Abstract
We have recently identified several aroylhydrazone-based molecular hybrids with antiseizure activity. We aimed to investigate the analgesic activity of these molecules further. Male ICR mice were injected intraperitoneally with the tested substances or with the vehicle only (controls). We performed hot plate tests, formalin test and maximal electroshock (MES) test and further studied the cytokine proteome in the brain. In silico analysis was performed for prediction of the physicochemical parameters and activity. The furan-substituted aroylhydrazone-based 2H-chromene hybrid showed a significant increase of the latent time as compared with the baseline values (p < .05). Both the chlorine-substituted and the methyl-substituted aroylhydrazone-based coumarin derivatives significantly decreased the first phase of the formalin test (p < .05). We also found suppression of all the cytokines which were overexpressed in the model of acute seizures (MES test) and in the formalin paw test. The in silico model predicted hydroxymethylglutaryl coenzyme A synthetase 2 (HMGCS2) enhancer activity. Further testing is needed to confirm the mechanism of the potentially beneficial effects of the tested substances and to evaluate the toxicity and efficacy of the proposed molecules.
Introduction
Epilepsy is a brain disorder characterized by recurrent unprovoked seizures as a result of abnormal electrical discharges. It afflicts between 0.5% and 1% of the population [Citation1]. The distribution of people with epilepsy across the globe is unequal, 85% of them live in low- and middle-income countries, where 49% of the world population is concentrated [Citation2], suggesting that perhaps some environmental factors, such as poverty and poor hygiene, interacting with immunity could explain at least in part this prevalence distribution. The rate of comorbidity, both psychiatric and non-psychiatric, in patients with epilepsy is very high [Citation3]. Several diseases including depression, anxiety, dementia, migraine, heart disease, peptic ulcers and arthritis are up to eight times more prevalent in adults with epilepsy than in the general population [Citation4]. Data for comorbidities in children with epilepsy also indicate an increased risk of almost all types of medical, neurologic, developmental and psychiatric disorders [Citation5]. The comorbidities seriously impair the quality of life and worsen the prognosis of epilepsy [Citation6]. Recently, an epilepsy-specific risk adjustment comorbidity index was developed for mortality outcomes research [Citation7].
Estimations show that at least one in five of the adult population suffers from pain and that another one in 10 adults is diagnosed with chronic pain each year [Citation8]. Unfortunately, the available analgesic drugs exert a wide range of side effects, and most are either too potent or too weak. Central hyper-excitability is a hallmark of both epilepsy and chronic pain conditions (neuropathic pain [Citation9], fibromyalgia [Citation10] and migraine [Citation11]). Chronic pain due to inflammatory diseases, that is new joint pain, and other types of pain, including neck, facial and low back pain, is more common in adults with epilepsy than in the general population. Pain disorder comorbidities are 1.36 up to 1.96 times higher in people with epilepsy, as compared with those without epilepsy [Citation12]. The rate of ictal non-epileptic headache ranges from 0.5 to 5%, and that of postictal headache ranges from 12 to 52% [Citation13]. Increased pain sensitivity during the interictal period [Citation14] and postictal analgesia [Citation15] has been reported. Ictal/postictal analgesia was proposed as a contributing factor for increased traumatism in patients with epilepsy [Citation16].
It is a well-accepted approach to evaluate the neuropharmacological spectrum of activities, including putative analgesic properties, of substances with already proved antiseizure activity. A lot of broad-spectrum antiepileptic drugs (AEDs) show efficacy against abnormal neuronal activity in bipolar disorders, migraine and neuropathic pain. Carbamazepine, gabapentin and pregabalin are currently the only three AEDs approved by the United States’ Food and Drug Administration (FDA) and the European Medicines Agency (EMA) for the treatment of neuropathic pain, but many others are presently used off-label. A recently published overview of Cochrane reviews [Citation17] concluded that there are satisfactory evidence-based efficacy data for neuropathic pain and fibromyalgia treatment only for gabapentinoids. Gabapentinoids bind with the α2δ subunit of L-type voltage-gated calcium channels and enhance non-vesicular GABA release, which modulates the peripheral and central trigeminovascular dural nociceptive neurons. However, the gabapentinoids are not useful for prophylaxis of migraine, as they do not target nociceptive neurons in the thalamus [Citation18]. Among AEDs, only valproate and topiramate have analgesic effect in the preventive treatment of migraine [Citation19], suggesting an implication of different pathological processes.
