2013;14(5):461–482.
- Hosaka S, Obuki M, Nakajima J, et al. Comparative study of antioxidants as quenchers or scavengers of reactive oxygen species based on quenching of MCLA-dependent chemiluminescence. Lumin J. 2005;20(6):419–427.
- Wang JY, Lee YJ, Chou MC, et al. Astaxanthin protects steroidogenesis from hydrogen peroxide-induced oxidative stress in mouse Leydig cells. Mar Drugs. 2015;13(3):1375–1388.
- Li Z, Dong X, Liu H, et al. Astaxanthin protects ARPE-19 cells from oxidative stress via upregulation of Nrf2-regulated phase II enzymes through activation of PI3K/Akt. Mol Vis. 2013;19:1656–1666.
- Song XD, Liu WB, Zhang LX. Antioxidative action of astaxanthin in ECV-304 cells and its mechanism. J Binzhou Med Uni. 2009;6:161–166.
- Liu X, Luo Q, Cao Y, et al. Mechanism of different stereoisomeric astaxanthin in resistance to oxidative stress in caenorhabditis elegans. J Food Sci. 2016;81(9):H2280–2287.
- Fan DD, Lin S, Song YP, et al. Astragaloside IV protects rat gastric mucosa against aspirin-induced damage. Int Immunopharmacol. 2016;41:47–55.
- Feng Y, Chu A, Luo Q, et al. The protective effect of astaxanthin on cognitive function via inhibition of oxidative stress and inflammation in the brains of chronic T2DM rats. Front Pharmacol. 2018;9:748–752.
- Gopinath B, Flood VM, Kifley A, et al. Smoking, antioxidant supplementation and dietary intakes among older adults with age-related macular degeneration over 10 years. PLoS One. 2015;10(3):e0122548. DOI:10.1371/journal.pone.0122548
- Plestina-Borjan I, Katusic D, Medvidovic-Grubisic M, et al. Association of age-related macular degeneration with erythrocyte antioxidant enzymes activity and serum total antioxidant status. Oxidative Med Cell Longev. 2015;2015:1–8. DOI:10.1155/2015/804054
- Ayaz L, Dinc E. Evaluation of microRNA responses in ARPE-19 cells against the oxidative stress. Cutaneous Ocul Toxicol. 2018;37(2):121–126.
- Zhu C, Dong Y, Liu H, et al. Hesperetin protects against H2O2-triggered oxidative damage via upregulation of the Keap1-Nrf2/HO-1 signal pathway in ARPE-19 cells. Biomed Pharmacother. 2017;88:124–133.
- Wang K, Yao Y, Zhu X, et al. Amyloid beta induces NLRP3 inflammasome activation in retinal pigment epithelial cells via NADPH oxidase- and mitochondria-dependent ROS production. J Biochem Mol Toxicol. 2017;31:e21887. DOI:10.1002/jbt.21887