Abstract
The long period of seed dormancy is one of the most important reasons leading to the low fertility of dove tree (Davidia involucrata Baill., Davidia hereinafter). During the fruit development process of Davidia, the endocarps will become particularly compact and hard, which was considered as a critical reason for the long seed dormancy of Davidia. However, the biological significance and regulatory mechanism of this process remain unclear. In this study, we identified a CCoAOMT gene, a key member of the lignin biosynthesis pathway, from Davidia endocarp. The gene named DiCCoAOMT1 has an ORF (open reading frame) of 744 bp and encodes a predicted protein of 239 amino acids. The expression of DiCCoAOMT1 is endocarp-specific and the expression levels increased along with the fruit development process. The encoding protein of DiCCoAOMT1 demonstrated a relatively high O-methyltransferase activity when using caffeic acid as a substrate in vitro. The tissue-specific CCoAOMT gene identified in this study might play a key role in specifically regulating the rapid lignification process in Davidia endocarp.
Introduction
Dove tree (Davidia involucrata Baill., Davidia hereinafter) is a monotypic species of Cornales [Citation1]. It is a relic species of Paleocene and now endemic to China [Citation2]. Davidia is known as the ‘living fossil’, which has a unique position in the phylogeny/evolution history of angiosperm plants [Citation3]. Davidia has a particularly low fecundity under natural conditions, largely due to the long dormancy and the low germination rate of its seeds. A lot of researches have been focused on the mechanism of seed dormancy in Davidia and there are two explanations. One proposed that the inhibitors in the seeds and fruits of Davidia are the main determinants. It was reported that the content of endogenous abscisic acid (ABA) and gibberellic acid (GA) in Davidia seeds has a strong correlation with the length of seed dormancy [Citation4]. Several germination inhibitors, including 5-fluorouracil (5-Fu), ABA, cycloheximide (CH) and acid phosphatase were also found in both the endosperm and the sarcocarp of Davidia fruit [Citation5]. Another explanation assumed that the long seed dormancy of Davidia is caused by its compact and hard endocarps, which encapsulate the seeds into a poorly permeable and weakly breathable condition [Citation6]. Our previous study confirmed that the endosperm-removed embryo of Davidia can directly germinate without dormancy, while embryo with endosperm cannot, indicating that the seed dormancy of Davidia is influenced by both the endocarp structure and the internal inhibitors [Citation7].
During the early developmental stage of Davidia fruit in July every year, the structure of endocarps will change from delicate to compact and hard in about 20 days. Lignin is rapidly accumulated in the endocarps during this process. To identify some key genes involved in this process, we performed transcriptome sequencing and Digital Gene Expression Profiling (DGE) analysis in different time points of the early developmental stage of Davidia endocarps (unpublished data). Among the Differentially Expressed Genes (DEGs), the members of the caffeoyl-CoA 3-O-methyltransferase (CCoAOMT) gene family, the key members of the lignin biosynthesis pathway, were dramatically up- or down-regulated along with the lignification process of endocarps. Therefore, the members of the CCoAOMT gene family were selected as target genes in this study.
The CCoAOMT gene was firstly found in the parsley-suspended cells [Citation8] and was subsequently verified to participate in the biosynthesis of lignin and play a role in plant defense responses [Citation9]. CCoAOMT transfers a methyl group from S-adenosyl-L-methionine (SAM) to caffeoyl-CoA, and catalyses CoA and 5-hydroxyacetic acid CoA to produce ferulic acid-CoA and erucanoyl-CoA [Citation10,Citation11]. CCoAOMT genes have been isolated and characterized from various plants such as Arabidopsis [Citation12], cotton (Gossypium spp.) [Citation13] and switchgrass (Panicum virgatum) [Citation14]. CCoAOMT is one of the key enzymes involved in the biosynthesis of lignin monomers. It plays a vital role in G lignin synthesis and provides a substrate for the synthesis of S lignin [Citation15]. Down-regulation or inhibition of the expression of the CCoAOMT gene in tobacco (Nicotiana tabacum), poplar (Populus trichocarpa) and alfalfa (Medicago sativa) resulted in a significant decrease in G-lignin but not S-lignin content [Citation16–18]. Down-regulating the expression of the CCoAOMT gene by RNAi resulted in a significant decrease in lignin content, an increase in cellulose content and a significantly higher S/G lignin ratio in maize (Zea mays) and salvia (Salvia japonica) [Citation19,Citation20]. Besides lignin biosynthesis, the CCoAOMT gene also plays a role in abiotic stress resistance [Citation21]. The CCoAOMT gene was reported to be involved in the response to drought and cold in various plants, such as longan (Dimocarpus longan Lour.) [Citation22] and salvia (S. japonica) [Citation14]. In addition, two CCoAOMT genes were strongly induced by mechanical injury, and the amount of lignin and lignin-like substances in the injured areas was heavily deposited, indicating the underlying relationship between stress response and lignin accumulation regulated by CCoAOMT [Citation23].
