Abstract
Avain influenza (AI) virus H5N1 poses a serious threat to public health. Yamanaka factors (Oct4, Sox2, Klf4, c-Myc) are usually transcription factors involved in proliferation, apoptosis, differentiation, immunity and somatic cell reprogramming. However, the expression status of Yamanaka factors related to influenza virus infection has not yet been described. Here our results showed that SOX2, MYC and KLF4 were significantly overexpressed in HEK293T cells as compared to A549 cells. The H5N1 virus infection experiment showed that OCT4 and KLF4 were up-regulated and MYC was down-regulated during high titer virus infection in A549 cells. In HEK293T cells, OCT4, SOX2 and KLF4 were up-regulated and MYC was down-regulated by H5N1 infection. Overexpression of NP and PB2 of H5N1 virus induced up-regulation of OCT4 in A549 cells. In addition, KF4 was increased only by overexpression of NP protein in A549 cells. However, KLF4 was down-regulated by overexpression of PB2 protein in HEK293T cells. Yamanaka factors were not found to be significantly affected by NS1 and M1 in A549 and HEK293T cells. HA or NA did not affect the expression of Yamanaka factors in HEK293T cells. In conclusion, the expression patterns of Yamanaka factors during H5N1 infection could be a novel regulatory mechanism involved in the pathogenesis of influenza viruses.
Introduction
Avian influenza A virus H5N1 is one of the greatest worldwide pandemic threats to poultry and human health. H5N1 influenza virus contains eight negative-sense, single-stranded RNAs that code for 11 proteins (HA, NA, NP, M1, M2, NS1, NEP, PA, PB1, PB1-F2 and PB2) [Citation1,Citation2]. The structural and non-structural proteins of the virus are relevant in respect of the adaptive and innate immune responses through activation or inhibition of a series of immune-related cytokines. Cytokines, being innate antiviral cell-intrinsic restriction factors, prevent viruses from traversing the lipid bilayer and accessing the cytoplasm. Examples of such viruses are influenza A virus (IAV), dengue virus (DENV), Japanese encephalitis virus (JEV), human immunodeficiency virus (HIV), Rift Valley fever virus (RVFV), Ebola virus (EBOV), etc. [Citation3–9]. Currently, research shows that pluripotent stem cells (iPSC) induced by Yamanaka transcription factors (Oct4, Sox2, Klf4, c-Myc) can develop from different terminally differentiated cells. Krüppel-like factor 4 (KLF4) is an evolutionarily conserved zinc finger-containing transcription factor implicated in many biological processes, such as proliferation, differentiation, apoptosis and development [Citation10–12]. A recent study showed that SOX2-positive airway progenitor cells induce generation of KRT5+ cells that repopulate damaged alveolar parenchyma following influenza virus infection [Citation13]. Oct4+ stem/progenitor swine lung epithelial cells are targets for influenza virus replication [Citation14]. Influenza A infection significantly increases the glutamine consumption rate of cultured primary cells; glutaminase inhibition decreases the replication of influenza A in cultured primary cells. In addition, adenovirus-induced MYC activation promotes increased glutamine uptake and increased use of glutamine, thus raising the question whether MYC also plays a role in influenza virus replication [Citation15]. However, to date, there is little information whether Yamanaka transcription factors are involved in influenza virus infection. Thus, in the present study, we first found Yamanaka factors produce a novel transcription pattern following H5N1 virus infection.
Materials and methods
Cell culture
A549 and HEK 293T cells were cultured in high-glucose Dulbecco’s modified Eagle’s medium (DMEM, Gibco) with 10% fetal bovine serum (FBS, Gibco), in humidified 5% CO2 and 95% air at 37 °C.
Virus manipulation
Mouse adapted influenza virus A/environment/Qinghai/1/2008 (H5N1) and sterile phosphate buffered saline (PBS) was injected into 10-day-old embryonated chicken eggs at 37 °C for 2 days. Allantoic fluid was collected for the experimental group and the mock group, respectively. Allantoic fluid was purified by centrifugation and stored at −80 °C until use. Viral infection was induced when cells were 80% confluent. The serum-free DMEM medium containing allantoic fluid was added at 0.5, 1, 2 multiplicities of infection (MOI) and the samples were incubated for 1 h. Then cells were washed thrice with PBS and cultured in DMEM containing 2% FBS. All experiments with this infectious virus were carried out in a Biosafety Level 3 containment laboratory.
