Abstract
Abiotic stresses, including extreme temperature, drought and salinity, seriously threaten the growth and development of tea plants. The tea plant fights against various threats to innate defensive systems by regulating the expression profiles of related anti-genes. R2R3-type MYB family transcription factors (TFs) contribute to plant defense against abiotic stresses. Five CsMYB TFs were identified from the tea plant according to the transcriptome database. Conserved motif analysis revealed that subgroups 20 and 22 were conserved in the N-terminus with two motifs. All five CsMYB TF sequence homologies had a flexible C-terminal region. Subcellular localization analysis indicated that the protein of CsMYB19 was specifically localized in the nucleus. The expression patterns of CsMYB genes under abiotic stress treatments were significantly higher than that of the control, and the members from subgroup 20 had higher response than those in subgroup 22. During hormonal treatment, CsMYB8 was selected as highly responsive to methyl jasmonate (MeJA) treatment. The expression level of CsMYB20 was highest under ABA treatment and lowest under SA treatment. Our study serves as a foundation for understanding the response mechanisms of MYB genes under abiotic stress in tea plants.
Introduction
Tea is a non-alcoholic beverage that benefits humans. Tea positively affects weight loss [Citation1–3], reduces the incidence of all cancers [Citation2, Citation4, Citation5], improves cardiovascular health [Citation6, Citation7], enhances glucose homeostasis [Citation8, Citation9] and protects against ultraviolet (UV) radiation [Citation10]. The tea plant is an important leaf economic crop, and its growth and development, yield, and quality are greatly affected by abiotic stress. Abiotic stress seriously threatens plant growth, development, and yield [Citation11, Citation12]. Extreme temperature, drought, salt, and other abiotic stress can weaken the tea plant’s growth trend and largely reduce the yield of tea plantations [Citation13–15]. Enhancing the tolerance of tea plants against abiotic stress can improve the quality and production of tea plantations.
The MYB transcription factor (TF) family is one of the largest TFs families in plants. According to the number of adjacent repeats, MYB TFs are classified into 1R, R2R3, 3R, and 4R MYB subfamilies [Citation16]. The members of the MYB family control various processes, such as epidermal cell differentiation, stomatal aperture [Citation17, Citation18], flavonoid and anthocyanin synthesis [Citation19–21], cold and drought tolerance [Citation22], and pathogen resistance [Citation23–26]. Klempnauer et al. [Citation27] first identified the ‘oncogene’ V-myb from avian myeloblastosis virus (AMV). Since then, numerous homologous genes from MYB gene super-family have been identified in different species, including plants, animals, and fungi [Citation16, Citation28, Citation29].
The R2R3-MYB TF family is the largest MYB subfamily [Citation30]. The functions of R2R3-MYB TFs in animals are restricted to the control of cell division and differentiation, whereas those in plants are diverse and with superior specific determination [Citation23]. A total of 126 R2R3-MYB members have been found in Arabidopsis thaliana [Citation16, Citation31], 88 in Oryza sativa [Citation32], 158 in Zea mays [Citation33], 192 in Populus tremula [Citation34], 244 in Glycine max [Citation35], 205 in Gossypium raimondii [Citation36], 118 in Vitis vinifera [Citation19], and 256 in Brassica rapa [Citation37]. In the model system from A. thaliana, the AtR2R3MYB family members are divided into 25 subgroups [Citation30]. Subgroups 20 and 22 are involved in the response to biotic and abiotic stresses. For instance, AtMYB2 is highly expressed during dehydration, and transcriptional activation is detected in the C-terminal acidic region [Citation38, Citation39]. AtMYB44 could regulate EIN TF and promote the perception of ethylene. In addition, the AtMYB44 protein is localized in the nucleus [Citation40]. The high expression level of AtMYB15 can improve plant tolerance to drought and salt through coordination with interrelated protein and involvement in ABA biosynthesis and signalling [Citation41, Citation42]. MYB73 is involved in JA and SA signalling pathways as mediated by NPR1 [Citation43].
From the existing transcription group data [Citation44], 190 similar clips were screened out in the tea plant [Citation45], and 27 members with incomplete R2R3 domain were identified. On the basis of evolutionary relationships and structural analysis, five R2R3-MYB family TFs were identified from the tea plant. These TFs include CsMYB15, CsMYB19, and CsMYB21 from subgroup 22 and CsMYB8 and CsMYB20 from subgroup 20. We focused on the response of the CsMYB genes to abiotic stresses in Longjing 43 cultivar. Our study serves as a foundation for future research into the MYB TFs of tea plants.