In this study, we analyzed the expression profiles of the CCoAOMT gene family in Davidia and selected a CCoAOMT gene with the most significant changes during endocarp development for cloning and characterization. The expression pattern analysis and the enzyme activity assay together indicated that the identified CCoAOMT gene plays a key role in the rapid lignification of Davidia endocarp in a tissue-specific manner.
Materials and methods
Plant materials and standards
The fruits, leaves, pistils, ovaries and bracts of Davidia were collected from the Badagong Mountain National Nature Reserve in Sangzhi County, Zhangjiajie City, Hunan Province (110°5’30”E, 28°46’60”N, 1383 m altitude). The fruits were harvested from 30 June 2016 to 30 September 2016. The fruits were immediately dissected and the endocarps were sampled. Leaves, pistils, ovaries and bracts were collected from late April to late May, 2016. Samples were quickly frozen in liquid nitrogen and stored at –70 °C.
Standards of caffeic acid and EGCG (epigallocatechin gallate) were purchased from Beijing Solarbio Science & Technology Co., Ltd.
Total RNA extraction and cDNA synthesis
Samples (about 100 mg each) were rapidly ground into powder in liquid nitrogen. Total RNA was extracted using the E.Z.N.ATM Plant RNA Kit (Omega Bio-Tek, Norcross, GA). The integrity of the extracted total RNA was detected by 1% agarose gel electrophoresis, and the purity of the total RNA was detected by a UV spectrophotometer (Eppendorf, Hauppauge, NY). Subsequently, cDNA was reverse transcribed using the Prime ScriptTM 1st Strand cDNA Synthesis Kit (Takara, Tokyo, Japan).
Cloning of target gene and sequence analysis
The coding sequence (CDS) of the target gene was obtained from the transcriptome database of Davidia endocarp (unpublished). The DNA fragment of the target gene was amplified by polymerase chain reaction (PCR) using the primer pair DiCCOAOMT1-F/DiCCOAOMT1-R (primer sequences are shown in ). BamHI and XhoI digestion sites were added at the ends of the primers, respectively. The PCR amplification procedure was as follows: 94 °C 5 min; 94 °C 45 s, 55 °C 45 s, 72 °C 1 min, 35 cycles. PCR products were analyzed in 1% agarose gel and purified using the EasyPure Quick Gel Extraction Kit (TransGen Biotech, Beijing, China). Purified DNA fragments were inserted into a pMD18-T vector (Takara, Tokyo, Japan) and transferred into Escherichia coli DH5α (maintained at our laboratory). The single positive colony was sent to be sequenced by Hunan Tsingke Biotechnology Co., Ltd. Sequence alignment and phylogenetic analysis were performed using BLAST algorithm and CLC Sequence Viewer 6.0.
Table 1. Sequences of the primers used in this study.
qPCR analysis
qPCR reaction was performed using 2 × SYBR Green qPCR Master Mix (Biotool, Jupiter, FL) on ABI StepOneTM. Three independent biological replicates of each sample and three technical replicates of each biological replicate were used for qPCR analysis. A Davidia gene, DiUBQ, derived from our previously obtained transcriptome data of Davidia, was used as the reference gene for data normalization [Citation24,Citation25]. The primers used in qPCR are shown in . The relative expression fold of each sample was calculated by its CT value normalized to the CT value of the reference gene using the 2–ΔΔCT method described by Livak and Schmittgen [Citation26].