Gene cloning and plasmids
The open reading frame of influenza virus NS1, M1, NP, PB2, HA and NA genes were amplified by polymerase chain reaction (PCR) and cloned into pcDNA3.1/myc-His(+)A vector for gene overexpression experiments. All constructs were verified by sequencing, and the primers used in this study are listed in .
Table 1. Primer sequences of full-length gene annealing temperature used in construct plasmids.
Transfection
Cells were seeded in 12-well plates overnight and transfected with 2 μg plasmids suspended in 3 μL Lipofectamine 3000 (Invitrogen) and 100 μL OPTI-MEM Reduced Serum Medium. After 6 h transfection, the cell medium was replaced by fresh DMEM medium with 10% FBS. HEK 293T and A549 cells were transfected for 36 h, then harvested and resolved in TRIzol Reagent for RNA extraction and reverse transcription.
RNA extraction and cDNA synthesis
Total RNA was extracted from A549 and HEK 293T cells using the TRIzol Reagent according to the supplier’s specifications. A spectrophotometer (Thermo) was used to determine the concentration of RNA at 260/280 nm. cDNA was synthesized using 5 μg of total RNA from A549 and HEK 293 T cells. GoScriptTM Reverse Transcription System (Promega) was used for first-stand synthesis following the manufacturer’s instructions. The samples were stored at −80 °C until used.
PCR and real-time PCR
Real-time PCR was performed in an ABI 7500 fast real-time system (Life Technology) with SYBR green real-time PCR master mix (CWBIO). Real-time PCR conditions were as follows: 95 °C for 10 min, then 94 °C for 30 s, 60 °C for 1 min, 40 cycles, followed by 72 °C for 7 min. Reactions were performed in a 20 μL volume containing 10 μL SYBR green real-time PCR master mix, 8.5 μL double-distilled water, 1 μL forward and reverse primers, and 0.5 μL cDNA template. The primers are listed in [Citation16–19]. We used β-actin as an internal reference in order to normalize the mRNA expression levels. The generation of specific PCR products was confirmed by melting curve analysis. The 2−ΔΔCT method was used to quantify the relative mRNA expression levels. All PCR products were confirmed in 1.5% agarose gels with ethidium bromide staining.
Table 2. Primer sequences, amplicon size and annealing temperature used in real-time PCR assays.
Statistical analysis
All data were expressed as the means ± S.E.M. (standard error of the mean). Student’s t-test was used to determine the significance of differences between two groups, whereas one-way analysis of variance (ANOVA) was used when there were more than two groups. P-values of less than 0.05 were considered to be statistically significant.
Results and discussion
H5N1 virus promoted the overexpression of OCT4 and KLF4 in A549 cells
A549 and HEK293T cells developed obvious lesions with the increase of H5N1 influenza virus titer (). First, we determined the background expression levels of Yamanaka factors; our results showed that SOX2, MYC and KLF4 were significantly overexpressed in HEK293T cells as compared to A549 cells (). H5N1 virus experimental infection showed that OCT4 and KLF4 were up-regulated and MYC was down-regulated with high titer virus infection in A549 cells (). OCT4, SOX2 and KLF4 were up-regulated and MYC was down-regulated in HEK293T cells (). A549 and HEK293T cells have been used as model cells for influenza virus research in a large number of scientific experiments. H7N9 nucleoprotein was transfected into human HEK293T cells, followed by immunoprecipitation for proteomic analysis [Citation20]. Influenza pseudotype particles are obtained by co-transfecting human embryonic kidney HEK293T cells using plasmids coding for the influenza HA, HIV gag-pol and a lentiviral vector incorporating firefly luciferase [Citation21]. Quantitative phosphoproteomic analysis of A549 cells infected with 2009 pandemic influenza virus A (H1N1) was done to identify potential drug targets [Citation22]. The A549 cell line was used to study the relationship of some apoptosis-associated molecules with novel pandemic influenza A (H1N1) virus, A/California/04/2009 [Citation23]. The antiviral effect in interferon (IFN)-stimulated A549 cells was most apparent after infection with avian influenza virus [Citation24]. The expression levels of viral proteins and their nucleocytoplasmic localization were not affected upon hStau1 silencing, but virus particle production, as determined by purification of virions from supernatants, was reduced in Human HEK293T cells or A549 cells [Citation25]. Although HEK293T and A549 cells have been involved in many influenza virus experiments, our results suggested that the background level of Yamanaka factors may cause transcription sensitivity to H5N1 virus infection.
