Abstract
The plant-specific LBD (LATERAL ORGAN BOUNDARIES DOMAIN) genes belong to a major family of transcription factors that encode a zinc finger-like domain. This family plays crucial roles in plant development, including lateral organ development, pathogen response, pollen development, plant regeneration and hormone response. However, there is no detailed information of LBD genes in the tea plant. Tea plant [Camellia sinensis (L.) O. Kuntze] is a perennial evergreen woody crop that is cultivated worldwide. Tea leaves are used as a non-alcoholic beverage, which is known for its beneficial ingredients and multiple flavours. In this study, a total of 31 CsLBD genes were identified and characterized based on the genome of C. sinensis. CsLBD factors and Arabidopsis counterparts were clustered into 10 subgroups after phylogenetic analysis. Conserved motif analysis revealed that CsLBD proteins with a close evolutionary relationship possessed uniform or similar motif compositions. The interaction networks of LBD proteins in C. sinensis were established according to the data in Arabidopsis. Seven CsLBD genes were examined through qRT-PCR (quantitative real time polymerase chain reaction) in tea plant cultivar, ‘Longjing43’. The expression profiles of these CsLBD genes were investigated in different tissues (i.e. root, stem, leaf and flower) and subjected to five hormonal stimuli (i.e. abscisic acid, gibberellin acid, ethephon, methyl jasmonate and salicylic acid). This study provides an extensive overview of the CsLBD family genes and a valuable foundation for future functional studies of CsLBD factors.
Introduction
In plants, the transcription factor (TF) families have been implicated in many important biological processes. The LBD (LATERAL ORGAN BOUNDARIES DOMAIN) gene family is one of the plant-specific TF families in plants. These TFs have a highly conserved, approximately 100-amino acid LOB domain, which acts in the regulation of lateral organ development and metabolic processes in various plants [Citation1,Citation2]. In Arabidopsis, the LBD protein family is divided into two classes, class I and class II, based on the structure of the LOB domain in the N terminus [Citation3,Citation4]. Class I proteins contain a completely conserved CX2CX6CX3C zinc-finger-like domain and an LX6LX3LX6L Leucine zipper-like coiled-coil motif; whereas class II members only have the zinc-finger-like motif [Citation4,Citation5]. The zinc finger-like domain is thought to be required for DNA binding, and the C-terminal leucine zipper-like sequence is probably involved in protein dimerization [Citation2,Citation4].
The first LBD gene was identified in Arabidopsis thaliana based on the distinctive gene expression pattern of an enhancer trap insertion [Citation1,Citation6]. To date, functional analysis of the LBD family members has been performed in several plants, such as A. thaliana, apple (Malus domestica), rice (Oryza sativa), maize (Zea mays), soybean (Glycine max), grape (Vitis vinifera) and barley (Hordeum vulgare) [Citation3,Citation4,Citation7–11].
LBD genes have varied expression patterns ranging from temporal to tissue differences; moreover, they may function in diverse processes [Citation1,Citation2,Citation4,Citation12,Citation13]. ASL18/LBD16 contains two domains comprising an atypical nuclear localization signal (NLS) with basic amino acid residues in the coiled-coil motif and a monopartite-like NLS in the C-terminal region for nuclear targeting [Citation14]. The AtLOB gene encodes a DNA-binding protein and can interact with the members of the bHLH (basic helix-loop-helix) family TFs [Citation15]. Numerous LBD genes were expressed in specific regions of the adaxial base of lateral tissues, shoot apical meristem, indicating that they play critical roles in the development of the plant side tissues [Citation1,Citation2,Citation16,Citation17]. Some AS2/LOB genes in Arabidopsis, ASL1/LBD36, ASL4/LOB and ASL5/LBD12, are involved in the development of a range of lateral tissues from the meristem [Citation1,Citation17,Citation18]. LBD genes play a role in different aspects of root development including lateral root development in monocots and dicots [Citation19–21]. The maize Ramosa2 gene plays a role in regulation of inflorescence architecture [Citation22]. In Arabidopsis, the activity of the auxin response factors AtARF7 and AtARF19, interacted with AtMYB77 could induce the formation of lateral roots [Citation23].