Prokaryotic expression and purification
The complete CDS of the target gene was constructed into a pET-28a (+) vector through digestion (BamHI and XhoI) and ligation, and the constructed vector was introduced into E. coli strain BL21 (DE3). IPTG (isopropyl β-D-1-thiogalactopyranoside) with a final concentration of 0.1 mmol/L was used to induce the expression of the target protein. After induction, the bacteria were cultured at 37 °C, 200 rpm for 6 h. Samples were collected at 1, 2, 3, 4 and 5 h during the expression process, respectively. Target protein was purified using His Mag Sepharose™ Ni kit (GE Healthcare, Pittsburgh, PA). Finally, 12% SDS-PAGE electrophoresis was used to analyze the expression product and the purified protein.
In vitro enzyme activity assay
The O-methytransferase activity of the target protein was assayed in vitro using SAM510: SAM Methyltransferase Assay (Biosciences, San Diego, CA). Two compounds, caffeic acid and EGCG, were used as substrates, respectively. Assaying was performed on a microplate reader (Thermo, Waltham, MA) and the absorbance at 510 nm of each sample was measured. Methyltransferase activity was calculated using the equation:
Methyltransferase Activity (μmol H2O2/min/mg) = (ΔAbs/min)/15.0 mM−1 × (0.115 mL/0.005 mL)/m [Citation27]
Three independent biological replicates of each sample and three technical replicates of each biological replicate were performed. The concentration of purified protein was assayed by the Bradford method [Citation28].
Lignin staining
Davidia endocarps were crosscut, and treated by 25% HCL for 2 min, then stained by 1% phloroglucinol for 2 min for observation.
Statistical analysis
All data were derived from three independent experiments (with internal replicates). The significance of the differences among the means of the data was analyzed using a t-test run in the SAS8.1 program.
Results and discussion
Rapid lignin accumulation in Davidia endocarps
To record the rapid lignification process, Davidia endocarps were collected from four different developmental time points (5 d as an interval) during the early developmental stage of the fruits and were stained for the observation of lignin content. The results demonstrated that the lignin content in the endocarp was dramatically increased at the early developmental stage of Davidia fruit (early in July every year), and reached a relatively high content in about 20 d (). The endocarps were delicate at the beginning of this stage, but became compact and hard after the 20-day lignification process. Then lignin would continually deposit in the endocarps until the fruits and seeds became fully mature and the endocarps became completely lignified (). This observation indicates that the lignification process, as well as the gene regulation involved in it, initiates at the very early stage of the fruit development in Davidia, and the lignin accumulation rate is the fastest in the first 20 days.
Figure 1. Lignin content at different stages of Davidia endocarps: Stage I, 30 June 2016 (a); Stage II, 10 July 2016 (b); Stage III, 15 July 2016 (c); Stage IV, 20 July 2016 (d); and Stage V, 30 September 2016 (e).
Note: Fruits were immediately crosscut and stained. Red parts represent lignin deposition. Endocarp in (e) was not stained because it was completely lignified.
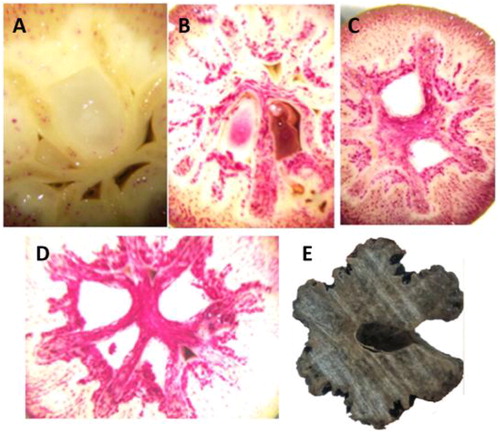
Lignin biosynthesis is an essential biological process for structural support and water transport in woody plants [Citation29]. The lignin biosynthesis pathway has been well documented and genes involved in this process have been comprehensively studied by comparative genome analysis [Citation30]. Although the phenylpropanoid and lignin pathways have been well clarified, their regulatory mechanisms remain unclear. Most lignin related researches were focused on the lignin accumulation in xylem. However, lignin is also accumulated in other tissues or organs such as shells and endocarps in many woody plants. Whether the key genes involved in the lignification process in different tissues are similar or different is unknown. The particularly rapid lignification process occurring in Davidia endocarps is an ideal object to investigate the key genes involved in lignin biosynthesis in other tissues rather than the xylem.