Material and methods
Preparation of plant material
Several 2-year-old vegetatively propagated cuttings of tea plant cultivar Longjing 43 were planted at the Tea Science Research Institute of Nanjing Agriculture University. The relative humidity was programmed at 70 ± 10%, and the light intensity was maintained at 300 μmol m−2 s−1 with 16 h of light during daytime and 8 h of darkness. For artificial abiotic stress treatments, the tea plants were irrigated with ddH2O (control), 200 mmol L−1 NaCl (salt treatment), and 200 g L−1 PEG (drought treatment) at room temperature (25 °C). Cold and heat treatments were conducted by placing the tea plants in two illumination boxes (4 and 38 °C). Samples with the mixture of young leaves were collected at 0, 1, 2, 4, 8, and 24 h after different treatments; immediately frozen in liquid nitrogen; and stored at −80 °C.
Hormone treatments were performed by spraying the leaves once with 1 mmol L−1 methyljasmonate (MeJA treatment), 1 mmol L−1 gibberellic acid (GA3 treatment), 1 mmol L−1 salicylic acid (SA treatment), 1 mmol L−1 3-indoleacetic acid (IAA treatment), and 0.1 mmol L−1 abscisic acid (ABA treatment) and harvesting them after 2 h. ABA was dissolved in distilled water, and the rest of the hormones were dissolved in distilled water with 2% absolute ethanol.
RNA isolation, cDNA synthesis, and gene cloning
Total RNA was extracted using the Quick RNA extract kit. RNA reverse transcription was obtained by placing 1 µg of RNA as the template for the reverse transcription system into a 20 µL reaction volume. Reverse transcription was performed using the PrimeScript RT reagent kit with gDNA Eraser (TaKaRa, Dalian, China), and the system was diluted 16-fold for quantitative real-time polymerase chain reaction (qRT-PCR).
The five deduced sequences from CsR2R3MYB were retrieved from Camellia sinensis transcriptome [Citation44]. The CsMYB genes were amplified from Longjing 43 cultivars using cDNA as the template and the primer sequences listed in . The PCR conditions were as follows: 95 °C for 5 min; followed by 95 °C for 30 s, 55 °C for 15 s, and 72 °C for 60 s for 35 cycles; and finally 72 °C for 10 min. The sequences were amplified using S1000 Thermal Cycler PCR (Bio-Rad, Hercules, CA). The PCR products were recovered, inserted into a plasmid pMD-19T vector, and applied with the ligation mixture to transform into Escherichia coli strain (DH5α). The clones were identified using ampicillin resistance and were sequenced by Tongyong Biotechnology Inc. (Quzhou, China).
Table 1. Primers used in the text.
Quantitative real-time PCR of the CsMYB genes under abiotic stresses and hormone treatments
qRT-PCR was performed on CFX96 real-time PCR system (Hercules, CA) by using SYBR Premix Ex-Taq (TaKaRa, Dalian, China). The conditions of qRT-PCR were as follows: 95 °C for 3 min, followed by 40 cycles of 95 °C for 10 s, and 60 °C for 20 s. All reactions were performed in triplicate. GAPDH was utilized to regulate the expression level [Citation46], and the 2−ΔΔCt method was used to calculate the relative gene expression levels [Citation47].
Multiple sequence alignment, phylogenetic analysis, and conserved motif analysis
The nucleotide and amino acid sequences were examined with BLAST on the National Center for Biotechnology Information (NCBI) website. DNAMAN version 6.0 was utilized to analyze the full alignment. Information on AtR2R3MYB, OsR2R3MYB, and ZmR2R3MYB family genes was downloaded from the website of Plant TFDB [Citation48]. MEGA5 was used to create the molecular phylogenetic tree with the neighbour-joining method [Citation49]. The secondary structure prediction of the proteins was analyzed using SOPMA. The MEME website was utilized to search for conserved motifs [Citation50].
Subcellular localization analysis of CsMYB19 in tea plants
We used PSORT II Prediction to predict the subcellular localization of the five CsMYB proteins. The subcellular localization of the five CsMYB proteins was predicted to be in the nucleus. CsMYB19 was subsequently selected to further analyze the subcellular localization by using Li’s method with modifications [Citation51]. CsMYB19 was amplified with stop codons, excised, and inserted into a pA7 vector, which was used as a control and contains 35S::GFP fusion protein. The constructed plasmids were then transferred into onion epidermal cells placed on 1/2 MS (Murashige and Skoog) medium by using a helium-driven particle accelerator (PDS-1000, Bio-Rad). Production of cultures was conducted for 18–24 h and was observed using the LSM780 confocal microscopy imaging system (Zeiss, Oberkochen, Germany).