Combining two independently active pharmacophores into one covalently linked hybrid compound is an approach of rational drug design. The advantage of the hybrid compounds lies in the simultaneous modulation of multiple drug targets [Citation20], and both epilepsy and chronic pain can be considered multifactorial. We have previously reported antiseizure activities of several new aroylhydrazone hybrid molecules, containing either coumarin or 2H-chromene scaffolds, using maximal electroshock (MES), pentylenetetrazole (PTZ) and 6-Hz tests in the experimental studies [Citation21]. Aroylhydrazones are a class of azomethines having the –C = N−N − group. These compounds exhibit dynamic reversible isomerization when photochemically and thermally activated, hydrazine substitution and coordination to metallic centres [Citation22]. Their biological activity is widely studied. Coumarins (2H-chromen-2-one) and 2H-chromenes serve as unique pharmacophores in medicinal chemistry arena, due to the key features, such as planar aromatic ring fused with lactone functionality, a readily available group for hydrogen bonding as well as for protein–ligand interaction [Citation23].
Recent research attributed the aetiology of seizures to cytokine disbalance. Pro-inflammatory cytokine production and inflammatory reactions have been evaluated both in experimental models [Citation24] and in clinical trials [Citation25]. Cytokines are produced from glial cells, neurons and T lymphocytes. The role of cytokines in neuronal plasticity and synaptic transmission is extensively evaluated. Brain inflammation is thought to be the common cause of epilepsy comorbidities, such as depression and autism spectrum disorders. Cytokines and chemokines are considered to be at the crossroads of neuroinflammation, neurodegeneration and neuropathic pain [Citation26]. However, their role in the mechanism of the acute pain is rarely studied. Another open question is the overlapping of the cytokine effects in epilepsy and pain disorders.
The aim of our study was (1) to test several aroylhydrazone-based molecular hybrids with antiseizure activity for analgesic action, (2) to evaluate the effect of the tested substances on the cytokine proteome in MES-induced seizures and in the formalin test and (3) to perform in silico search for possible underlying antiseizure and/or analgesic mechanisms of the tested substances to guide our further research.
Materials and methods
Animals
Male ICR mice (25–30 g) obtained from the vivarium of the Medical University of Sofia were randomly distributed in groups of six and placed in standard laboratory conditions (12-h/12-h cycle, with access to water and standard rodent food ad libitum). All experiments were performed after one week of adaptation, between 9.00am and 12.00am. Mice underwent overnight fasting but had free access to water. Each animal was tested only once, 30 min after an intraperitoneal injection of the tested compounds. During the hot plate test, the mice were tested twice, before and after the injection of the tested substance.
The tested substances were provided by the Department of Chemistry of the Pharmaceutical Faculty of the Medical University of Sofia. They were dissolved in the vehicle (10% dimethyl sulphoxide (DMSO) in normal saline) and were injected intraperitoneally, in concentrations equal to the EC50 value determined in the MES test in our preliminary experiments (). The control group was injected with the vehicle only. At the end of the tests, the animals were decapitated, and the brains were removed, flash-frozen in liquid nitrogen and stored at −80°С for further evaluation of the cytokine proteome.
Table 1. Tested compounds ED50 (MES test) and TD50 (rotarod test) were previously evaluated.
Behaviour tests
All behaviour tests were observed by two independent observers who were unaware of the injected substances.