Expression profiles of the CCoAOMT gene family in Davidia
The gene expression in endocarp samples at different stages of the lignification process (Stage I–IV, ) were analyzed by transcriptome sequencing (unpublished data). Fourteen CCoAOMT genes, which are key members of the pathway of lignin biosynthesis, were found differentially expressed during the lignification process. Seven of them were up-regulated and others were down-regulated (). Among them, the expression level of transcript Cluster-149.63013 showed strong positive correlation with the lignin content of endocarps. Therefore, Cluster-149.63013 was selected as the target gene for further analysis.
Figure 2. Expression profiles of Davidia CCoAOMT genes during different stages of endocarps.
Note: The codes of transcripts are indicated at the side of each step. Stage I to IV represent the samples of different developmental stages of endocarp, which are consistent with those in . Red frame represents the further investigated gene of the present study.
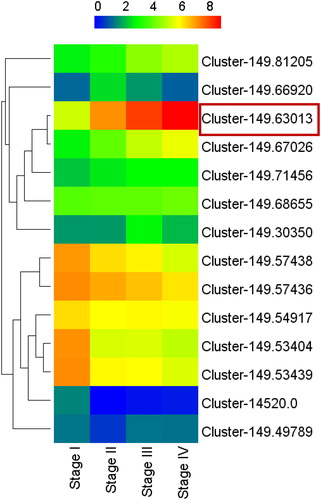
CCoAOMT is one of the key enzymes of the lignin pathway, which plays an essential role in the synthesis of guaiacyl lignin units as well as in the supply of substrates for the synthesis of syringyl lignin units [Citation31]. There are usually a number of members of the CCoAOMT gene family in a plant species; whether their functions are redundant or finely differentiated remains unclear. We have identified 14 CCoAOMT genes from the transcriptome data, while only the target gene DiCCoAOMT1 showed high expression level in the endocarps, indicating the existence of fine division of function among CCoAOMT genes. Our results demonstrated that the expression levels of Davidia CCoAOMT genes in endocarps are relatively low except for DiCCoAOMT1, indicating that DiCCoAOMT1 is critical for the lignin biosynthesis of endocarps.
Cloning and sequence analysis of the DiCCoAOMT1 gene
A 744 bp fragment was amplified by PCR from the cDNA of Davidia endocarp. Its ORF encodes a predicted protein of 247 amino acids, which has 91% homology to a CCoAOMT sequence from Capsicum annuum (NP_001311511.1). The Cluster-149.63013 sequence was named DiCCoAOMT1 (GenBank accession: KY243330), for it is the first identified CCoAOMT gene in Davidia.
Conserved domain analysis showed that the amino acid sequence of DiCCoAOMT1 contains eight conserved motifs (A–H), which are the conserved domain of O-methyltransferase [Citation32] (). It is notable that the amino acid residues H39 (in motif D) and S123 (in motif F) of DiCCoAOMT1 are distinctive. The Y39H mutant changes a neutral amino acid into an alkaline amino acid, and the V/I123S mutant changes a hydrophobic amino acid into a hydrophilic amino acid. These variations in the conserved domains of DiCCoAOMT1 might influence its function. Phylogenetic analysis showed that DiCCoAOMT1 has a close relationship with DcCCoAOMT1 from Daucus carota subsp. sativus and InCCoAOMT5 form Ipomoea nil (). Comparing to CCoAOMT sequences from other species, the deduced amino acid sequence of DiCCoAOMT1 has two distinctive mutant variants, Y39H and V/I123S, which are located in the conserved domain D and F, respectively. These sites might be key sites to determine the activity and specificity of CCoAOMT enzymes.
Figure 3. Sequence analysis (a) and phylogenetic analysis (b) of the predicted protein sequence of DiCCoAOMT1.