LBD genes were also involved in plant anthocyanin biosynthesis, nitrate metabolism as well as hormone-mediated plant lateral organ development [Citation24–26]. In Arabidopsis, three genes including AtLBD37, AtLBD38 and AtLBD39, are involved in anthocyanin biosynthesis and nitrate metabolism [Citation24]. In rice, OsLBD37 is also associated with nitrogen metabolism [Citation25]. AtARF7 and AtARF19 directly induced AtLBD29 and AtLBD16 expression in the formation of lateral roots [Citation19]. The ARL1 gene promoted the formation of adventitious roots in rice induced by auxin [Citation21]. AtLBD3 was identified as a primary target of cytokinin-mediated phosphorelay signal transduction, indicating that this gene plays an important role in cytokinin-responsive biological events in plants [Citation27].
The tea plant [Camellia sinensis (L.) O. Kuntze] is a perennial evergreen woody crop cultivated worldwide [Citation28]. Tea leaves are one of the most important resources used as a non-alcoholic beverage, which is known for its beneficial ingredients and multiple flavours that are good for human health [Citation29,Citation30]. The accumulation and transformations of substances in tea depends on the biological process of growth and development [Citation31–33]. Many studies have revealed numerous LBD genes involved in the response of plant growth and hormone signalling. However, there is little available information to clarify the cellular and molecular mechanisms of tissue development and hormonal regulation of LBD genes in tea plants.
This study aimed to explore the LBD gene family in the tea plant. A total of 31 CsLBD genes were identified based on the genome database of the tea plant [Citation34]. Subsequently, a comprehensive analysis of multiple sequence alignments, phylogenetic analysis and conserved motif prediction were performed. Quantitative real-time PCR (qRT-PCR) was used to detect the expression patterns of CsLBD genes in various tissues (leaves, stems, roots and flowers) and in response to various hormone stimuli: abscisic acid (ABA), gibberellic acid (GA3), ethephon (Eth), methyl jasmonate (MeJA), and salicylic acid (SA), in ‘Longjing43’, one of the widely planted tea plant cultivars in China. The results will provide a reference foundation for future functional studies of CsLBD genes in the tea plant.
Materials and methods
Plant materials and treatments
Two-year-old propagated cuttings of tea plants (C. sinensis cv. ‘Longjing 43’) were grown in a growth chamber at the Tea Science Research Institute of Nanjing Agricultural University (Nanjing, China). The artificial climate condition was programmed with 70% relative humidity, 14/10-hour light/dark cycle and 23 °C temperature. Tea plants were subjected to various hormone treatments. The details were as follows: leaves were sprayed once with 1 mmol/L gibberellic acid (GA3 treatment), 1 mmol/L salicylic acid (SA treatment), 1 mmol/L methyl jasmonate (MeJA treatment), 0.1 mmol/L abscisic acid (ABA treatment) or 0.1 mmol/L ethephon (Eth treatment), respectively. The young leaves of all tested plants were harvested at 0, 2, 4, 8 and 24 hours after the treatments. To study the expression patterns of CsLBD genes in different tissues, leaves, stems, roots and flowers were collected from growing plants under normal conditions. Three biological replicates were prepared. All samples were immediately frozen in liquid nitrogen and then stored at −80 °C until RNA extraction.
Retrieval and control of sequences
All CsLBD genes of the tea plant were retrieved from the C. sinensis genome database [Citation34]. All of the CsLBD genes were confirmed by NCBI Blast analysis. The amino acid sequences of all the LBD proteins of Arabidopsis were downloaded from the Plant Transcription Factor Database (PlantTFDB) v3.0 (http://planttfdb.cbi.pku.edu.cn/) (Supplementary Information ) [Citation35].
Table 1 Primers for qRT-PCR of CsLBD genes.
Multiple sequence alignment, phylogenetic analysis and conserved motif analysis
To survey the phylogenetic relationships of LBD proteins in the tea plant, the domain sequences of the LBD proteins in the tea plant and Arabidopsis were used to construct a phylogenetic tree. After multiple alignments were carried out, phylogenetic trees were generated and displayed by the MEGA program (version 5.0) with the following parameters: neighbour-joining (NJ) method, p-distance model, pairwise deletio, and 1000 bootstrap replicates [Citation36]. The Multiple Em for Motif Elicitation (MEME) suite (version 4.12.0) (http://meme-suite.org/tools/meme) was used to identify the conserved motifs, and the default parameters were used, in addition to the maximum number of motifs (20) [Citation37]. The predicted protein–protein interactions were constructed by using STRING (version10.0) (http://string-db.org/). A. thaliana was selected as the model organism of STRING [Citation38].