Results and discussion
In terms of functionality, the members of R2R3-type MYB play an important role in the response to environmental stress, primary and secondary metabolism, cell fate and identity, and developmental processes [Citation16, Citation24, Citation25, Citation52–54]. The first plant R2R3 MYB-encoding gene COLORED1(C1) was cloned in maize and is involved in anthocyanin metabolism [Citation55]. In the model plant Arabidopsis, the AtR2R3MYB gene family was divided into 25 subgroups [Citation30]. Subgroups 1, 2, 11, 20, and 22 are vital in the response to biotic and abiotic stresses [Citation17, Citation22, Citation36, Citation56–58]; subgroups 3, 4, 5, 6, 7, and 12 are mainly involved in primary and secondary metabolism [Citation20, Citation59, Citation60]; and subgroups 9, 14, 15, 18, 19, and 21 affect plant growth and development [Citation61–64]. The function of the rest of the subgroups remains unclear. According to alignments with the sequence in the existing C. sinensis transcriptome database [Citation44], a number of abiotic stress-related genes were detected. In addition, CsMYB genes were identified for further study.
Gene cloning from Longjing 43 cultivar
The five genes, CsMYB8, CsMYB15, CsMYB19, CsMYB20, and CsMYB21, were cloned from Longjing 43 cultivar. The open reading frame (ORF) of cloned sequences contained 933, 843, 921, 864, and 1005 bp. The integral nucleotide sequences and deduced amino acid sequences are shown in Supplementary Figures S1–S5 in the Supplemental data.
Analysis of CsMYB TF structure and conserved motif
Imperfect helix-turn-helix (HTH) structure is typically found in the MYB TF family. The R2R3-MYB TF family has two HTH structures in the N-terminus. The third helix of the HTH structure working as a recognition motif directly intercalates in the major groove of DNA [Citation31, Citation65]. The 3D structure model of CsMYB19 protein was constructed to intuitively display the HTH structure (). Two incomplete HTH structures were presented on the model of CsMYB19 protein.
Figure 1. Multiple-sequence alignment of five CsMYB transcription factors from C. sinensis with A. thaliana and O. sativa. The 3D structure model of the CsMYB19 protein (A) and conserved domains of the five predicted CsMYB proteins from subgroups 20 and 22 (B).
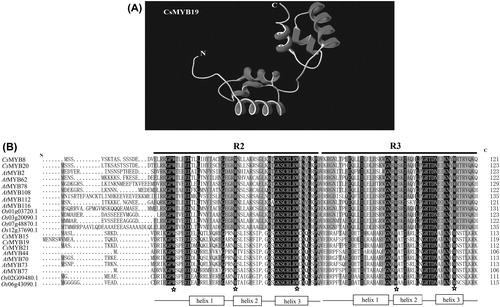
Model plants, A. thaliana and O. sativa, were selected to create multiple sequence alignment with the CsMYB TFs. shows that the domains of R2 and R3 were conserved in those species, and the sequences from the same subgroup were highly conserved. The complete multiple sequence alignments of those proteins are shown in Supplementary Figure S6. The secondary structure was predicted and analyzed on the SOPMA website to further analsze the CsMYB TFs (). The protein structures of α-helices, random coils and extended strands were consistent in the same subgroup. The content of random coil was the highest with an average of 58%. The α-helix contents of the other four predicted TFs were 20–27%. The extended strand contents were similar in the three members of subgroup 22 and the two members of subgroup 20. There were no β-turns in the predicted CsMYB TFs. In conclusion, the main elements of the secondary structure in subgroups 20 and 22 were random coils, α-helices, and extended strands.
Table 2. Secondary structure prediction of the five CsMYB transcription factors.
As illustrated in , the characteristic motifs of these five CsMYB TFs were highly conserved. Two conserved structural motifs were detected in the N-terminus, and the number of amino acids was approximately 50–53. CsMYB15 also contained an HNF-1-N domain in the C-terminus ().
Phylogenetic analysis of the CsMYB proteins
An unrooted phylogenetic tree was constructed to analyze the evolutionary relationships of the R2R3-MYB protein in tea plants with that in maize, rice, and Arabidopsis. As shown in , CsMYB8 and CsMYB20 were classified into subgroup 20, whereas CsMYB15, CsMYB19, and CsMYB21 were clustered into subgroup 22. The tea plant was closely related to dicotyledonous Arabidopsis but different from monocotyledonous maize and rice.
Figure 3. Phylogenetic tree and conserved motif analysis of R2R3 MYB protein from tea plant (C. sinensis), Arabidopsis (A. thaliana), rice (O. sativa), and maize (Z. mays).