Figure 1. A549 cells (A) and HEK293T (B) cells infected with H5N1 influenza virus.
Note: Overnight cell cultures seeded on 12-well plates were inoculated with 0.5 MOI, 1 MOI and 2 MOI H5N1 virus and incubated for 36 h. The mock group was inoculated with sterile allantoic fluid.
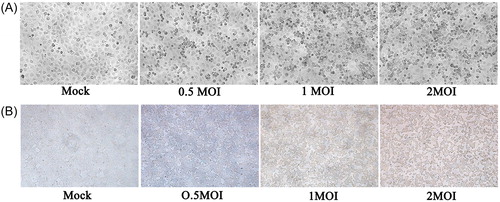
Figure 2. Expression of Yamanaka factors in A549 cells and HEK293T cells.
Note: Quantitative data (mean ± SEM) from at least three independent experiments were normalized to β-actin. *p < 0.05; **p < 0.01; ***p < 0.001.
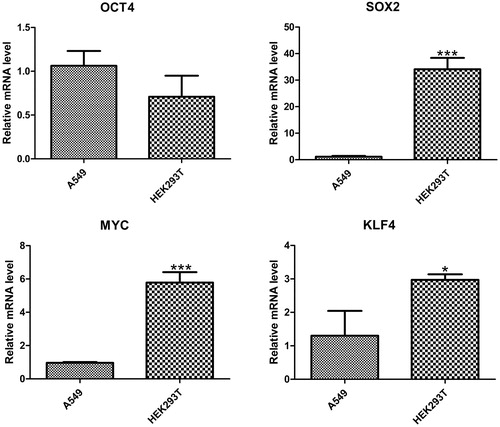
Figure 3. Gene expression of Yamanaka factors in A549 cells (A) and HEK293T cells (B) with H5N1 virus infection.
Note: Overnight cell cultures seeded 12-well plates were inoculated with 0.5 MOI, 1 MOI and 2MOI H5N1 virus and incubated for 36 h. Quantitative data (mean ± SEM) from at least three independent experiments were normalized to β-actin. *p < 0.05; **p < 0.01; ***p < 0.001. The mock group was inoculated with sterile allantoic fluid.
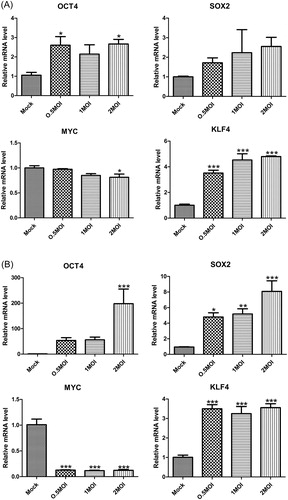
OCT4 expression was significantly increased by H5N1 virus proteins NP and PB2
The above results revealed that H5N1 influenza virus could significantly affect the transcription of Yamanaka factors both in A549 cells and in HEK293T cells. Next, we tried to reveal which H5N1 virus proteins are further influenced by the activities of Yamanaka factors. Our study showed that overexpression of NP and PB2 of H5N1 virus induced up-regulation of OCT4 in A549 cells. In addition, KF4 was increased only by overexpression of NP protein in A549 cells (). However, KLF4 was down-regulated by overexpression of PB2 protein in HEK293T cells (). Yamanaka factors are transcription factors that are usually highly expressed in embryonic stem (ES) cells; their up-regulation can promote pluripotency in both human and mouse somatic cells. Yu et al. [Citation26] isolated primary LECs (lens epithelial cells) from postnatal 3–5-day old SD rats and transfected by Sendai virus loaded with four factors, OCT3/4, Sox2, c-Myc and Klf4, which could induce LEC-iPSC-like cells appearance. Both NP and PB2 as effectors of influenza virus participate in cellular biochemical reactions. For example, NP possibly has a proapoptotic role in influenza virus pathogenesis, and PB2 protein of H5N1 influenza virus isolated from a human increased the growth ability of an avian H5N1 virus in human lung epithelial A549 cells [Citation27,Citation28].