RNA isolation, cDNA reverse transcription and qRT-PCR analysis
Each RNA sample from tea plant materials was extracted using the RNA Isolation Kit (Huayueyang, Beijing, China) in accordance with the manufacturer’s instructions. Before cDNA synthesis, RNA integrity was verified using 1.5% denaturing agarose gel, and the purity and concentration were measured on a NanoDrop ND-2000 Spectrophotometer (Thermo Scientific, USA). Each RNA sample was reverse transcribed into complementary DNA (cDNA) using the PrimeScript RT reagent Kit with gDNA Eraser (TaKaRa, Dalian, China).
Each sample was run in triplicate. CsTBP served as the internal control gene [Citation39]. The SYBR Premix Ex Taq kit (TaKaRa, Dalian, China) was used for qRT-PCR on the Bio-rad IQ5 fluorescence quantitative PCR platform. The experiments were completed in a volume of 20 μL: 0.4 μL of each specific primer, 10 μL of 2 × SYBR Premix, 2 μL of diluted cDNA as a template and 7.2 μL of ddH2O. The qRT-PCR conditions were as follows: denaturation at 95 °C for 30 seconds, followed by 40 cycles of 95 °C for 10 seconds and 60 °C for 20 seconds. The melting curves (65–95 °C, at increments of 0.5 °C) were obtained to verify the amplification specificity. Detection primers were designed using the Primer Premier 5.0 software. The primer sequences used in this study are provided in .
Data analysis
All reactions were performed in triplicate, and the relative gene expression levels were calculated on the basis of the threshold cycle using the 2−ΔΔCt method [Citation40]. Data are presented as mean values with standard deviation (±SD). Statistical analysis was performed using SPSS 17.0 software (SPSS Inc., Chicago, IL, USA); significant differences between values were evaluated by Duncan’s multiple range test at 0.05 probability level (P < 0.05).
Results and discussion
Identification of CsLBD transcription factors
According to the tea plant genome database, a total of 31 sequences encoding CsLBD proteins were obtained (Supplementary Information ). All CsLBD genes were confirmed by NCBI Blast analysis. To determine the sequence features of LOB domains, multiple alignments of all CsLBD proteins were analyzed (Supplementary Information Figure S1). All of the CsLBD proteins had a conserved LOB domain in the N terminus, which was further divided into three conserved blocks, the C block, GAS block and a C-terminal leucine-zipper-like motif [Citation2].
Table 2 Regular expression levels of conserved motifs from CsLBD proteins
Sequence alignment, gene structure and conserved motifs of CsLBD
To identify and classify CsLBD TFs, we performed sequence alignment. On the basis of the structure of the LOB domain, the CsLBD family TFs could be divided into two classes, class I (25 members) and class II (6 members). The class I consensus sequence is CAACKFLRRKCX3C, and the class II consensus sequence is CNGCRVLRKGCX3C. The GAS block is 49 amino acids in length, beginning with an FX2VH motif and ending with a DP(V/I)YG motif only in class I.
Class I proteins contain two domains, the C domain contains four fully conserved Cys residues in the CX2CX6CX3C motif (zinc finger like domain), which is presumably required for DNA binding [Citation3]. The GAS block was between the C block and the LX6LX3LX6L leucine zipper-like block (L domain). The L domain was in the C-terminus acting as a probable protein dimerization domain [Citation1,Citation3]. Class II members only have the zinc finger like domain.
To further identify the potential conserved motifs, CsLBD proteins were analyzed using the MEME program ( and Supplementary Information Figure S2). Members with a close evolutionary relationship displayed uniform or similar motif compositions [Citation41]. Notably, motif 2 is embedded in the LOB domain, which exists in all LBD proteins (). All CsLBDs contained motif 1 except CsLBD8; motif 3 was widespread in class I; motif 4 was widespread in class II. Motif 3 may serve as a probable protein dimerization domain [Citation1]. Some motifs were varied. For instance, class II proteins were split into two groups according to the different distribution of motifs 6, 7 and 11, suggesting that those motifs may play key roles in specific functions. In addition, motif 13, 14 and 16 were uniquely found in class Ia (CsLBD18 and CsLBD28).
Previously, 10 putative motifs from G. max, 15 high-confidence motifs from Lotus japonicus and Medicago truncatula, 20 putative motifs from Eucalyptus grandis were predicted [Citation6,Citation9,Citation42]. Similarly, most of the conserved motifs were also observed at the N-terminus of the LOB domain region, whereas few motifs appeared at the diversified C-terminal region. The distribution of the conserved motifs indicated that the functional characteristics of LBD proteins may be mainly determined by the N-terminal conserved domain, whereas the C-terminal region may also be involved in determining the functions of these proteins.