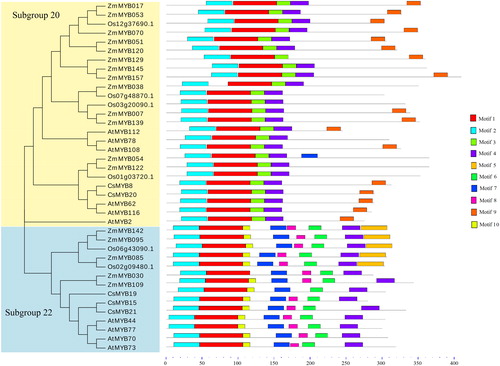
In the case of domain, the amino acid sequences of the 20 and 22 subgroups among C. sinensis, A. thaliana, Z. mays, and O. sativa were predicted using the MEME website (). Motif 1 and motif 2 of the two subgroups were conserved. The motifs at the C-terminus of the TFs in subgroup 20 were less than in subgroup 22, which only includes motif 3, motif 4, and motif 9. By contrast, the members of subgroup 22 were predicted to contain many different motifs at the C-terminus. The functions of those different motifs were not defined (). This finding was consistent with the conclusion that R2R3-type MYB TFs were conserved at the N-terminus and composed of two incomplete motifs. On the contrary, the C-terminal region of all sequences was flexible and responsible for regulating protein activity [Citation25]. In soybean, Du et al. [Citation33] observed that the C-terminal motifs from different subfamilies exhibit specific characteristics.
Table 3. Regular expression of conserved motifs from CsMYB proteins.
Subcellular localization analysis of CsMYB19
Under the control of the CaMV 35S promoter, CsMYB19 gene was inserted into the vector containing the GFP reporter gene. The CsMYB19-GFP fusion gene and GFP recombinant constructs as contrast were introduced into onion epidermal cells through particle bombardment. The results showed that the CsMYB19-GFP fusion protein was specifically localized in the nucleus with a large proportion in the nucleolus and a small proportion in other places of the nucleus (). The empty vector GFP showed ubiquitous distribution in the whole cell. The subgroup 22 gene, AtMYB44, was located in the nuclei [Citation40], thus indicating that CsMYB19 was also located in the nuclei.
Expression profiles of CsMYB genes in response to abiotic stress in tea plants
Abiotic stress seriously threatens the growth and development of tea plants [Citation11, Citation12, Citation66, Citation67]. R2R3-type MYB TFs participate in abiotic stress response in plants and regulate related gene expression during exposure to abiotic stress [Citation24, Citation68]. The genes from subgroups 20 and 22 of the R2R3 type MYB family participate in abiotic stress response [Citation38, Citation39, Citation41–43]. For instance, MYB2 regulates a specific CaM isoform, which mediates salt-induced Ca2+ signalling in tolerating salt stress [Citation69]. qRT-PCR analysis was performed to detect the expression levels of the five CsMYB genes CsMYB8, CsMYB20, CsMYB15, CsMYB19 and CsMYB21 and explore their expression patterns under various abiotic stresses.
High temperature treatment
Under heat treatment (), the expression profiles of the five CsMYB genes were gradually up-regulated. CsMYB8 and CsMYB20 were significantly up-regulated compared with the rest of the studied genes and reached the highest level at 24 h. CsMYB15 and CsMYB21 were first down-regulated after 2 h and then were up-regulated again. CsMYB19 was continuously up-regulated and reached the highest level at 24 h but still had lower expression than the two genes (CsMYB8 and CsMYB20) from subgroup 20.
Low temperature treatment
Under the low temperature treatment (), CsMYB8 and CsMYB20 were highly up-regulated and reached the highest level at 24 h. The relative expression levels of other genes were first up-regulated at 4 h and then down-regulated at 8 h. The expression of CsMYB19 was highest at 4 h and was 41.41 times higher than that of the control. The CsMYB8 expression was highest at 24 h and was 38.72 times higher than that of the control. The expression levels of CsMYB8, CsMYB19, and CsMYB20 were higher than those of CsMYB15 and CsMYB21.
Drought treatment
In the drought treatment experiments (), the expression levels of the five CsMYB genes were up-regulated at 1 and 8 h. All of other genes showed the highest level at 8 h, except for CsMYB21 with the highest level at 1 h. The expression levels of CsMYB8 and CsMYB19 exhibited an increasing trend at 1 h and were then slightly down-regulated at 2 and 4 h. The CsMYB8 and CsMYB19 genes showed the highest expression level at 8 h and then decreased at 24 h. The response to drought stress involved a complex gene regulatory system in tea plants. Here, the expression trends of CsMYB8 and CsMYB19 under drought treatments were maybe due to the complexity of the response to drought conditions in tea plants. The expression profiles showed that the highest expression levels of CsMYB genes were in the successive order of CsMYB20 > CsMYB19 > CsMYB8 > CsMYB21 > CsMYB15.
Salt treatment
Under salt treatment (), the expression level of CsMYB8 exhibited an increasing trend and was the highest at 24 h, which was 27 times higher than that of the control. By contrast, the rest of the genes were up-regulated at 8 h and down-regulated at 24 h. The results showed that the expression level of CsMYB8 was the highest, followed by that of CsMYB20.
The members of subgroup 20 showed higher responses than those of subgroup 22 under abiotic stress. In addition, the CsMYB genes were more sensitive to extreme temperature than to drought and salt. The results indicated the important roles of subgroup 20 members in regulating abiotic stress. This finding is consistent with previous reports on A. thaliana [Citation16, Citation24, Citation25, Citation31].