Hotplate test
Acute thermal pain was induced using a temperature of 47 °C. The tested mice were placed in an open-ended cylinder over the hot plate equipped with a thermostat and observed for two types of reaction, that is paw licking and jumping. The latent period for the antinociceptive behaviour of mice was measured (licking of the hind paws or jumping). This test was performed twice: at baseline and 30 min post-injection.
Formalin test
This test in mice is indicative of continuous pain. Subdermal injection (left posterior paw) of 1% 0.02 mL formalin solution was applied 30 min after the mice were injected intraperitoneally with the test compounds or with the vehicle (controls). The time interval was chosen based on the time-to-peak effect (TPE) of the tested substances in the MES test. The animals were observed for 20–40 min to detect a typical two-phase reaction. During the first phase (0–5 min), we recorded the time (in seconds) for licking of the paw. After a latent period, we observed the second phase of antinociceptive behaviour (between the 20th and 30th min) and recorded the time in seconds.
Statistics
Data from the analgesia testing are presented as mean values with standard deviation (±SD). After testing for normality of distribution and equality of variances, data were evaluated with one-way analysis of variance (ANOVA) and Holm–Sidak post hoc tests with the accepted level of significance р < .05.
Maximal electroshock test
We aimed to compare the cytokine release during the formalin test with or without pretreatment with the tested substances and further to examine it with cytokines released during seizures. Tonic–clonic seizures were induced with transcorneal application of short (0.2 s) suprathreshold electrical impulses in mice (50 mA).
Cytokine array analysis
For simultaneous determination of 40 cytokines, the Mouse Cytokine Array Panel A kit (Proteome Profiler Array, R&D Systems) was used. Eight pools were prepared as follows: A. controls; B. MES test group; C. formalin-injected group (1% 0.02 mL in the left posterior paw); D–H. formalin applied 30 min after the intraperitoneal application of substances 8a, 8b, 4a, 4b, 4c, respectively, in dosages as described in .
The whole brain of each mouse was weighed and homogenized with a mechanical homogenizer in a phosphate buffer with protease inhibitor (10 μg/mL aprotinin, 10 μg/mL leupeptin and 10 μg/mL pepstatin) to a final ratio of 0.5:1 (tissue: volume). After the homogenization, Triton X-100 was added to a final concentration of 1%. Each pool was prepared from the brain homogenates from the samples of all mice (n = 6) in each group with a final volume of 6 mL. After gentle stirring, 1 mL was taken from each pool, and after that the manufacturer’s protocol was followed. The membranes were screened with a С-DiGit Blot Scanner (Li-Cor), and the pixel intensity was analyzed with HLImage++ suite (R&D Systems).
Ethics of experimentation
The protocol of the study was approved by the Ethics Committee of the Bulgarian Food Safety Agency and meets the Animals in Research: Reporting In Vivo Experiments (ARRIVE) guidelines.
In silico analysis
Chemicalize suite ChemAxon Ltd. 2018 was used for prediction of the physicochemical parameters. To seek a plausible explanation of the analgesic and/or antiseizure activity of the tested substances, and also to shape our future research in this field, we performed in silico evaluations with PASS suite of Way2Drug. We preselected structure-based predicted activity with p > .7 ().
Table 2. Predicted activities of the tested substances.
Results and discussion
Analgesic activity of aroylhydrazone-based molecular hybrids with antiseizure activity
We assessed the potential analgesic activity of aroylhydrazone-based molecular hybrids for which our earlier evaluations demonstrated antiseizure activity and the rotarod test did not reveal adverse effects on locomotion. Analgesic activity was tested with two different tests: the hot plate test and the formalin test. The hot plate test assesses higher brain functions, and the following response represents a supraspinal coordinated reaction [Citation27]. Both types of response, that is paw licking and jumping, are thought to be supraspinally integrated responses. Paw licking is accepted as more sensitive for analgesic testing, whereas decreased jumping reflects effects on locomotion. Analgesics with anti-inflammatory activity, that is aspirin, paracetamol, ibuprofen, are less effective in this test [Citation28] as compared with more potent ones, that is opioids. presents the latent period (seconds) to the paw licking. The furan-substituted aroylhydrazone-based 2H-chromene hybrid showed statistically significant (p < .05) increase in the latent period as compared to the baseline values of the latent time measured in the same mice.