Phylogenetic analysis of the LBD proteins in C. sinensis and A. thaliana
Motif analysis further supported the phylogenetic tree analysis, which was similar to the previous reports in A. thaliana, O. sativa and M. domestica [Citation3,Citation4,Citation7]. To clarify the phylogenetic relationships of LBD family proteins in the tea plant and Arabidopsis, an unrooted tree was established on the basis of the aligned LOB domain sequences. As shown in , the tea plant and Arabidopsis LBDs were divided into two clades, class I and class II. Within class I, the members could be further grouped into eight sub-classes (Ia–Ih), and the class II members were divided into two sub-classes (IIa and IIb).
In this study, Class Id was predicted to have six tea plant members (CsLBD1, 3, 5, 10, 13 and 24) and four Arabidopsis LBD members, respectively. The tea plant had more LBD members in class Id than Arabidopsis, suggesting that the woody tea plant maybe evolutionarily needs more class Id LBD genes to adapt to the developmental changes of leaves. This may be an evolution diversity of LBD genes between herbaceous Arabidopsis and the woody tea plant [Citation7,Citation43–46]. Phylogenetic analysis can be utilized to predict gene function [Citation47,Citation48]. In Arabidopsis, AtLBD37, AtLBD38 and AtLBD39 genes in Class IIb are involved in the regulation of nitrogen metabolism and anthocyanin synthesis, acting as repressors of anthocyanin synthesis and N availability signals in the plant [Citation24,Citation49]. CsLBD5 (ortholog of AtLBD6) gene may involve in early leaf development [Citation1,Citation50,Citation51]. AtLBD6 is not only involved in leaf development but also in flower development [Citation12].
Evolution of the LBD family among plants
In order to analyze the relationship between C. sinensis and other plants, the evolution of the LBD TFs in C. sinensis and other plants was studied (). Eleven species were included in the analysis: A. thaliana [Citation3], Populus trichocarpa [Citation44], Fragaria vesca [Citation52], M. domestica [Citation4], L. japonicus [Citation42], M. truncatula [Citation42], G. max [Citation9], V. vinifera [Citation10], E. grandis [Citation6], Z. mays [Citation8] and O. sativa [Citation7]. All of the species belong to monocots or eudicots. The proportion of LBD in eudicots was significantly higher than in monocots. The total number of LBDs was relatively lower in the tea plant than in other species. In addition, the LBDs distributed in Class I were more than the ones in Class II in these species.
Interaction network of LBD proteins in C. sinensis and A. thaliana
Plant growth is a developmental process that involves multiple genes and their interactions. To further understand the interaction between LBDs and other genes in the tea plant, CsLBD proteins were predicted associated with the Arabidopsis model using STRING software (). The leucine zipper-like motif may function in probable protein–protein interactions [Citation2]. Previously, it has been shown that members of MYB and bHLH proteins interact with AtLOB [Citation15]. The TF AtLOB could potentially interact with at least 10 proteins, indicating that it was very important in the regulation at the transcriptional level. The amino acid sequence of AtLOB showed a high similarity to the four CsLBD proteins (CsLBD1, 3, 10 and 13). Ectopic expression of the AtLOB gene causes alterations in the size, shape of the leaves and floral tissues and leads to male and female infertility. We found interactions among CsLBD5, CsLBD1, CsLBD3, CsLBD10 and CsLBD13, which might regulate the shoot apex development. AtLBD16, AtLBD18 and AtLBD29 are involved in the formation of lateral roots by being regulated by transcriptional activation of ARF7 and ARF19 [Citation13,Citation19,Citation53]. AtLBD16, AtLBD18 and AtLBD29 showed high similarities to CsLBD26, CsLBD17 and CsLBD18, respectively.
Tissue-specific expression profiles of CsLBD genes in tea plants
LBD genes are involved in various developmental processes [Citation2,Citation6]. In order to identify CsLBD genes involved in tea plant development and to characterize the expression patterns of CsLBD in the root, the stem, leaves and flowers of C. sinensis, we used qRT-PCR. Seven CsLBD genes were selected, including CsLBD2, CsLBD4, CsLBD8, CsLBD14, CsLBD16, CsLBD27 and CsLBD29.
