Abstract
In this study, the exopolysaccharide from Bacillus amyloliquefaciens PB6 was purified and identified as levan. To explore the application of this levan in nanotechnology, its silver nanoparticles (AgNPs) were prepared in a simple and green method. Transmission electron microscopy analysis revealed that the size of the synthesised AgNPs was in the range of 19.47 ± 2.45 nm. X-ray powder diffraction analysis confirmed the face-centred cubic crystalline structure of metallic silver. Moreover, the AgNPs at the concentration of 0–12 μg/mL exhibited a significant inhibitive effect on Staphylococcus aureus and Escherichia coli. Tested by tetrazolium-based colourimetric assay, the viability of RAW 264.7 cells was higher than 75% in the presence of 20 μg/mL AgNPs. The results not only proved the feasibility of producing levan from B. amyloliquefaciens with high safety and a high yield, but also demonstrated the simple and cost-efficient approach of AgNPs synthesis as potential antibacterial agents from levan.
Introduction
Several levansucrases from the generally regarded as safe (GRAS) strain of Bacillus amyloliquefaciens have been purified, characterised and heterologously expressed, for use in the biosynthesis of levan [Citation1,Citation2]. Some exopolysaccharides also have been produced from B. amyloliquefaciens [Citation3,Citation4]. However, to the best of our knowledge, there have been few reports on the direct production of levan in a high yield from B. amyloliquefaciens [Citation5,Citation6]. In the past, we optimised the fermentation of an exopolysaccharide from B. amyloliquefaciens PB6 at a high yield of 104 g/L, but the structure of the produced exopolysaccharide was not identified [Citation6].
Levan is a natural homopolysaccharide composed of D-fructo-furanosyl residues linked by β-(2,6) glycosidic linkage as the main chain. In the last few years, levan and its derivatives have attracted significant attention in the biotech and the chemical industry [Citation7,Citation8]. At the same time, silver nanoparticles (AgNPs) have shown great application potential in the research of catalysis, biosensing, drug delivery and medical textiles, due to their unique optical, electrical and biological properties [Citation9–11]. Polysaccharides have been widely used in the synthesis of AgNPs as eco-friendly reducing and capping agents, and therein exopolysaccharides are fascinating in nanocomposites, attributed to their feasibility of large-scale or mass industrial production [Citation12–15]. As for levan, besides antibacterial iron oxide nanoparticles prepared from levan of Bacillus polymyxa PTCC1020 [Citation16], only AgNPs from levan of Acetobacter xylinum with catalytic ability have been reported by Ahmed et al. [Citation17]. Moreover, with the optimised variables from the statistical methods, the highest yield of levan produced from B. polymyxa PTCC1020 and A. xylinum NCIM2526 was only 27 g/L and 13.25 g/L, respectively [Citation18], indicating that levan from the two strains was not suitable for the large-scale industrial production of AgNPs.
In this article, the exopolysaccharide produced from B. amyloliquefaciens PB6 was identified as levan and its AgNPs were further prepared. Moreover, the antimicrobial and cytotoxic effects of the prepared AgNPs were studied.
Materials and methods
Materials
Silver nitrate (AgNO3) and sodium hydroxide (NaOH) were purchased from Sigma Chemical Co. (St. Louis, MO, USA). All other chemicals, containing 3-(4,5-dimethyl-2-thiazolyl)-2,5-diphenyl-2-H-tetrazolium bromide (MTT), were analytical grade reagents and were used without further purification.
The exopolysaccharide-producing B. amyloliquefaciens PB6 is a strain maintained in the National Engineering Laboratory for Industrial Enzymes, Tianjin Institute of Industrial Biotechnology, Chinese Academy of Sciences.
The bacterial strains used in the antimicrobial activity assay, including Escherichia coli (CGMCC 1.90), Staphylococcus aureus (CGMCC 1.89), Salmonella typhimurium (CGMCC 1.1190) and Klebsiella pneumonia (CGMCC 1.10612), were all obtained from the China General Microbiological Culture Collection Center (CGMCC).