Figure 4. Effects of influenza virus proteins NP and PB2 on the gene expression of Yamanaka factors in A549 cells (A) and HEK293T cells (B). Quantitative data (mean ± SEM) from at least three independent experiments were normalized to β-actin. *p < 0.05; **p < 0.01. The mock group was inoculated with sterile allantoic fluid.
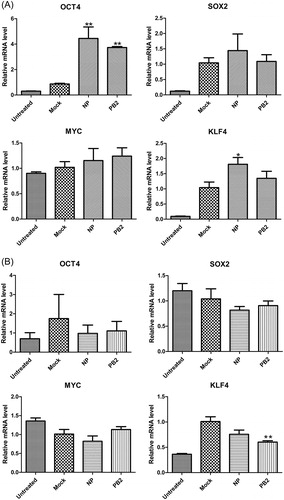
NS1 and M1 did not affect Yamanaka factors
The results obtained using NS1 and M1 plasmids constructed in this study showed that neither NS1 nor M1 had an effect on the expression of Yamanaka factors either in A549 cells or in HEK293T cells (). As a non-structural protein, NS1 dramatically inhibits cellular gene expression and prevents the activation of key players in the IFN system [Citation29]. NS1 of H7N9 virus increased the protein levels of total p53, p53 phosphorylated at Ser46 and Ser37, activated caspase 9, and the Bax/Bcl-2 ratio in A549 cells [Citation30]. Expression of NS1 of A/Beijing (H1N1) and A/Indonesia (H5N1) significantly induced excessive phosphorylation of ERK and elevated cell viability [Citation31]. The matrix protein M1 plays a pivotal role in the budding of influenza virus from the plasma membrane of infected cells [Citation32]. Previous research showed that amino acid residue changes within the M1 protein of H5N1 virus are important determinants for pathogenicity in mice [Citation33].
HA and NA did not affect Yamanaka factors in HEK293T cells
Influenza type A viruses are categorized into subtypes based on the type of both HA and NA on the surface of the viral envelope. To investigate whether HA and NA were associated with the transcriptional regulation of Yamanaka factors, HA and NA plasmids were constructed in this study. The results showed that neither HA nor NA affected the expression of Yamanaka factors in HEK293T cells (). HA and NA were used as the main immune antigens for influenza vaccines and are ineffective if the glycoprotein of the vaccine strains is a mismatch with that of the epidemic strain [Citation34]. In another report, HEK293T cell-produced HA displayed a very narrow receptor specificity, but insect cell-produced HA demonstrated decreased receptor specificity [Citation35].
Figure 6. Effects of influenza virus proteins HA and NA on the gene expression of Yamanaka factors in HEK293T cells.
Note: Statistically non-significant differences following over-expression of HA and NA of influenza virus in HEK293T cells.