Some of the genes showed similar expression patterns, but most of them exhibited different ones (). These genes were highly expressed in the roots except CsLBD4. According to previous functional studies, LBD genes affect the root development in maize and rice [Citation20,Citation21,Citation54]. AtLBD16, 18 and 29 participate in the auxin signal transduction cascade through direct or indirect regulation by AtARF7 and AtARF19, and then lead to the formation of lateral roots in Arabidopsis [Citation13,Citation19,Citation53]. CsLBD27 showed relatively high expression in the root and homology with AtLBD18, implying that this gene may be involved in lateral root formation in C. sinensis. CsLBD4 was highly expressed in the flowers of the tea plant, and AtLBD30 is thought to regulate the floral development in Arabidopsis [Citation55,Citation56], suggesting this gene probably has similar functions. CsLBD29 is predominately expressed in leaves, higher than other genes. These results indicated that the studied genes play a critical role in plant growth and development. Tissue-specific genes facilitate the development of specific tissues.
Expression profiles of CsLBD genes under various hormone treatments in tea plants
Hormones have a certain effect on plant growth and cause a series of morphological, physiological, biochemical and molecular changes [Citation2]. Considerable evidence shows that LBD genes play important roles in the response to hormones [Citation2]. To clarify the potential functions of CsLBD genes in response to different hormonal treatments, the expression patterns of seven selected CsLBD genes (CsLBD2, CsLBD4, CsLBD8, CsLBD14, CsLBD16, CsLBD27 and CsLBD29) were analyzed through qRT-PCR under five hormonal stimuli (i.e., ABA, GA3, SA, MeJA and Eth) in ‘Longjing43’ ().
Figure 6. Expression profiles of CsLBD genes in the tea cultivar ‘Longjing43’ under hormone treatments: ABA (0.1 mmol/L), GA3 (1 mmol/L), SA (1 mmol/L), MeJA (1 mmol/L), Eth (0.1 mmol/L). Note: Error bars represent standard deviation (±SD). The values indicated by different letters significantly differ at P < 0.05.
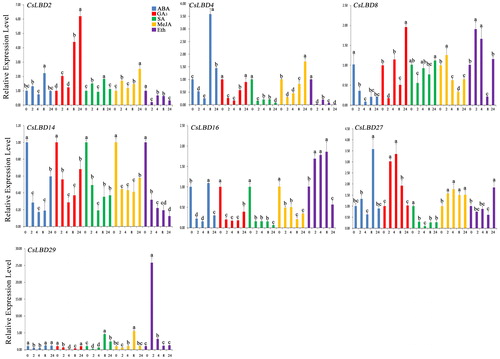
Under ABA treatment, the expression of CsLBD4 and CsLBD29 initially decreased and then increased. The expression patterns of CsLBD8 and CsLBD14 showed similar trends towards down-regulation. The seven CsLBD genes responded to ABA treatment, suggesting that these genes may regulate the response of tea plants in an ABA-dependent manner. CsLBD2 and CsLBD27 maintained high expression levels 24 hours after the GA3 treatment. CsLBD4, CsLBD14, CsLBD16 and CsLBD29 had the same trend: their expression levels were downregulated from 2 hours, but were then upregulated from 8 hours. The CsLBD genes in response to SA showed different expression regulation. CsLBD4, CsLBD14, CsLBD16 and CsLBD27 were downregulated. CsLBD2 and CsLBD29 were upregulated. Under MeJA treatment, CsLBD2, CsLBD27 and CsLBD29 were markedly upregulated. The expression trend of CsLBD8 was similar to that of CsLBD29 under Eth treatment, as both increased to the maximum level at 2 hours. EgLBD37 was upregulated under exogenous gibberellic acid treatment and may differentially regulate GA-mediated growth and developmental processes in E. grandis [Citation6]. GA perception and signal transduction also play an important role in GA-mediated plant growth and development [Citation57]. MdLBD40 was strongly upregulated under ABA treatment in apple [Citation4]. GmLBD12 and GmLBD23 responded to ABA and SA treatment [Citation9]. Overall the CsLBD genes were widely involved in various hormonal stimulations. In this study, seven CsLBD genes had differential functions in response to five hormone treatments. These results demonstrate that phytohormones play a critical role in normal plant growth and development.
Conclusions
In this study, we identified 31 CsLBD TFs from tea genome data. CsLBD proteins were divided into two classes. Class I was predicted to contain eight sub-classes and class II to contain two sub-classes. CsLBDs in the same subgroup share similar physicochemical properties. Bioinformatics analysis was used to predict the physicochemical properties of CsLBDs. qRT-PCR results showed that the CsLBD genes can respond to various hormonal stimuli in different tissues. Our study will provide useful information to further study the role of CsLBD TFs in the regulation mechanism in tea development. This study may serve as a basis in selecting candidate genes related to tea plant development and in further elucidating the functional roles of these CsLBD genes.
Disclosure Statement
The authors declare that they have no conflict of interest.