RAW 246.7, a murine macrophage cell line was purchased from the Type Culture Collection of the Chinese Academy of Sciences (Shanghai, China).
Methods
Production of exopolysaccharide from B. amyloliquefaciens PB6 by fermentation
According to our reported procedure [Citation6], the fermentation production of the exopolysaccharide from B. amyloliquefaciens PB6 was carried out under the optimal culture medium (400 g/L sucrose, 2.5 g/L yeast extract, 10 g/L sodium chloride) and the optimal fermentation conditions (initial pH 7.0, 4% inoculation amount, 30 °C, 150 r/min) for 26 h. Then the culture medium was centrifuged to remove the bacterial cells, and the exopolysaccharide was harvested from the culture broth by ethanol precipitation, followed by deproteinization with Sevag reagent and dialysis through a membrane with a 10-kDa cutoff. Finally, after freeze drying, the exopolysaccharide was obtained as white powder.
Molecular weight distribution
The molecular weight distribution was determined by gel permeation chromatography on an Agilent 1200 Series HPLC (high performance liquid chromatography) system (Agilent Technologies, Palo Alto, CA, USA) equipped with a refractive index detector and an Agilent PL aquagel-OH MIXED-H column (300 × 7.5 mm, 8 µm), using standard dextrans as references.
Sugar composition and methyl analysis
The sugar composition and methyl analysis were carried out strictly as described by Dong et al. [Citation19].
Fourier transform infrared spectroscopy
The IR spectra (400–4000 cm−1) were recorded by a Bruker Vertex 70 spectrometer (Bruker Optics, Billerica, MA, USA).
Nuclear magnetic resonance (NMR) analysis
1H NMR and 13C NMR spectra were recorded on a Brucker AVANCE III spectrometer (Bruker-Biospin, Rheinstetten, Germany) using D2O as solvent.
Preparation of AgNPs
According to the method of Ahmed et al. [Citation17], 20 mg levan was dissolved in 49 mL of 0.2% sodium hydroxide solution, by subsequent addition of 1 mL silver nitrate aqueous solution (1 mmol/L final concentration). The mixture was allowed to stir at room temperature for 5 min and then heated to 100 °C for 30 min. Then the synthesised AgNPs were dialysed with a 3.5-kDa cutoff membrane for 24 h in darkness and were freeze-dried.
Characterization of AgNPs
The ultraviolet (UV)–vis spectrum was measured using a UV 1800 spectrophotometer (Shimadzu, Tokyo, Japan). X-ray powder diffraction (XRD) measurement was performed at room temperature by a Bruker D8 Advance diffractometer (Bruker Optik GMBH, Karlsruhe, Germany) at an accelerating voltage of 40 kV using CuKα (α = 1.5406A˚) as an X-ray source. The shape, size and dispersity of AgNPs were monitored by transmission electron microscopy (TEM; H-7500, Hitachi, Tokyo, Japan) at an accelerating voltage of 80 kV. Silver content was determined by inductively coupled plasma atomic emission spectroscopy (ICP-AES; Agilent Technologies 700 Series).
Antibacterial assay
The antibacterial activity of AgNPs was studied by the growth curve method with different concentrations of AgNPs [Citation20]. Log phase bacterial cultures in Luria–Bertani (LB) medium with an initial 0.1 absorbance at OD600 were incubated at 37 °C at 200 rpm, after the addition of AgNPs. The bacterial growth was monitored at an interval of 2 h by the absorbance of the culture media at 600 nm (OD600).
Cell viability analysis
Cell viability was measured with tetrazolium-based colourimetric assay in RAW 264.7 cells. Briefly, RAW 264.7 cells (100 μL, 5 × 104 cell/mL) were incubated in a 96-well plate for 24 h at 37 °C and 5% CO2 conditions. Then the cells were washed with fresh Dulbecco’s Modified Eagle Medium (DMEM medium) and treated with AgNPs at final concentrations of 0–20 µg/mL for 24 h. After that, 10 μL of 5 mg/mL MTT was added into each well and the plate was incubated at 37 °C for 4 h. Finally, the medium was replaced with 150 µL of dimethyl sulphoxide to dissolve the formazan crystals, and the absorbance of the solution was measured at 570 nm. DMEM medium with only RAW 264.7 cells served as the control.