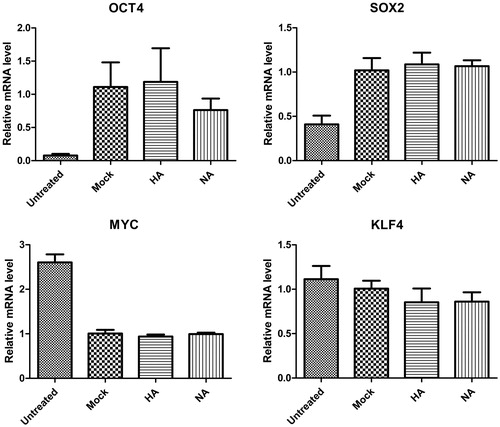
The terminal stage of H5N1 influenza and other viral diseases is often the onset of a cytokine storm, the massive overproduction of cytokines by the body's immune system [Citation36–38]. Usually the production of cytokines requires different types of transcription factors. In view of the outstanding performance of Yamanaka factors in stem cell induction, we considered whether there is a relationship between Yamanaka factors and influenza virus infection. Our results showed that different cell lines may be the cause for the stimulation of individual viral proteins to induce expression of different Yamanaka factors. Meanwhile, PB2 and NP have a positive influence on the transcription of Yamanaka factors. In addition to promoting the formation of pluripotent stem cells, Yamanaka factors are also implicated in cancerogenesis and the mechanism of some virus infections. For example, alpha fetoprotein is closely related to hepatocarcinogenesis; therefore Oct4, Klf4, Sox2 and c-myc expression was positively associated with hepatocellular carcinoma tissues prior to involvement in HBV-related hepatocarcinogenesis [Citation39]. OCT4 is critically associated with the pluripotency and self-renewal of ES cells; however, hepatitis C virus core protein can upregulate OCT4 expression and promote cell cycle progression in the mechanism of hepatocarcinogenesis by HCV infection [Citation40]. System biology demonstrated MYC-associated zinc finger (MAZ) transcription factor may be involved in the regulation of viral RNA sensing, immune cell signalling and cell cycle arrest in infected lungs [Citation41]. In addition, plasmablastic lymphoma in both HIV-positive and HIV-negative patients has been associated with Epstein-Barr virus infection and MYC gene aberrations [Citation42]. In glioblastoma, the most lethal brain tumor, ubiquitination of c-MYC was antagonized through deubiquitinase USP13, thus further maintaining the glioblastoma stem cells activities [Citation43]. Reportedly, post-transcriptional regulation of KLF4 by high-risk human papillomaviruses is critical for the differentiation-dependent viral life cycle [Citation44]. Another previous study showed that H5N1 virus promotes the expression of KLF4 and KLF5 in both A549 and HEK293T cells, but overexpression of KLF5 increases the transcription of IFITM1, IFITM2 and IFITM3 in A549 cells, not in HEK293T cells [Citation45].
Conclusions
Our results provide evidence that virions and viral proteins of H5N1 influenza virus display different regulatory mechanisms for the Yamanaka factors. Virions had a more pronounced effect than viral proteins, NP and PB2 can induce up-regulation of OCT4, therefore KLF4 was increased only by NP protein in A549 cells. In addition, the expression of Yamanaka factors was determined in different cell types with H5N1 influenza virus infection, and H5N1 virus proteins performed different roles in the transcriptional regulation of Yamanaka factors. Our present study is still in its early stages and more studies are needed to clarify the potential molecular mechanisms.
Disclosure statement
The authors declare no conflict of interests.
Additional information
Funding
References
- Resa-Infante P, Jorba N, Coloma R, et al. The influenza virus RNA synthesis machine: advances in its structure and function. RNA Biol. 2011;8:207–215.
- Rossman JS, Lamb RA. Influenza virus assembly and budding. Virology. 2011;411:229–236.
- Friedman RL, Manly SP, McMahon M, et al. Transcriptional and posttranscriptional regulation of interferon-induced gene expression in human cells. Cell. 1984;38:745–755.
- Reid LE, Brasnett AH, Gilbert CS, et al. A single DNA response element can confer inducibility by both alpha- and gamma-interferons. Proc Natl Acad Sci USA. 1989;86:840–844.
- Diamond MS, Farzan M. The broad-spectrum antiviral functions of IFIT and IFITM proteins. Nat Rev Immunol. 2013;13:46–57.
- Bailey CC, Zhong G, Huang IC, et al. IFITM-family proteins: the Cell's First Line of Antiviral Defense. Annu Rev Virol. 2014;1:261–283.
- Huang IC, Bailey CC, Weyer JL, et al. Distinct patterns of IFITM-mediated restriction of filoviruses, SARS coronavirus, and influenza A virus. PLoS Pathog. 2011;7:e1001258. DOI: 10.1371/journal.ppat.1001258
- John SP, Chin CR, Perreira JM, et al. The CD225 domain of IFITM3 is required for both IFITM protein association and inhibition of influenza A virus and dengue virus replication. J Virol. 2013;87:7837–7852.
- Brass AL, Huang IC, Benita Y, et al. The IFITM proteins mediate cellular resistance to influenza A H1N1 virus, West Nile virus, and dengue virus. Cell. 2009;139:1243–1254.