Additional information
Funding
References
- Shuai B, Reynaga-Pena CG, Springer PS. The lateral organ boundaries gene defines a novel, plant-specific gene family. Plant Physiol. 2002;129(2):747–761.
- Majer C, Hochholdinger F. Defining the boundaries: structure and function of LOB domain proteins. Trends Plant Sci. 2011;16(1):47–52.
- Matsumura Y, Iwakawa H, Machida Y, et al. Characterization of genes in the ASYMMETRIC LEAVES2/LATERAL ORGAN BOUNDARIES (AS2/LOB) family in Arabidopsis thaliana, and functional and molecular comparisons between AS2 and other family members. Plant J. 2009;58(3):525–537.
- Wang X, Zhang S, Su L, et al. A genome-wide analysis of the LBD (LATERAL ORGAN BOUNDARIES domain) gene family in Malus domestica with a functional characterization of MdLBD11. PLoS One. 2013;8(2):e57044. DOI: 10.1371/journal.pone.0057044
- Iwakawa H, Ueno Y, Semiarti E, et al. The ASYMMETRIC LEAVES2 gene of Arabidopsis thaliana, required for formation of a symmetric flat leaf lamina, encodes a member of a novel family of proteins characterized by cysteine repeats and a leucine zipper. Plant Cell Physiol. 2002;43(5):467–478.
- Lu Q, Shao FJ, Macmillan C, et al. Genomewide analysis of the lateral organ boundaries domain gene family in Eucalyptus grandis reveals members that differentially impact secondary growth. Plant Biotechnol J. 2018;16(1):124–136.
- Yang Y, Yu XB, Wu P. Comparison and evolution analysis of two rice subspecies LATERAL ORGAN BOUNDARIES domain gene family and their evolutionary characterization from Arabidopsis. Mol Phylogenet Evol. 2006;39(1):248–262.
- Zhang YM, Zhang SZ, Zheng CC. Genomewide analysis of LATERAL ORGAN BOUNDARIES domain gene family in Zea mays. J Genet. 2014;93(1):79–91.
- Yang H, Shi G, Du H, et al. Genome-wide analysis of soybean LATERAL ORGAN BOUNDARIES domain-containing genes: a functional investigation of GmLBD12. Plant Genome-US. 2017;10(1):1–19.
- Cao H, Liu CY, Liu CX, et al. Genomewide analysis of the lateral organ boundaries domain gene family in Vitis vinifera. J Genet. 2016;95(3):515–526.
- Guo BJ, Wang J, Lin S, et al. A genome-wide analysis of the ASYMMETRIC LEAVES2/LATERAL ORGAN BOUNDARIES (AS2/LOB) gene family in barley (Hordeum vulgare L.). J Zhejiang Univ Sci B. 2016;17(10):763–774.
- Xu B, Li Z, Zhu Y, et al. Arabidopsis genes AS1, AS2, and JAG negatively regulate boundary-specifying genes to promote sepal and petal development. Plant Physiol. 2008;146(2):566–575.
- Lee HW, Kim NY, Lee DJ, et al. LBD18/ASL20 regulates lateral root formation in combination with LBD16/ASL18 downstream of ARF7 and ARF19 in Arabidopsis. Plant Physiol. 2009;151(3):1377–1389.
- Kim MJ, Kim J. Identification of nuclear localization signal in ASYMMETRIC LEAVES2-LIKE18/LATERAL ORGAN BOUNDARIES DOMAIN16 (ASL18/LBD16) from Arabidopsis. J Plant Physiol. 2012;169(12):1221–1226.
- Husbands A, Bell EM, Shuai B, et al. LATERAL ORGAN BOUNDARIES defines a new family of DNA-binding transcription factors and can interact with specific bHLH proteins. Nucleic Acids Res. 2007;35(19):6663–6671.
- Sun SB, Song JP, Meng LS. ASYMMETRIC LEAVES2 gene, a member of LOB/AS2 family of Arabidopsis thaliana, causes an abaxializing leaves in transgenic cockscomb. Mol Biol Rep. 2012;39(4):4927–4935.
- Chalfun-Junior A, Franken J, Mes JJ, et al. ASYMMETRIC LEAVES2-LIKE1gene, a member of the AS2/LOB family, controls proximal-distal patterning in Arabidopsis petals. Plant Mol Biol. 2005;57(4):559–575.
- Nakazawa M, Ichikawa T, Ishikawa A, et al. Activation tagging, a novel tool to dissect the functions of a gene family. Plant J. 2003;34(5):741–750.