Data analysis
Data are expressed as mean values with standard deviation (±SD) from three independent experiments. Statistical comparisons were performed using analysis of variance (ANOVA), and differences at P < 0.05 were considered statistically significant.
Results and discussion
Identification of B. amyloliquefaciens levan
The purified exopolysaccharide from B. amyloliquefaciens PB6 had only one single symmetrical peak on gel permeation chromatography (), indicating its molecular weight homogeneity. The average molecular weight of the exopolysaccharide was estimated as 6.02 kDa. In the sugar composition analysis, the exopolysaccharide was determined to predominantly have fructose (97.7%) with some glucose (2.3%), as seen in . Methyl analysis confirmed the existence of major (2→6)-linked Fruf (90.02%) units and a small amount of (1,2→6)-linked Fruf (2.14%), t-Glcp (2.29%) as well as t-Fruf (5.55%) units in the exopolysaccharide. In Fourier transform infrared spectroscopy (FTIR), the exopolysaccharide showed a broad peak centred at 3400 cm−1 from the O–H stretching, a peak at 2937 cm−1 from the C–H stretching, three peaks at 1128, 1061 and 1019 cm−1 from the glycosidic linkage (C–O–C) stretching and two peaks at 928 and 814 cm−1 representative of the furanoid fructose (). The results from 1 H NMR and 13C NMR are shown in , respectively. In the 13C NMR spectrum, the chemical shift at 104.2, 103.8 and 103.6 ppm indicating β-type glycosidic linkages corresponded to the fructosyl anomeric carbon in (2→6), (1,2→6) linkage and at the terminus, respectively, whereas the chemical shifts at 80.3, 76.3, 75.2, 63.3 and 60.1 ppm were representative of C-5, C-3, C-4, C-6 and C-1 of the fructosyl unit, respectively.
Figure 1. Characterisation of levan by gel permeation chromatography (a) and monosaccharides analysis by HPLC (b), 1H NMR (c) and 13C NMR (d).
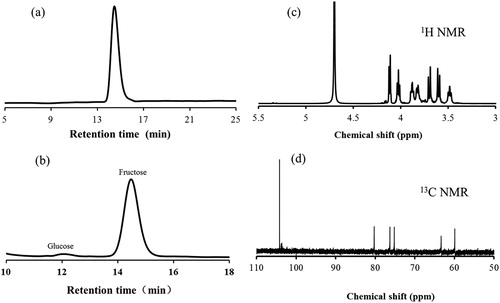
Consistent with other reports on microbial levans [Citation21,Citation22], the above analysis suggested that the exopolysaccharide from B. amyloliquefaciens PB6 existed as a levan structure having β-(2,6) linked fructofuranosyl backbone and some β-(1,2→6) linked fructofuranosyl branches with a D-fructosyl or D-glucosyl residue at the end of the main chain. Gu et al. [Citation23] reported that, through metabolic optimisation of regulatory elements, the levan production in the recombinant B. amyloliquefaciens strain could be improved to 102 g/L, which was close to the reported highest levan production level in the literature. Considering that B. amyloliquefaciens PB6 is a non-engineered strain and its exopolysaccharide yield was 104 g/L, the confirmation of levan structure in this study implied that levan could be produced from B. amyloliquefaciens PB6 with high safety and a high yield.