Note: (a) Alignment of predicted amino acid sequence of DiCCoAOMT1 and homologous sequences of other species. Capital letters with underline represent conserved motifs of CCoAOMT. (b) Phylogenetic analysis of amino acid sequence of DiCCoAOMT1 and homologous sequences of other species. Black points represent target protein. Cf, Cephalotus follicularis; Dz, Durio zibethinus; Bp, Broussonetia papyrifera; Ep, Eucalyptus pilularis; Vv, Vitis vinifera; Pl, Paeonia lactiflora; Eg, Erythranthe guttata; Pt, Populus tremuloides, and Populus trichocarpa; Pe, Populus euphratica; Mc, Macleaya cordata; Nn, Nelumbo nucifera; At, Amborella trichopoda; Mn, Morus notabilis; Zj, Ziziphus jujuba; In, Ipomoea nil; Dc, Daucus carota subsp. sativus; Nt, Nicotiana tomentosiformis; Na, Nicotiana attenuata; Cc, Capsicum chinense; Cl, Codonopsis lanceolata.
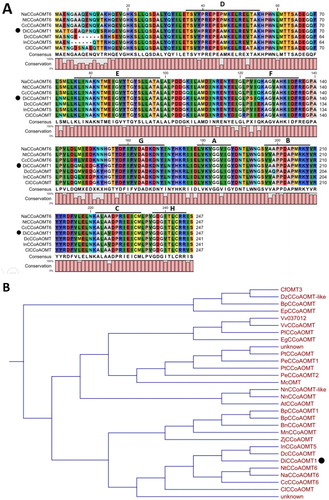
Expression pattern of DiCCoAOMT1
qPCR analysis was used to detect the expression pattern of DiCCoAOMT1. The results showed that, in endocarps, the expression level of DiCCoAOMT1 is significantly higher than those of other members of CCoAOMT gene family (). We furtherly analyzed the tissue-specificity of DiCCoAOMT1. The results showed that DiCCoAOMT1 has significantly higher expression level in endocarps than in other tissues, verifying the expression of DiCCoAOMT1 is dominant in endocarp (). In addition, DiCCoAOMT1 has a relatively higher expression in the large white bract, which is a distinctive organ of Davidia.
Figure 4. Expression patterns of CCoAOMT genes in Davidia. (a) Relative expression levels of Davidia CCoAOMT genes in endocarps. The relative expression levels are presented in relation to the expression levels of DiUBQ transcripts (as 1). (b) Relative expression levels of DiCCoAOMT1 in different tissues.
Note: Values are means ± SD of three biological replicates. Different lower-case letters in a column in the same panel indicate significant difference at p < 0.05.
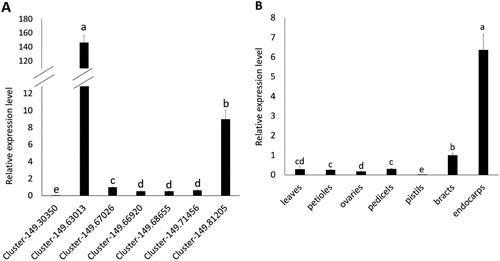
We found that the expression of DiCCoAOMT1 has tissue-specificity and was strictly induced according to the development degree of the endocarp. Interestingly, the expression levels of DiCCoAOMT1 in pedicels, ovaries and pistils, which are closely related to endocarps, were quite low, indicating that the spatiotemporal expression of DiCCoAOMT1 was under strict regulation. The subtle expression of DiCCoAOMT1 is supposed to be governed by certain endocarp-specific and/or development-related CIS (cis-acting) elements existing in its promoter, which needs further studies. The expression pattern of DiCCoAOMT1 is supposed to be another important factor to realize the rapid lignification just in Davidia endocarps.
Enzyme activity of DiCCoAOMT1 in vitro
The CDS of the DiCCoAOMT1 gene was introduced into a pET-28a (+) vector for prokaryotic expression. An approximately 29.0-kDa product was obtained in E. coli by IPTG induction (). Then the protein was purified for the enzyme activity assay ().
Figure 5. Prokaryotic expression (a) and purification (b) of DiCCoAOMT1 protein.
Note: (a) Prokaryotic expression of DiCCoAOMT1 protein. Lane M, Marker; Lane Citation1–6, IPTG induction for 0–5 h. (b) Purification of DiCCoAOMT1 protein. Lane M, Marker; Lane Citation1, supernatant before purification; Lane Citation2, primary purification products; Lane Citation3, secondary purification products.