Expression profiles of CsMYB genes in response to hormonal treatment
Tea plants were treated with SA (1 mmol L−1), GA3 (1 mmol L−1), MeJA (1 mmol L−1), ABA (0.1 mmol L−1) and IAA (1 mmol L−1) to determine the effect of different hormonal treatments on the expression levels of CsMYB genes.
The expression level of CsMYB20 was significantly up-regulated under all hormonal treatments. Moreover, the highest expression level was observed under ABA treatment, which was 3.10 times higher than that of the control. With regard to the response to hormonal treatments, the expression levels of CsMYB20 showed a successive order of ABA > GA > MeJA > IAA > SA. The lowest expression level was under SA treatment, which was 1.17 times higher than that of the control. CsMYB8 was up-regulated under MeJA treatment, and the level was 11.03 times higher than that of the control; however, the expression level of CsMYB8 under other hormonal treatments was lower than that of the control. The CsMYB8 expression was induced by MeJA hormone treatment and was inhibited under the other four hormone treatments. The expression levels of CsMYB genes from subgroup 20 were lower than that of the control and were inhibited under five different hormone treatments. As illustrated in , the expression levels of CsMYB19 and CsMYB21 under MeJA treatment were higher than those under other hormonal treatments and were 0.76 and 0.47 times higher than that of the control, respectively.
In conclusion, the genes from subgroup 22 were inhibited in varying degrees under all hormonal treatments. The CsMYB20 expression was highest under ABA treatment and was lowest under SA treatment, but still was higher than that of the control. The CsMYB8 expression was 11.03 times higher than that of the control under MeJA treatment and was inhibited under the other hormonal treatments. Different hormone treatments showed various degrees of inhibition on the CsMYB genes from subgroup 22. We speculated that CsMYB15, CsMYB19, and CsMYB21 from subgroup 22 were negatively regulated by the hormonal treatments.
The AtMYB2 gene from subgroup 20 encodes a protein that recognizes the sequence of ‘TAACTG’. This sequence is found in the 5′-upstream regions of many ABA-responsive genes, and AtMYB2 is responsive to dehydration [Citation70]. Similarly, the homologous gene CsMYB20 exhibited high expression level under hormonal and abiotic stress treatments, especially upon ABA treatment. In conclusion, CsMYB20 might also be involved in recognizing the conservative motif and inducing the expression of ABA-responsive genes in tea plants. For abiotic stress treatments, the CsMYB gene expression levels were significantly higher than that of the control, and the relative expression levels of the subgroup 20 genes were higher than those of the subgroup 22 genes. This finding is consistent with previous reports, indicating that subgroups 20 and 22 play an important role in plant resistance to stress [Citation22, Citation71–73]. The expression level of the CsMYB genes were highly regulated under abiotic stress but not under hormone treatments, suggesting that the CsMYB genes may have other regulatory pathways of promoting their expression.
Conclusions
In this study, five CsMYB genes encoding TFs were cloned from the tea plant cultivar Longjing 43. A phylogenetic relationship was studied. The expression profiles of the CsMYB genes under abiotic stresses and hormonal treatment were detected, and the expression levels of subgroup 20 genes were always higher than those of the subgroup 22 genes. We speculated that subgroup 20 genes have higher response than subgroup 22 genes under abiotic stress, and the expression levels of CsMYB15, CsMYB19, and CsMYB21 from subgroup 22 were negatively regulated under different hormonal treatments in tea plants. These results offered a useful framework for further research to understand the evolution of 20 and 22 R2R3-MYB subgroups in tea plants. CsMYB19-GFP fusion protein was specifically localized in the nucleus. Our research lays a foundation for future research on abiotic stress with MYB TFs in tea plant.
Disclosure statement
No potential conflict of interest was reported by the authors.
Additional information
Funding
References
- Ogden CL, Carroll MD, Curtin LR, et al. Prevalence of overweight and obesity in the united states, 1999-2004. JAMA. 2006;295(13):1549–1555.
- Nagao T, Hase T, Tokimitsu I. A green tea extract high in catechins reduces body fat and cardiovascular risks in humans. Obesity (Silver Spring). 2006;15(6):1473–1483.
- Auvichayapat P, Prapochanung M, Tunkamnerdthai O, et al. Effectiveness of green tea on weight reduction in obese Thais: a randomized, controlled trial. Physiol Behav. 2008;93(3):182–189.
- Hertog M, Hollman P, Katan MB, et al. Intake of potentially anticarcinogenic flavonoids and their seterminants in adults in the netherlands. Nutr Cancer. 1993;20:21–29.
- Soobrattee MA, Bahorun T, Aruoma OI, et al. Chemopreventive actions of polyphenolic compounds in cancer. Biofactors. 2006;27(1–4):19–35.