Characterisation of AgNPs
Attributed to the excitation of surface plasmon resonance (SPR) in the metal nanoparticles, the formation of AgNPs from levan was preliminarily detected by the colour change of the reaction solution from colourless to yellow-brown [Citation24]. A typical SPR band of AgNPs around 409 nm was also observed in the UV–vis spectrum (). According to Yang et al. [Citation25], the SPR band at 409 nm indicated that the size of the AgNPs was approximately between 4 and 20 nm. The TEM image showed that the AgNPs from levan were almost spherical with an average diameter of 19.47 ± 2.45 nm (). Though the size was larger than that reported by Ahmed et al. [Citation17], our AgNPs from levan had a smaller diameter than the AgNPs synthesised from exopolysaccharides of Leuconostoc lactis KC117496, Streptomyces violaceus MM72 and Cs-Hk fungal cultures [Citation26–28]. It was speculated the molecular dimension of these exopolysaccharides might be one cause of the different size of their AgNPs. In the XRD pattern analysis, five main characteristic peaks at 2θ values of 38°, 44°, 64°, 77° and 81° corresponding to 111, 200, 220, 311 and 222 planes for silver (), confirmed the face-centred cubic silver in the AgNPs from levan [Citation29]. In the FTIR spectrum of AgNPs (), all peaks of levan were still observed and the stretching vibration absorption peak of O–H at 3400 cm−1 in levan was shifted to a lower wavenumber round 3320 cm−1, suggesting interactions between the hydroxyl groups of the levan and the AgNPs [Citation30].
Antibacterial and cytotoxic activity of AgNPs
The results from the antibacterial test are shown in . AgNPs exhibited a dose-dependent growth inhibition activity against both Gram-positive and Gram-negative bacteria, including K. pneumoniae and S. typhimurium. The minimum inhibitory concentration (MIC) value of the AgNPs for E. coli was determined to be 12.8 µg/mL, and its best antimicrobial activity was detected in S. aureus: the growth of this strain was almost inhibited in 10 h with the AgNPs concentration at or higher than 9.6 µg/mL. Our results had some differences from the report of Sivasankar et al. [Citation25], in which exopolysaccharide mediated AgNPs synthesised by S. violaceus MM72 showed MIC values of 6.15 and 14.6 μg/mL against E.coli and S. aureus, respectively. However, the high sensitivity of S. aureus to AgNPs was not only observed by us, but also reported in the study of AgNPs prepared from exopolysaccharide of Cordyceps militaris Hk fungal cultures [Citation26]. The reason for this observation was unclear and needs more investigation. Despite of its potent antibacterial activity and wide biological applications, the use of AgNPs as a therapeutic agent is limited due to their potential cytotoxic activity against mammalian cells [Citation31]. Thus, the effect of AgNPs on the viability of macrophage RAW 264.7 cells was also tested by tetrazolium-based colourimetric assay. The result showed that the cell inhibition was below 25% at the presence of 20 μg/mL AgNPs (). Freire et al. [Citation32] thought that colloidal chitosan-silver nanoparticle-fluoride nanocomposites were non-toxic at a concentration of 50 mg/mL, since in their presence, the RAW 264.7 cell inhibition was below 50%. According to their report, our result indicated that AgNPs from levan are able to display antibacterial activity without being harmful to macrophages.
Figure 4. Antibacterial activity of AgNPs from levan against Escherichia coli (a), Staphylococcus aureus (b), Klebsiella pneumonia (c) and Salmonella typhimurium (d).
Note: Data are expressed as mean values; error bars represent ± SD (n = 3).
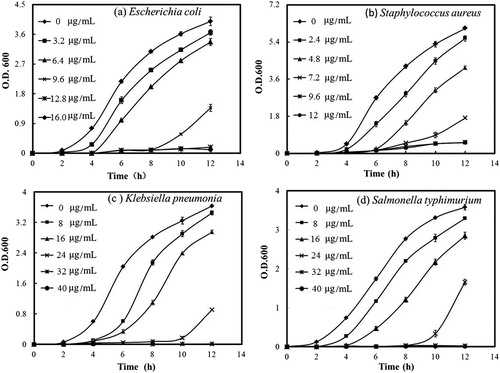
Figure 5. Viability of RAW 264.7 cells in the presence of AgNPs from levan.
Note: Silver ion in the form of AgNO3 was used as the negative control. Data are expressed as mean values; error bars represent ± SD (n = 3). Asterisk indicates statistically significant difference between untreated cells and synthesised AgNPs (or AgNO3) treated cells (*P < 0.05).