Figure 1. Hotplate test results. Note: Data are mean values (±SD), n = 6 in each group, *p < .05, statistically significant difference as compared with the baseline value (0 min) of the same group.
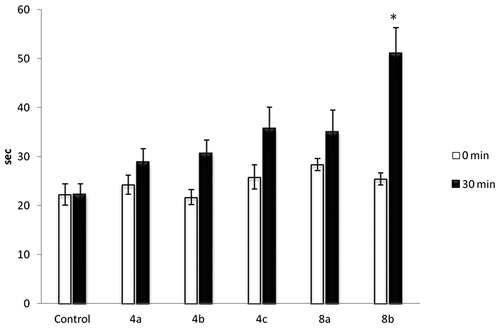
The results from the formalin test revealed that the coumarin derivatives 4a and 4c significantly decreased the first phase of the test (). The biphasic response is thought to be peripherally generated. The interphase is a result of hyperpolarization and transient inactivation by formaldehyde of the surviving neurons; their recovery and the centrifugal spread of formalin in the skin induce the second phase of nociceptive activity before the formalin concentration falls below a threshold.
Brain cytokine overexpression in MES test and formalin paw test: modulation by aroylhydrazone-based molecular hybrids
The analysis of the cytokine production in the control group showed expression of most of the evaluated cytokines. These results are in agreement with the concept that most of the cytokines are produced through constitutive exocytosis, involving trafficking of newly synthesized protein cargos that may or may not be initiated by receptor stimulation of nuclear DNA transcription and RNA translation [Citation29,Citation30]. Cytokines are frequently formed in cascades making their activity redundant, that is similar actions can be triggered by different cytokines (reviewed in [Citation31]). Proteome analysis gave us the opportunity for a global assessment simultaneously of 40 cytokines and to compare their expression immediately after seizure induction, after the formalin injection and the effect of the tested substances.
As we expected, several cytokines were highly expressed after the MES test. These included B lymphocyte chemoattractant (BLC, also known as chemokine (C-X-C motif) ligand 13, or CXCL-13), soluble intracellular adhesion molecule 1 (sICAM-1), interferon gamma-induced protein 10 (IP-10, also known as CXCL10), interferon-inducible T-cell alpha chemoattractant (I-TAC, also known as CXCL11), chemokine regulated on activation, normal T cell expressed and secreted (RANTES, also known as CCL5) among others ().
Figure 3. Proteome cytokine expression. Controls (A), MES test group (B), formalin group (C), 8a (D), 8b (E), 4a (F), 4b (G) 4c (H). Note: Results are expressed in pixel density.
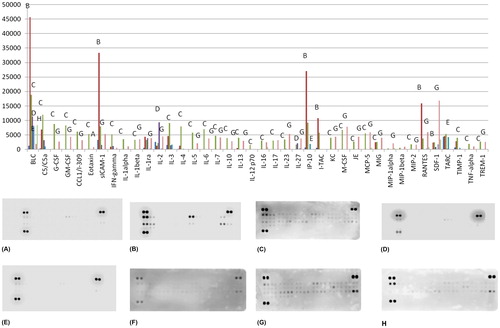
CXCL13 is a small chemokine serving as a chemoattractant for B lymphocytes. A recent study [Citation32] on the expression of some cytokines in experimentally induced seizures showed that the Cxcl13 gene remains upregulated for up to 72 h, suggesting its involvement in the induction and maintenance of the seizure hypersusceptibility. The CXCL13 expression is a hallmark of microglial activation [Citation32]. Interestingly, we succeeded to identify an elevation of CXCL13 after the formalin injection, too. Several investigators revealed the role of CXCL13 also in the astrocyte activation and neuropathic pain [Citation33].