- Nakachi K, Matsuyama S, Miyake S, et al. Preventive effects of drinking green tea on cancer and cardiovascular disease: epidemiological evidence for multiple targeting prevention. Biofactors. 2000;13(1–4):49–54.
- Ryu OH, Lee J, Lee KW, et al. Effects of green tea consumption on inflammation, insulin resistance and pulse wave velocity in type 2 diabetes patients. Diabetes Res Clin Pract. 2006;71(3):356–358.
- Crespy V, Williamson G. A review of the health effects of green tea catechins in in vivo animal models. J Nutr. 2004;134(12):S3431–S3440.
- Iso H, Date C, Wakai K, et al. The relationship between green tea and total caffeine intake and risk for self-reported type 2 diabetes among Japanese adults. Annals Intern Med. 2006;144(8):554–562.
- Roche HM, Seagrove S, Mehta A, et al. Using natural dietary sources of antioxidants to protect against ultraviolet and visible radiation-induced DNA damage: an investigation of human green tea ingestion. J Photochem Photobiol B. 2010;101(2):169–173.
- Cheong YH, Chang HS, Gupta R, et al. Transcriptional profiling reveals novel interactions between wounding, pathogen, abiotic stress, and hormonal responses in Arabidopsis. Plant Physiol. 2002;129(2):661–677.
- Xiong LS, Schumaker KS, Zhu JK. Cell signaling during cold, drought, and salt stress. Plant Cell. 2002;14(Suppl. 1):s164–s183.
- Vyas D, Kumar S. Tea (Camellia sinensis (L.) O. Kuntze) clone with lower period of winter dormancy exhibits lesser cellular damage in response to low temperature. Plant Physiol Biochem. 2005;43(4):383–388.
- Wang L, Li XW, Zhao Q, et al. Identification of genes induced in response to low-temperature treatment in tea leaves. Plant Mo Biol Rep. 2008;27(3):257–265.
- Das A, Das S, Mondal TK. Identification of differentially expressed gene profiles in young roots of tea [Camellia sinensis (L.) O. Kuntze] subjected to drought stress using suppression subtractive hybridization. Plant Mol Biol Rep. 2012;30(5):1088–1101.
- Dubos C, Stracke R, Grotewold E, et al. MYB transcription factors in Arabidopsis. Trends Plant Sci. 2010;15(10):573–581.
- Cominelli E, Galbiati M, Vavasseur A, et al. A guard-cell-specific MYB transcription factor regulates stomatal movements and plant drought tolerance. Curr Biol. 2005;15(13):1196–1200.
- Jung C, Seo JS, Han SW, et al. Overexpression of AtMYB44 enhances stomatal closure to confer abiotic stress tolerance in transgenic Arabidopsis. Plant Physiol. 2008;146(2):623–635.
- Matus JT, Aquea F, Arce-Johnson P. Analysis of the grape MYB R2R3 subfamily reveals expanded wine quality-related clades and conserved gene structure organization across Vitis and Arabidopsis genomes. BMC Plant Biol. 2008;8(1):1471–2229.
- Akagi T, Ikegami A, Tsujimoto T, et al. DkMyb4 is a Myb transcription factor involved in proanthocyanidin biosynthesis in persimmon fruit. Plant Physiol. 2009;151(4):2028–2045.
- Xu ZS, Feng K, Que F, et al. A MYB transcription factor, DcMYB6, is involved in regulating anthocyanin biosynthesis in purple carrot taproots. Sci Rep. 2017;7:45324.
- Li CN, Ng CKY, Fan LM. MYB transcription factors, active players in abiotic stress signaling. Environ Exp Bot. 2015;114:80–91.
- Martin C, Paz-ares J. MYB transcription factors in plants. Trends Genet. 1997;13(2):0168–9525.
- Kranz HD, Denekamp M, Greco R, et al. Towards functional characterisation of the members of the R2R3-MYB gene family from Arabidopsis thaliana. Plant J. 1998;16(2):263–276.
- Jin HL, Martin C. Multifunctionality and diversity within the plant MYB-gene family. Plant Mol Biol. 1999;41(5):577–585.
- Katiyar A, Smita S, Lenka SK, et al. Genome-wide classification and expression analysis of MYB transcription factor families in rice and Arabidopsis. BMC Genom. 2012;13(1):1471–2165.
- Klempnauer KH, Gonda TJ, Bishop JM. Nucleotide sequence of the retroviral leukemia gene v-myb and its cellular progenitor c-myb: the architecture of a transduced oncogene. Cell. 1982;31(2):453–463.
- Rosinski JA, Atchley WR. Molecular evolution of the Myb family of transcription factors: evidence for polyphyletic origin. J Mol Evol. 1998;46(1):74–83.