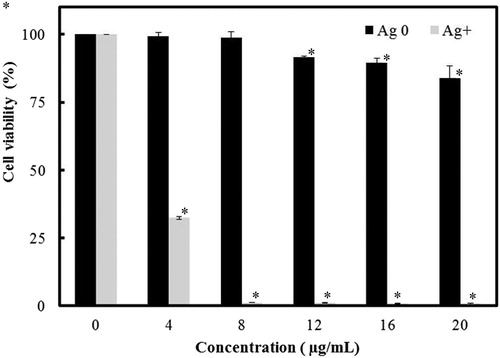
Conclusions
The identification of the low-molecular weight levan confirmed the direct fermentation production of levan from B. amyloliquefaciens at a high yield, providing a safe and efficient industrial production process for levan. Moreover, AgNPs could be prepared from this levan and showed good antibacterial activity with low cytotoxicity, indicating the application potential of levan-based AgNPs in biomedical and nanotechnology industries.
Disclosure statement
No potential conflict of interest was reported by the authors.
Additional information
Funding
References
- Tian F, Inthanavong L, Karboune S. Purification and characterization of levansucrases from Bacillus amyloliquefaciens in intra- and extracellular forms useful for the synthesis of levan and fructooligosaccharides. Biosci Biotech Biochem. 2013;42:1929–1938.
- Rairakhwada D, Seo JW, Seo MY, et al. Gene cloning, characterization, and heterologous expression of levansucrase from Bacillus amyloliquefaciens. J Ind Microbio Bio. 2010;37:195–204.
- Han Y, Liu E, Liu L, et al. Rheological, emulsifying and thermostability properties of two exopolysaccharides produced by Bacillus amyloliquefaciens LPL061. Carbohydr Polym. 2015;115:230–237.
- Yang H, Deng J, Yuan Y, et al. Two novel exopolysaccharides from Bacillus amyloliquefaciens C-1: antioxidation and effect on oxidative stress. Curr. Microbiol. 2015;70:298–306.
- Feng J, Gu Y, Quan, Y, et al. Recruiting a new strategy to improve levan production in bacillus amyloliquefaciens. Sci Rep. 2015;5:13814 [12 p.]. DOI: 10.1038/srep13814
- Zhang LJ, Zeng Y, Zhang Y, et al. Optimization of fermentation conditions for production of exopolysaccharide by Bacillus amyloliquefaciens PB6. Sci Technol Food Ind. 2015;9:214–219.
- Srikanth R, Reddy CH, Siddartha G, et al. Review on production, characterization and applications of microbial levan. Carbohydr Polym. 2015;120:102–114.
- Öner ET, Hernández L, Combie J. Review of levan polysaccharide: from a century of past experiences to future prospects. Biotechnol Adv. 2016;34:827–844.
- Begum T, Gogoi A, Gogoi PK, et al. Catalysis by mont K-10 supported silver nanoparticles: a rapid and green protocol for the efficient ipso-hydroxylation of arylboronic acids. Tetrahedron Lett. 2015;56:95–97.
- Zhang S, Huang N, Lu Q, et al. A double signal electrochemical human immunoglobulin G immunosensor based on gold nanoparticles-polydopamine functionalized reduced graphene oxide as a sensor platform and AgNPs/carbon nanocomposite as signal probe and catalytic substrate. Biosens Bioelectron. 2016;77:1078–1085.
- Jia Z, Xiu P, Li M, et al. Bioinspired anchoring AgNPs onto micro-nanoporous TiO2 orthopedic coatings: trap-killing of bacteria, surface-regulated osteoblast functions and host responses. Biomaterials. 2016;75:203–222.
- Wei L, Lu J, Xu H, et al. Silver nanoparticles: synthesis, properties, and therapeutic applications. Drug Discov Today. 2015;20:595–601.
- Emam, HE, Ahmed HB. Polysaccharides templates for assembly of nanosilver. Carbohydr Polym. 2016;135:300–307.
- Liu Q, Duan B, Xu X, et al. Progress in rigid polysaccharide-based nanocomposites with therapeutic functions. J Mater Chem B. 2017;5:5690–5713.
- Ma Y, Liu C, Qu D, et al. Antibacterial evaluation of sliver nanoparticles synthesized by polysaccharides from Astragalus membranaceus roots. Biomed Pharmacother. 2017;89:351–357.