- Okushima Y, Fukaki H, Onoda M, et al. ARF7 and ARF19 regulate lateral root formation via direct activation of LBD/ASL genes in Arabidopsis. Plant Cell. 2007;19(1):118–130.
- Inukai Y, Sakamoto T, Ueguchi-Tanaka M, et al. Crown rootless1, which is essential for crown root formation in rice, is a target of an AUXIN RESPONSE FACTOR in auxin signaling. Plant Cell. 2005;17(5):1387–1396.
- Liu H, Wang S, Yu X, et al. ARL1, a LOB-domain protein required for adventitious root formation in rice. Plant J. 2005;43(1):47–56.
- Bortiri E, Chuck G, Vollbrecht E, et al. ramosa2 encodes a LATERAL ORGAN BOUNDARY domain protein that determines the fate of stem cells in branch meristems of maize. Plant Cell. 2006;18(3):574–585.
- Shin R, Burch AY, Huppert KA, et al. The Arabidopsis transcription factor MYB77 modulates auxin signal transduction. Plant Cell. 2007;19(8):2440–2453.
- Rubin G, Tohge T, Matsuda F, et al. Members of the LBD family of transcription factors repress anthocyanin synthesis and affect additional nitrogen responses in Arabidopsis. Plant Cell. 2009;21(11):3567–3584.
- Albinsky D, Kusano M, Higuchi M, et al. Metabolomic screening applied to rice FOX Arabidopsis lines leads to the identification of a gene-changing nitrogen metabolism. Mol Plant. 2010;3(1):125–142.
- Bell EM, Lin WC, Husbands AY, et al. Arabidopsis LATERAL ORGAN BOUNDARIES negatively regulates brassinosteroid accumulation to limit growth in organ boundaries. Proc Natl Acad Sci USA. 2012;109(51):21146–21151.
- Naito T, Yamashino T, Kiba T, et al. A link between cytokinin and ASL9 (ASYMMETRIC LEAVES 2 LIKE 9) that belongs to the AS2/LOB (LATERAL ORGAN BOUNDARIES) family genes in Arabidopsis thaliana. Biosci Biotechnol Biochem. 2007;71(5):1269–1278.
- Chen Y, Yu M, Xu J, et al. Differentiation of eight tea (Camellia sinensis) cultivars in China by elemental fingerprint of their leaves. J Sci Food Agr. 2009;89(14):2350–2355.
- Bordoni A, Hrelia S, Angeloni C, et al. Green tea protection of hypoxia/reoxygenation injury in cultured cardiac cells. J Nutr Biochem. 2002; 13(2):103–111.
- Yang CS, Wang X, Lu G, et al. Cancer prevention by tea: animal studies, molecular mechanisms and human relevance. Nat Rev Cancer. 2009; 9(6):429–439.
- Ashihara H, Deng WW, Mullen W, et al. Distribution and biosynthesis of flavan-3-ols in Camellia sinensis seedlings and expression of genes encoding biosynthetic enzymes. Phytochemistry. 2010; 71(5-6):559–566.
- Jiang XL, Liu YJ, Li WW, et al. Tissue-specific, development-dependent phenolic compounds accumulation profile and gene expression pattern in tea plant [Camellia sinensis]. PLoS One. 2013;8(4):e62315. DOI: 10.1371/journal.pone.0062315
- Liu M, Tian HL, Wu JH, et al. Relationship between gene expression and the accumulation of catechin during spring and autumn in tea plants (Camellia sinensis L.). Hortic Res. 2015;2(1):15023. DOI: 10.1038/hortres.2015.11
- Xia EH, Zhang HB, Sheng J, et al. The tea tree genome provides insights into tea flavor and independent evolution of caffeine biosynthesis. Mol Plant. 2017;10(6):866–877.
- Riano-Pachon DM, Ruzicic S, Dreyer I, et al. PlnTFDB: an integrative plant transcription factor database. BMC Bioinform. 2007;8:42. DOI: 10.1186/1471-2105-8-42
- Tamura K, Peterson D, Peterson N, et al. MEGA5: molecular evolutionary genetics analysis using maximum likelihood, evolutionary distance, and maximum parsimony methods. Mol Biol Evol. 2011;28(10):2731–2739.
- Bailey TL, Boden M, Buske FA, et al. MEME SUITE: tools for motif discovery and searching. Nucleic Acids Res. 2009;37(Web Server issue):W202–208.
- Szklarczyk D, Franceschini A, Wyder S, et al. STRING v10: protein-protein interaction networks, integrated over the tree of life. Nucleic Acids Res. 2015; 43(Database issue):D447–452.