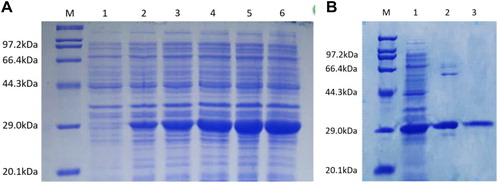
Caffeic acid was used as a substrate instead of caffeoyl-CoA to measure the catalytic activity of DiCCoAOMT1. The optimum substrate concentration of the enzymatic reaction was assayed and 0.2 mmol/L caffeic acid was used for assaying the enzyme activity (). The enzymatic reaction results are shown in . According to the reaction curve, the enzyme activity of DiCCoAOMT1 was calculated to be 0.0703 (μmol H2O2/min/mg protein). It was reported that EGCG is another substrate of CCoAOMT [Citation33], therefore the catalytic activity of DiCCoAOMT1 using EGCG as a substrate was assayed. The optimum substrate concentration of the enzymatic reaction was assayed and 20 mmol/L EGCG was used for assaying the enzyme activity (). The results showed that the catalytic activity of DiCCoAOMT1 using EGCG as a substrate is 0.0053 (μmol H2O2/min/mg protein) (), which is 0.07-fold of the enzyme activity when using caffeic acid as a substrate although the concentration of EGCG is 100-fold that of caffeic acid, indicating DiCCoAOMT1 has a relatively stringent substrate specificity.
Figure 6. O-methyltransferase catalytic activity of DiCCoAOMT1 in vitro. Caffeic acid and EGCG was used as substrate to assay the enzyme activity of DiCCoAOMT1. (a) Reaction systems with different concentration of caffeic acid catalyzed by DiCCoAOMT1 (After 200 min of reaction). (b) Profile of absorbance at 510 nm during the catalytic reaction process; mean values ± SD of three replicates. (c) Reaction systems with different concentration of EGCG catalysed by DiCCoAOMT1 (after 200 min of reaction). (d) Profile of absorbance at 510 nm during the catalytic reaction process. Mean values ± SD of three replicates.
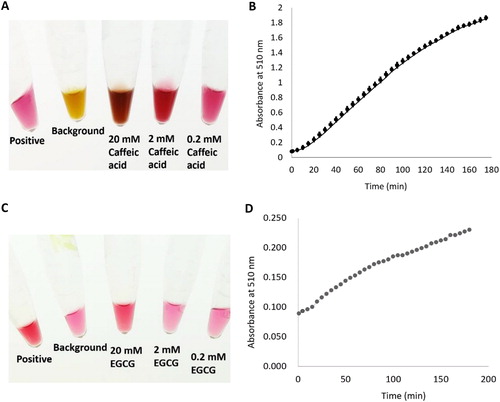
Comparing with the process of lignin accumulation in the xylem of woody plants, the lignification processes in a few fruits, such as the shell of walnut and Macadamia nut, are much faster due to the limited period of fruit development [Citation34]. To form these highly lignified structures, such as the structure of Davidia endocarp, in a relatively short time, lignification-related enzymes with higher activity are supposed to be involved. We purified the encoding product of DiCCoAOMT1, and confirmed that its O-methyltransferase activity is higher than the reported CCoAOMT enzymes, for example, CCoAOMT from N. tabacum and Camellia sinensis [Citation18,Citation33]. CCoAOMT from C. sinensis was reported to catalyze the formation of three monomethylated EGCG compounds (EGCG4"Me, EGCG3"Me and EGCG3'Me), indicating other functions of CCoAOMT [Citation33]. However, DiCCoAOMT1 showed very low activity when using EGCG as its substrate, suggesting stringent specificity of DiCCoAOMT1.
Conclusions
In this study, we identified a CCOAOMT gene from Davidia, which has a dominant expression in endocarp and has an increasing expression pattern along with the lignification process of the endocarps. The identified DiCCoAOMT1 gene and its function provide new insights into the regulatory mechanism and biological significance of rapid lignification in Davidia endocarps. On the other hand, the high O-methyltransferase activity of DiCCoAOMT1 showed the potential to accelerate the process of lignin biosynthesis, which will be valuable for the genetic improvement of timber tree species.