- Sathiyanarayanan G, Dineshkumar K, Yang YH. Microbial exopolysaccharide-mediated synthesis and stabilization of metal nanoparticles. Crit Rev Microbiol. 2017;43:731–752.
- Taran M, Etemadi S, Safaei M. Microbial levan biopolymer production and its use for the synthesis of an antibacterial iron (II, III) oxide–levan nanocomposite. J Appl Polym Sci. 2017;134:44613. [5 p.] DOI: 10.1002/APP.44613
- Ahmed KBA, Kalla D, Uppuluri KB, et al. Green synthesis of silver and gold nanoparticles employing levan, a biopolymer from Acetobacter xylinum NCIM 2526, as a reducing agentand capping agent. Carbohyd Polym. 2014;112:539–545.
- Srikanth R Siddartha G, Reddy CHSS, et al. Antioxidant and anti-inflammatory levan produced from Acetobacter xylinum NCIM2526 and its statistical optimization. Carbohyd Polym. 2015;123:8–16.
- Dong CX, Zhang LJ, Xu R, et al. Structural characterization and immunostimulating activity of a levan-type fructan from Curcuma kwangsiensis. Int J Biol Macromol. 2015;77:99–104.
- Venkapurwar V, Pokhakar V. Green synthesis of silver nanoparticles using marine polysaccharide: study of in-vitro antibacterial activity. Mater Lett. 2011;65:999–1002.
- Dahech I, Fakhfakh J, Damak M, et al. Structural determination and NMR characterization of a bacterial exopolysaccharide. Int J Biol Macromol. 2013;59:417–422.
- Shih IL, Chen LD, Wang TC, et al. Tandem production of levan and ethanol by microbial fermentation. Green Chem. 2010;12:1242–1247.
- Gu Y, Zheng J, Feng J, et al. Improvement of levan production in bacillus amyloliquefaciens through metabolic optimization of regulatory elements. Appl Microbiol Biot. 2017;101:4163–4174.
- Pallavicini P, Arciola C R, Bertoglio F, et al. Silver nanoparticles synthesized and coated with pectin: An ideal compromise for anti-bacterial and anti-biofilm action combined with wound-healing properties. J Colloid Interf Sci. 2017;498:271–281.
- Yang G, Xie J, Hong F, et al. Antimicrobial activity of silver nanoparticle impregnated bacterial cellulose membrane: Effect of fermentation carbon sources of bacterial cellulose. Carbohyd Polym. 2012;87:839–845.
- Saravanan C, Rajesh R, Kaviarasan T, et al. Synthesis of silver nanoparticles using bacterial exopolysaccharide and its application for degradation of azo-dyes. Biotechnol Rep. 2017;15:33–40.
- Sivasankar P, Seedevi P, Poongodi S, et al. Characterization, antimicrobial and antioxidant property of exopolysaccharide mediated silver nanoparticles synthesized by Streptomyces violaceus MM 72. Carbohyd Polym. 2018;181:752–759.
- Chen X, Yan JK, Wu JY. Characterization and antibacterial activity of silver nanoparticles prepared with a fungal exopolysaccharide in water. Food Hydrocolloid. 2016;53:69–74.
- Jin C, Sun Q. Stress sensitive electricity based on Ag/cellulose nanofiber aerogel for self-reporting. Carbohyd. Polym. 2017;168:265–273.
- Lorestani F, Shahnavaz Z, Mn P, et al. One-step hydrothermal green synthesis of silver nanoparticle-carbon nanotube reduced-graphene oxide composite and its application as hydrogen peroxide sensor. Sensor Actuat B Chem. 2015;208:389–398.
- Gopinath V, Priyadarshini S, Loke MF, et al. Biogenic synthesis, characterization of antibacterial silver nanoparticles and its cell cytotoxicity. Arab J Chem. 2017;10:1107–1117.
- Freire PLL, Albuquerque AJR, Farias IAP, et al. Antimicrobial and cytotoxicity evaluation of colloidal chitosan–silver nanoparticles–fluoride nanocomposites. Int J Biol Macromol. 2016,93:896–903.