- Weston K. Myb proteins in life, death and differentiation. Curr Opin Genet Dev. 1998;8(1):76–81.
- Riechmann JL, Heard J, Martin G, et al. Arabidopsis transcription factors: genome-wide comparative analysis among eukaryotes. Science. 2000;290(5499):2105–2110.
- Stracke R, Werber M, Weisshaar B. The R2R3-MYB gene family in Arabidopsis thaliana. Curr Opin Plant Biol. 2001;4(5):447–456.
- Chen YH, Yang XY, He K, et al. The MYB transcription factor superfamily of Arabidopsis: expression analysis and phylogenetic comparison with the rice MYB family. Plant Mol Biol. 2006;60(1):107–124.
- Du H, Feng BR, Yang SS, et al. The R2R3-MYB transcription factor gene family in maize. Plos One. 2012;7(6):e37463.
- Wilkins O, Nahal H, Foong J, et al. Expansion and diversification of the Populus R2R3-MYB family of transcription factors. Plant Physiol. 2009;149(2):981–993.
- Du H, Yang SS, Liang Z, et al. Genome-wide analysis of the MYB transcription factor superfamily in soybean. BMC Plant Biol. 2012;12(1):1471–2229.
- He QL, Jones DC, Li W, et al. Genome-wide identification of R2R3-MYB genes and expression analyses during abiotic stress in Gossypium raimondii. Sci Rep. 2016;6:22980.
- Saha G, Park JI, Ahmed NU, et al. Characterization and expression profiling of MYB transcription factors against stresses and during male organ development in Chinese cabbage (Brassica rapa ssp. pekinensis). Plant Physio Biochem. 2016;104:200–215.
- Urao T, Noji MA, Yamaguchi-Shinozaki K, et al. A transcriptional activation doman of ATMYB2, a drought-inducible Arabidopsis Myb-related protein. Plant J. 1996;10(6):1145–1148.
- Abe H, Urao T, Ito T, et al. Arabidopsis AtMYC2 (bHLH) and AtMYB2 (MYB) function as transcriptional activators in abscisic acid signaling. Plant Cell Online. 2002;15(1):63–78.
- Liu RX, Chen L, Jia ZH, et al. Transcription factor AtMYB44 regulates induced expression of the ethylene insensitive2 gene in Arabidopsis responding to a harpin protein. Mol Plant Microbe Interact. 2011;24(3):377–389.
- Agarwal M, Hao YJ, Kapoor A, et al. A R2R3 type MYB transcription factor is involved in the cold regulation of CBF genes and in acquired freezing tolerance. J Biol Chem. 2006;281(49):37636–37645.
- Ding ZH, Li S, An XL, et al. Transgenic expression of MYB15 confers enhanced sensitivity to abscisic acid and improved drought tolerance in Arabidopsis thaliana. J Genet Genom. 2009;36(1):17–29.
- Jia J, Xing JH, Dong JG, et al. Functional analysis of MYB73 of Arabidopsis thaliana against Bipolaris oryzae. Agr Sci China. 2011;10(5):721–727.
- Wu ZJ, Li XH, Liu ZW, et al. Transcriptome-based discovery of AP2/ERF transcription factors related to temperature stress in tea plant (Camellia sinensis). Funct Integr Genom. 2015;15(6):741–752.
- Wu ZJ, Li XH, Liu ZW, et al. De novo assembly and transcriptome characterization: novel insights into catechins biosynthesis in Camellia sinensis. BMC Plant Biol. 2014;14(1):1471–2229.
- Liu ZW, Wu ZJ, Li XH, et al. Identification, classification, and expression profiles of heat shock transcription factors in tea plant (Camellia sinensis) under temperature stress. Gene. 2016;576(1):52–59.
- Pfaffl MW. A new mathematical model for relative quantification in real-time RT-PCR. Nucleic Acid Res. 2001;29(9):2003–2007.
- Jin JP, Tian F, Yang DC, et al. Plant TFDB 4.0: toward a central hub for transcription factors and regulatory interactions in plants. Nucleic Acids Res. 2017;45(D1):D1040–D1045.
- Tamura K, Peterson D, Peterson N, et al. MEGA5: molecular evolutionary genetics analysis using maximum likelihood, evolutionary distance, and maximum parsimony methods. Mol Biol Evol. 2011;28(10):2731–2739.
- Bailey TL, Williams N, Misleh C, et al. MEME: discovering and analyzing DNA and protein sequence motifs. Nucleic Acids Res. 2006;34(Web Server Issue):W369–W373.
- Li MY, Xu ZS., Tian C, et al. Genomic identification of WRKY transcription factors in carrot (Daucus carota) and analysis of evolution and homologous groups for plants. Sci Rep. 2016;6:23101.
- Martin C, Bhatt K, Baumann K, et al. The mechanics of cell fate determination in petals. Philos Trans R Soc Lond B Biol Sci. 2002;357(1422):809–813.