Abbreviations | ||
Davidia | = | Davidia involucrata Baill |
CCoAOMT | = | caffeoyl-CoA O-methyltransferase |
CDS | = | coding sequence |
ORF | = | open reading frame |
ABA | = | abscisic acid |
GA | = | gibberellic acid |
5-Fu | = | 5-fluorouracil |
CH | = | cycloheximide |
DGE | = | digital gene expression profiling |
DEG | = | differentially expressed gene |
SAM | = | S-adenosyl-L-methionine |
EGCG | = | epigallocatechin gallate |
CIS | = | cis-acting element |
Acknowledgements
We thank Li Jian for photograph and picture processing. We also thank Li Mei, Huang Fengzhen and Gao Runxin for valuable comments on the draft and language editing.
Disclosure statement
No potential conflict of interest was reported by the authors.
Funding
This research was supported by grants from the Excellent Youth Program in Hunan Education Department under grant number 17B282, the National Keypoint Researh and Invention Program under grant number 2016YFD0600102 and Youth Scientific Research Fund of CSUFT under grant number QJ201512.
References
- Cronquist A. An integrated system of classification of flowering plants. Columbia University Press; 1981.
- Spongberg, SA. Exploration and introduction of ornamental and landscape plants from eastern Asia. In: Janick J, Simon JE, editors. New crops. New York (NY): Wiley; 1993. p. 140–147.
- Manchester SR. Leaves and fruits of Davidia (Cornales) from the Paleocene of North America. Syst Bot. 2002;27(2):368–382.
- Lei N, Su Z, Chen J, et al. [Germination inhibitors in fruit of rare and endangered Davidia involucrate]. China J Appl Environ Biol. 2003;9(6):607–610. Chinese.
- Li Z, Chen R, Fu J. [Study on isophosphatases of dove tree (Davidia involucrata Baill.) seeds during dormancy and germination]. J Southwest Forest College. 1989;9:1. Chinese.
- Wan C. [Anatomical study on seed dormancy of dove tree (Davidia involucrata)]. J Central South Forest College. 1988;8:1. Chinese.
- Wu J, Li M, Cao F, et al. [Study on rapid propagation technology of Davidia involucrata seed]. J Central South Univ Forest Tech. 2016;36:2. Chinese.
- Pakusch AE, Kneusel RE, Matern U. S-adenosyl-L-methionine: trans-caffeoyl-coenzyme A 3-O-methyltransferase from elicitor-treated parsley cell suspension cultures. Arch Biochem Bioph. 1989;271(2):488–494.
- Schmitt D, Pakusch AE, Matern U. Molecular cloning, induction and taxonomic distribution of caffeoyl-CoA 3-O-methyltransferase, an enzyme involved in disease resistance. J Biol Chem. 1991;266(26):17416–17423.
- Wagner A, Tobimatsu Y, Phillips L, et al. CCoAOMT suppression modifies lignin composition in Pinus radiata. Plant J. 2011;67(1):119–129.
- Shen H, Mazarei M, Hisano H, et al. A genomics approach to deciphering lignin biosynthesis in switchgrass. Plant Cell. 2013;25(11):4342–4361.
- Do CT, Pollet B, Thévenin J, et al. Both caffeoyl coenzyme A 3-O-methyltransferase 1 and caffeic acid O-methyltransferase 1 are involved in redundant functions for lignin, flavonoids and sinapoyl malate biosynthesis in Arabidopsis. Planta. 2007;226(5):1117–1129.
- Ni Z, Lv M, Ma W, et al. Cloning and expression analysis of CCoAOMT gene from Gossypium hirsutum L. Acta Botanica Boreali-Occidentalia Sinica. 2009;29(10):1946–1953.
- Liu S, Huang Y, He C, et al. Cloning, bioinformatics and transcriptional analysis of caffeoyl-coenzyme A 3-O-methyltransferase in switchgrass under abiotic stress. J Integr Agr. 2016;15(3):636–649.
- Boerjan W, Ralph J, Baucher M. Lignin biosynthesis. Annu Rev Plant Biol. 2003;54:519–546.