The elevated sICAM-1 levels (which we found in the MES test pool) may be a marker of cerebral blood flow dysregulation due to endothelial damage. A human study revealed that sICAM-1 is elevated both in the serum and in the cerebrospinal fluid of patients with epilepsy, which is why it was proposed as a marker for treatment resistance [Citation34]. A small study evaluated sICAM as a potential biomarker for pain intensity in chronic pain patients [Citation35]. In this study, we found higher expression of sICAM after the formalin test as compared to the controls, but not as much as it was in the MES group.
CXCL10 is an early expressed T-helper 1 (Th1) cytokine. It is also an essential modulator of the synaptic plasticity and neuronal activity in the hippocampus [Citation36]. It is a small protein that mediates immune responses through the activation and recruitment of T cells, eosinophils, monocytes and NK cells [Citation37]. The overexpression of this chemokine has been observed in several neurodegenerative diseases including multiple sclerosis, Parkinson’s disease, HIV-associated dementia and Alzheimer’s disease [Citation26]. CXCL10 induces apoptosis in foetal neurons by elevating intracellular calcium levels. A control function of CXCL10 on the inflammatory pain was described recently [Citation38]. In our study, this chemokine was highly expressed in the formalin test and even higher in the MES test.
CXCL11 was also highly expressed in the MES group. CXCL11 is considered to be a marker of the M1 phenotype of microglial activation [Citation39]. Classically activated (M1) microglia can produce reactive oxygen species (respiratory burst) and increased production of proinflammatory cytokines such as tumour necrosis factor (TNF)-alpha and interleukin (IL)-1β [Citation40]. M1 types of microglia could mediate inflammatory tissue damage, while type M2a, Mb and Mc microglial activation phenotypes have increased phagocytosis to remove cellular debris and to promote tissue repair [Citation40]. In the formalin group, this cytokine was also highly expressed, though to a lesser extent than in the MES group.
Our results showed high expression of CCL5 in the MES test group. Data exist that this chemokine is not only produced and released in response to seizures but may also cause seizures [Citation41]. The injection of formalin alone was also followed by a cytokine increase as compared to the control. The level of the expression, however, was not as high as the in the MES test group. The expression of several cytokines was elevated: BLC, C5/C5a, granulocyte colony-stimulating factor (G-CSF), granulocyte-macrophage colony-stimulating factor (GM-CSF), chemokine (C-C motif) ligand 1 (CCL1/I-139), sICAM-1, interferon gamma (IFN-gamma), interleukins (IL-1alpha, IL-1beta, IL-3, IL-4, IL-5, IL-6, IL-7, IL-10, IL-13, IL-12p70, IL-16, IL-17, IL-23), keratinocyte chemoattractant (KC), macrophage colony-stimulating factor (M-CSF), Japanese encephalitis/macrophage/monocyte chemotactic protein (JE/MCP-1), MCP-5, chemokine induced by gamma (MIG), macrophage inflammatory proteins (MIP-1β, MIP-2), tissue inhibitor of metalloproteinases 1 (TIMP-1), tumour necrosis factor (TNF-α), triggering receptor expressed on myeloid cells 1 (TREM1).
It is believed that proinflammatory cytokines that participate in the noxious process may originate from immune, neuronal and glial cells (microglia and astrocytes), both in the peripheral and central nervous system [Citation42]. They could also trigger short- and long-term effects, with occasional chronic hyper-excitability and changes in phenotypic expression of nociceptors, abnormal processing of noxious signals and exacerbation of pain processes (reviewed in [Citation31]). Interleukin-1β and TNF-α are the first two cytokines to be locally released after tissue damage. They affect directly specific receptors on sensorial neurons, leading to the ‘cascade’ synthesis of other effectors, forming a complex network of independent activation of nociception [Citation31,Citation42]. The first phase of the formalin paw test is attributed to the activation of primary afferent neurons (injury discharge). The activation of nociceptors by transient receptor potential ankyrin 1 (TRPA1) was accepted as the primary mechanism in formalin-induced pain [Citation43].