- Ghaleb AM, Nandan MO, Chanchevalap S, et al. Krüppel-like factors 4 and 5: the yin and yang regulators of cellular proliferation. Cell Res. 2005;15:92–96.
- Dang DT, Pevsner J, Yang VW. The biology of the mammalian Krüppel-like family of transcription factors. Int J Biochem Cell Biol. 2000;32:1103–1121.
- Liu C, DeRoo EP, Stecyk C, et al. Impaired autophagy in mouse embryonic fibroblasts null for Krüppel-like factor 4 promotes DNA damage and increases apoptosis upon serum starvation. Mol Cancer. 2015;14:101. DOI: 10.1186/s12943-015-0373-6
- Ray S, Chiba N, Yao C, et al. Rare SOX2(+) airway progenitor cells generate KRT5(+) cells that repopulate damaged alveolar parenchyma following influenza virus infection. Stem Cell Reports. 2016;7:817–825.
- Khatri M, Goyal SM, Saif YM. Oct4+ stem/progenitor swine lung epithelial cells are targets for influenza virus replication. J Virol. 2012;86:6427–6433.
- Thai M, Thaker SK, Feng J, et al. MYC-induced reprogramming of glutamine catabolism supports optimal virus replication. Nat Commun. 2015;6:8873. DOI: 10.1038/ncomms9873
- Bahl K, Saraya A, Sharma R. Increased levels of circulating and tissue mRNAs of Oct-4, Sox-2, Bmi-1 and Nanog is ESCC patients: potential tool for minimally invasive cancer diagnosis. Biomarker Insights. 2012;7:27–37.
- Duangmano S, Dakeng S, Jiratchariyakul W, et al. Antiproliferative effects of cucurbitacin B in breast cancer cells: down-regulation of the c-Myc/hTERT/telomerase pathway and obstruction of the cell cycle. Int J Mol Sci. 2010;11:5323–5338.
- Sun XP, Dong X, Lin L, et al. Up-regulation of survivin by AKT and hypoxia-inducible factor 1α contributes to cisplatin resistance in gastric cancer. FEBS J. 2014;281:115–128.
- Lubecka-Pietruszewska K, Kaufman-Szymczyk A, Stefanska B, et al. Folic acid enforces DNA methylation-mediated transcriptional silencing of PTEN, APC and RARbeta2 tumour suppressor genes in breast cancer. Biochem Biophys Res Commun. 2013;430:623–628.
- Sun N, Sun W, Li S, et al. Proteomics analysis of cellular proteins co-immunoprecipitated with nucleoprotein of influenza A virus (H7N9). Int J Mol Sci. 2015;16:25982–25998.
- Ferrara F, Molesti E, Böttcher-Friebertshäuser E, et al. The human transmembrane protease serine 2 is necessary for the production of group 2 influenza A virus pseudotypes. J Mol Genet Med. 2013;7:309–314.
- Dapat C, Saito R, Suzuki H, et al. Quantitative phosphoproteomic analysis of host responses in human lung epithelial (A549) cells during influenza virus infection. Virus Res. 2014;179:53–63.
- Wang X, Tan J, Zoueva O, et al. Novel pandemic influenza A (H1N1) virus infection modulates apoptotic pathways that impact its replication in A549 cells. Microbes Infect. 2014;16:178–186.
- Škorvanová L, Švančarová P, Svetlíková D, et al. Protective efficacy of IFN-ω and IFN-λs against influenza viruses in induced A549 cells. Acta Virol. 2015;59:413–417.
- de Lucas S, Peredo J, Marión RM, et al. Human staufen1 protein interacts with influenza virus ribonucleoproteins and is required for efficient virus multiplication. J Virol. 2010;84:7603–7612.
- Yu Y, Jiang H, Li H, et al. Alpha-a-crystallin protects lens epithelial cell-derived iPSC-Like cells against apoptosis induced by oxidative stress. Cell Reprogram. 2016;18:327–332.
- Yamaji R, Yamada S, Le MQ, et al. Identification of PB2 mutations responsible for the efficient replication of H5N1 influenza viruses in human lung epithelial cells. J Virol. 2015;89:3947–3956.