- Gómez-Maldonado J, Avila C, Torre F, et al. Functional interactions between a glutamine synthetase promoter and MYB proteins. Plant J. 2004;39(4):513–526.
- Wang KL, Bolitho K, Grafton K, et al. An R2R3 MYB transcription factor associated with regulation of the anthocyanin biosynthetic pathway in Rosaceae. BMC Plant Biol. 2010;10(1):1471–2229.
- Paz-Ares J, Ghosal D, Wienand U, et al. The regulatory c1 locus of Zea mays encodes a protein with homology to myb proto-oncogene products and with structural similarities to transcriptional activators. EMBO J. 1987;6(12):3553–3558.
- Devaiah BN, Madhuvanthi R, Karthikeyan AS, et al. Phosphate starvation responses and gibberellic acid biosynthesis are regulated by the MYB62 transcription factor in Arabidopsis. Mol Plant. 2009;2(1):43–58.
- Guo YF, Gan SS. AtMYB2 regulates whole plant senescence by inhibiting cytokinin-mediated branching at late stages of development in Arabidopsis. Plant Physiol. 2011;156(3):1612–1619.
- Kim JH, Nguyen NH, Jeong CY, et al. Loss of the R2R3 MYB, AtMyb73, causes hyper-induction of the SOS1 and SOS3 genes in response to high salinity in Arabidopsis. J Plant Physiol. 2013;170(16):1461–1465.
- Deluc L, Barrieu F, Marchive C, et al. Characterization of a grapevine R2R3-MYB transcription factor that regulates the phenylpropanoid pathway. Plant Physiol. 2006;140(2):499–511.
- Zhao L, Gao LP, Wang HX, et al. The R2R3-MYB, bHLH, WD40, and related transcription factors in flavonoid biosynthesis. Funct Integr Genom. 2013;13(1):75–98.
- Millar AA, Gubler F. The arabidopsis GAMYB-like genes, MYB33 and MYB65, are microRNA-regulated genes that redundantly facilitate anther development. Plant Cell Online. 2005;17(3):705–721.
- Baumann K, Perez-Rodriguez M, Bradley D, et al. Control of cell and petal morphogenesis by R2R3 MYB transcription factors. Development. 2007;134(9):1691–1701.
- Mandaokar A, Browse J. MYB108 acts together with MYB24 to regulate jasmonate-mediated stamen maturation in Arabidopsis. Plant Physiol. 2008;149(2):851–862.
- Kang YH, Kirik V, Hulskamp M, et al. The MYB23 gene provides a positive feedback loop for cell fate specification in the Arabidopsis root epidermis. The Plant Cell Online. 2009;21(4):1080–1094.
- Boyer LA, Latek RR, Peterson CL. The SANT domain: a unique histone-tail-binding module? Nat Rev Mol Cell Biol. 2004;5(2):158–163.
- Zhou L, Xu H, Mischke S, et al. Exogenous abscisic acid significantly affects proteome in tea plant (Camellia sinensis) exposed to drought stress. Hortic Res. 2014;1:14029.
- Li H, Liu ZW, Wu ZJ, et al. Differentially expressed protein and gene analysis revealed the effects of temperature on changes in ascorbic acid metabolism in harvested tea leaves. Hortic Res. 2018 [Feb 25]. DOI:10.1038/s41438-018-0070-x.
- Rabbani MA, Maruyama K, Abe H, et al. Monitoring expression profiles of rice genes under cold, drought, and high-salinity stresses and abscisic acid application using cDNA microarray and RNA gel-blot analyses. Plant Physiol. 2003;133(4):1755–1767.
- Yoo JH, Park CY, Kim JC, et al. Direct interaction of a divergent CaM isoform and the transcription factor, MYB2, enhances salt tolerance in arabidopsis. J Biol Chem. 2004;280(5):3697–3706.
- Urao T, Yamaguchi-Shinozaki K, Urao S, et al. An Arabidopsis myb homolog is induced by dehydration stress and its gene product binds to the conserved MYB recognition sequence. Plant Cell. 1993;5(11):1529–1539.
- Seo JS, Soho HB, Noh K, et al. Expression of the Arabidopsis AtMYB44 gene confers drought/salt-stress tolerance in transgenic soybean. Mol Breeding. 2012;29(3):601–608.
- Yang A, Dai XY, Zhang WH. A R2R3-type MYB gene, OsMYB2, is involved in salt, cold, and dehydration tolerance in rice. J Exp Bot. 2012;63(7):2541–2556.
- Jaradat MR, Feurtado JA, Huang DQ, et al. Multiple roles of the transcription factor AtMYBR1/AtMYB44 in ABA signaling, stress responses, and leaf senescence. BMC Plant Biol. 2013;13(1):1471–2229.