- Wu ZJ, Tian C, Jiang Q, et al. Selection of suitable reference genes for qRT-PCR normalization during leaf development and hormonal stimuli in tea plant (Camellia sinensis). Sci Rep-UK. 2016;6:19748. DOI: 10.1038/srep19748
- Pfaffl MW. A new mathematical model for relative quantification in real-time RT–PCR. Nucleic Acids Res. 2001;29(9):2004–2007.
- Wang YX, Liu ZW, Wu ZJ, et al. Transcriptome-wide identification and expression analysis of the NAC gene family in tea plant [Camellia sinensis (L.) O. Kuntze]. PLoS One. 2016;11(11):e0166727. DOI:10.1371/journal.pone.0166727
- Yang T, Fang GY, He H, et al. Genome-wide identification, evolutionary analysis and expression profiles of LATERAL ORGAN BOUNDARIES DOMAIN gene family in Lotus japonicus and Medicago truncatula. PLoS One. 2016;11(8):e0161901. DOI:10.1371/journal.pone.0161901
- Schnable PS, Ware D, Fulton RS, et al. The B73 maize genome: complexity, diversity, and dynamics. Science. 2009;326(5956): 1112–1115.
- Zhu QH, Guo AY, Gao G, et al. DPTF: a database of poplar transcription factors. Bioinformatics. 2007;23(10):1307–1308.
- Zhuang J, Cai B, Peng RH, et al. Genome-wide analysis of the AP2/ERF gene family in Populus trichocarpa. Biochem Biophys Res Commun. 2008;371(3):468–474.
- Zhuang J, Peng RH, Cheng ZM, et al. Genome-wide analysis of the putative AP2/ERF family genes in Vitis vinifera. Sci Hortic-Amsterdam. 2009;123(1):73–81.
- Balazadeh S, Kwasniewski M, Caldana C, et al. ORS1, an H(2)O(2)-responsive NAC transcription factor, controls senescence in Arabidopsis thaliana. Mol Plant. 2011;4(2):346–360.
- Fang Y, You J, Xie K, et al. Systematic sequence analysis and identification of tissue-specific or stress-responsive genes of NAC transcription factor family in rice. Mol Genet Genomics. 2008;280(6):547–563.
- Scheible WR, Morcuende R, Czechowski T, et al. Genome-wide reprogramming of primary and secondary metabolism, protein synthesis, cellular growth processes, and the regulatory infrastructure of Arabidopsis in response to nitrogen. Plant Physiol. 2004;136(1):2483–2499.
- Dolan L, Langdale JA. New insights into plant development in New England. Development. 2004;131(21):5215–5220.
- Semiarti E, Ueno Y, Tsukaya H, et al. The ASYMMETRIC LEAVES2 gene of Arabidopsis thaliana regulates formation of a symmetric lamina, establishment of venation and repression of meristem-related homeobox genes in leaves. Development. 2001;128(10):1771–1783.
- Chen XD, Wang J, Zhao MZ, et al. Identification and expression analysis of LATERAL ORGAN BOUNDARIES DOMAIN (LBD) transcription factor genes in Fragaria vesca. Can J Plant Sci. 2018,98(2):288–299.
- Okushima Y, Overvoorde PJ, Arima K, et al. Functional genomic analysis of the AUXIN RESPONSE FACTOR gene family members in Arabidopsis thaliana: unique and overlapping functions of ARF7 and ARF19. Plant Cell. 2005; 17(2):444–463.
- Taramino G, Sauer M, Stauffer JL, et al. The maize (Zea mays L.) RTCS gene encodes a LOB domain protein that is a key regulator of embryonic seminal and post-embryonic shoot-borne root initiation. Plant J. 2007;50(4):649–659.
- Borghi L, Bureau M, Simon R. Arabidopsis JAGGED LATERAL ORGANS is expressed in boundaries and coordinates KNOX and PIN activity. Plant Cell. 2007;19(6):1795–1808.
- Soyano T, Thitamadee S, Machida Y, et al. ASYMMETRIC LEAVES2-LIKE19/LATERAL ORGAN BOUNDARIES DOMAIN30 and ASL20/LBD18 regulate tracheary element differentiation in Arabidopsis. Plant Cell. 2008;20(12):3359–3373.
- Wang GL, Xiong F, Que F, et al. Morphological characteristics, anatomical structure, and gene expression: novel insights into gibberellin biosynthesis and perception during carrot growth and development. Hortic Res. 2015;2(7):15028. DOI:10.1038/hortres.2015.28