- Zhong R, Morrison WH, Himmelsbach DS, et al. Essential role of caffeoyl coenzyme A O-methyltransferase in lignin biosynthesis in woody poplar plants. Plant Physiol. 2000;124(2):563–578.
- Guo D, Chen F, Inoue K, et al. Downregulation of caffeic acid 3-O-methyltransferase and caffeoyl CoA 3-O-methyltransferase in transgenic alfalfa. impacts on lignin structure and implications for the biosynthesis of G and S lignin. Plant Cell. 2001;13(1):73–88.
- Pinçon G, Maury S, Hoffmann L, et al. Repression of O-methyltransferase genes in transgenic tobacco affects lignin synthesis and plant growth. Phytochemistry. 2001;57(7):1167–1176.
- Li X, Chen W, Zhao Y, et al. Downregulation of caffeoyl-CoA O-methyltransferase (CCoAOMT) by RNA interference leads to reduced lignin production in maize straw. Genet Mol Biol. 2013;36(4):540–546.
- Wang Z, Ge Q, Chen C, et al. Function analysis of caffeoyl-COA O-methyltransferase for biosynthesis of lignin and phenolic acid in Salvia miltiorrhiza. Appl. Biochem. Biotechnol. 2017;181(2):562–572.
- Baxter HL, Stewart Jr CN. Effects of altered lignin biosynthesis on phenylpropanoid metabolism and plant stress. Biofuels. 2013;4(6):635–650.
- Chen H, He X, Luo C, et al. Molecular cloning of longan caffeoyl-CoA O-methyltransferase (DlCCoAOMT) and its expression analysis. Scientia Agr Sinica. 2012;45(1):118–126.
- Chen C, Meyermans H, Burggraeve B, et al. Cell-specific and conditional expression of caffeoyl-coenzyme A-3-O-methyltransferase in poplar. Plant Physiol. 2000;123(3):853–868.
- Li M, Dong X, Peng J, et al. De novo transcriptome sequencing and gene expression analysis reveal potential mechanisms of seed abortion in dove tree (Davidia involucrata Baill.). BMC Plant Biol. 2016;16(1):82. DOI: 10.1186/s12870-016-0772-x
- Ren R, Dai P, Li M, et al. [Selection and stability evaluation of reference genes for real-time quantitative PCR in dove tree (Davidia involucrata)]. Plant Physiol J. 2016;52(10):1565–1575. Chinese.
- Livak KJ, Schmittgen TD. Analysis of relative gene expression data using real-time quantitative PCR and the 2(-Delta Delta C(T)) Method. Methods. 2001;25:402–408.
- Kumar A, Saigal K, Malhotra K, et al. Structural and functional characterization of Rv2966c protein reveals an RsmD-like methyltransferase from Mycobacterium tuberculosis and the role of its N-terminal domain in target recognition. J Biol Chem. 2011;286:19652–19661.
- Bradford MM. A rapid and sensitive method for the quantitation of microgram quantities of protein utilizing the principle of protein-dye binding. Anal Biochem. 1976;72:248–254.
- Whetten R, Sederoff R. Lignin biosynthesis. Plant Cell. 1995;7:1001–1013.
- Xu Z, Zhang D, Hu J, et al. Comparative genome analysis of lignin biosynthesis gene families across the plant kingdom. BMC Bioinformatics. 2009;10(Suppl 11):S3. DOI: 10.1186/1471-2105-10-S11-S3
- Ye Z, Zhong R, Morrison III WH, et al. Caffeoyl coenzyme A O-methyltransferase and lignin biosynthesis. Phytochemistry. 2001;57:1177–1185.
- Joshi CP, Chiang VL. Conserved sequence motifs in plant S-adenosyl-L-methionine-dependent methyltransferases. Plant Mol Biol. 1998;37(4):663–674.
- Zhang Y, Lv H, Ma C, et al. Cloning of a caffeoyl-coenzyme A O-methyltransferase from Camellia sinensis and analysis of its catalytic activity. J Zhejiang Univ Sci B. 2015;16(2):103–112.
- Sinner M, Puls J, Dietrichs H. Carbohydrate composition of nut shells and some other agricultural residues. Starch–Stärke. 1979;31(8):267–269.