- Mayank AK, Sharma S, Nailwal H, et al. Nucleoprotein of influenza A virus negatively impacts antiapoptotic protein API5 to enhance E2F1-dependent apoptosis and virus replication. Cell Death Dis. 2015;6:e2018. DOI: 10.1038/cddis.2015.360
- Marc D. Influenza virus non-structural protein NS1: interferon antagonism and beyond. J Gen Virol. 2014;95:2594–2611.
- Yan Y, Du Y, Wang G, et al. The novel H7N9 influenza A virus NS1 induces p53-mediated apoptosis of A549 cells. Cell Physiol Biochem. 2016;38:1447–1458.
- Miyazaki M, Nishihara H, Hasegawa H, et al. NS1-binding protein abrogates the elevation of cell viability by the influenza A virus NS1 protein in association with CRKL. Biochem Biophys Res Commun. 2013;441:953–957.
- Hilsch M, Goldenbogen B, Sieben C, et al. Influenza a matrix protein M1 multimerizes upon binding to lipid membranes. Biophys J. 2014;107:912–923.
- Fan S, Deng G, Song J, et al. Two amino acid residues in the matrix protein M1 contribute to the virulence difference of H5N1 avian influenza viruses in mice. Virology. 2009;384:28–32.
- Wang W, Li R, Deng Y, et al. Protective efficacy of the conserved NP, PB1, and M1proteins asimmunogens in DNA- and vaccinia virus-based universal influenza A virus vaccines in mice. Clin Vaccine Immunol. 2015;22:618–630.
- de Vries RP, de Vries E, Bosch BJ, et al. The influenza A virus hemagglutinin glycosylation state affects receptor-binding specificity. Virology. 2010;403:17–25.
- Sordillo PP, Helson L. Curcumin suppression of cytokine release and cytokine storm. A potential therapy for patients with Ebola and other severe viral infections. In Vivo. 2015;29:1–4.
- Kalaiyarasu S, Kumar M, Senthil Kumar D, et al. Highly pathogenic avian influenza H5N1 virus induces cytokine dysregulation with suppressed maturation of chicken monocyte-derived dendritic cells. Microbiol Immunol. 2016;60:687–693.
- Phung TTB, Sugamata R, Uno K, et al. Key role of regulated upon activation normal T-cell expressed and secreted, nonstructural protein1 and myeloperoxidase in cytokine storm induced by influenza virus PR-8 (A/H1N1) infection in A549 bronchial epithelial cells. Microbiol Immunol. 2011;55:874–884.
- Zhu M, Li W, Lu Y, et al. HBx drives alpha fetoprotein expression to promote initiation of liver cancer stem cells through activating PI3K/AKT signal pathway. Int J Cancer. 2017;140:1346–1355. DOI: 10.1002/ijc.30553
- Zhou JJ, Meng Z, Zhou Y, et al. Hepatitis C virus core protein regulates OCT4 expression and promotes cell cycle progression in hepatocellular carcinoma. Oncol Rep. 2016;36:582–588.
- Shoemaker JE, Fukuyama S, Eisfeld AJ, et al. Integrated network analysis reveals a novel role for the cell cycle in 2009 pandemic influenza virus-induced inflammation in macaque lungs. BMC Syst Biol. 2012;6:117. DOI: 10.1186/1752-0509-6-117
- Olofson AM, Loo EY, Hill PA, et al. Plasmablastic lymphoma mimicking carcinomatosis: a case report and review of the literature. Diagn Cytopathol. 2017;45:243–246.
- Fang X, Zhou W, Wu Q, et al. Deubiquitinase USP13 maintains glioblastoma stem cells by antagonizing FBXL14-mediated Myc ubiquitination. J Exp Med. 2017;214:245–267.
- Gunasekharan VK, Li Y, Andrade J, et al. Post-transcriptional regulation of KLF4 by high-risk human papillomaviruses is necessary for the differentiation-dependent viral life cycle. PLoS Pathog. 2016;12:e1005747. DOI: 10.1371/journal.ppat.1005747
- Wang HF, Chen L, Luo J, et al. KLF5 is involved in regulation of IFITM1, 2, and 3 genes during H5N1 virus infection in A549 cells. Cell Mol Biol (Noisy-le-Grand). 2016;62:65–70. DOI: 10.14715/cmb/2016.62.13.12