Additionally, calcium release from intracellular stores by inhibition of the sarco/endoplasmic reticulum Ca2+-ATPase (SERCA) and inhibition of sodium channels also play a role [Citation44]. The first phase (0–5 min) of the formalin test is believed to be caused by non-inflammatory pain resulting from direct stimulation of nociceptors. The second phase (10–45 min) is thought to be an inflammation-induced pain modulated by inflammatory cytokines in the periphery and spinal cord [Citation45,Citation46]. The results of our study showed increased expression of almost all tested cytokines. Our data add to the published knowledge, as there are surprisingly few works aimed to investigate the cytokine production during the formalin paw test.
The tested substances 8a, 8b, 4a, 4b, 4c, in general, alleviated the expression of most of the cytokines which were overexpressed after the formalin injection. As all of the tested substances had antiseizure activity, we might conclude that decreasing the level of brain cytokine expression could be one of the mechanisms involved in the antiseizure activity.
In silico predicted activities of aroylhydrazone-based molecular hybrids bearing coumarin or 2H-chromene-substituted moieties
Predicted physicochemical properties indicated that all five studied substances are liposoluble () and showed agreement with the Lipinski criteria for druggability. All tested compounds were preselected by their antiseizure activity. The common predicted activity of the five tested substances was their potential to activate the mitochondrial enzyme hydroxymethylglutaryl-CoA synthase 2 (EC 2.3.3.10, HMGCS2), that is the critical enzyme for the generation of ketone bodies. For substance 4a, membrane integrity agonism was also predicted. Loss of the mitochondrial membrane integrity during epileptic seizures can activate caspase-mediated proteolysis of neurons and the further release of oxygen-free radicals [Citation31,Citation47], which could be a feasible goal for neuroprotective strategies in the treatment of epilepsy.
Two of the substances bearing a furan-2-carbohydrazide moiety, that is 4b and 8b, were predicted to possess antagonistic activity towards myeloid cell leukaemia 1 (Mcl-1), an anti-apoptotic member of apoptosis-regulating proteins. Mcl-1 could also be a potential therapeutic target for some tumours [Citation48]. The predicted antimycobacterial and antimalarial (plasmodium falciparum aminopeptidase M1 inhibitor – PfA-M1) activities of the tested substances could shape further research with those substances away from the neuropharmacology. Beta-amyloid precursor protein (β-APP), also known as Aβ peptide, has a crucial role in the pathogenesis of Alzheimer’s disease and is also likely to be involved in the development of refractory epilepsy [Citation49,Citation50]. The mechanism behind the association between β-APP and refractory epilepsy remains unexplained.
Monoamine oxidase (MAO) inhibition properties were predicted for two of the tested substances, 4c and 8a. Although MAO inhibitors (MAO, EC 1.4.3.4) are well known to have anticonvulsant activity, they have not been clinically used due to their adverse effects. A study on the kindling model of epilepsy reveals that the inhibition of MAO-A is an effective way of inducing anticonvulsant activity [Citation51]. Inhibition of MAO may be an exciting strategy for developing novel anticonvulsant agents. Further proof of monoaminergic involvement in the pathogenesis of epilepsy is the high rate of comorbidities like depression, bipolar disorders and other neuropsychiatric disorders classically related to monoamine dysfunctions [Citation52]. Also, it seems that MAO-B might exert a critical role in the development of postoperative and neuropathic pain [Citation53]. The predicted activity for phobic disorder treatment of substance 8a could be most probably linked to the MAO inhibitory potential.
Role of HMGCS2 expression enhancers
Although not all of the tested substances showed analgesic activity, practically, all five decreased the cytokine expression in the homogenates as compared with the formaldehyde-treated group. We might suggest that cytokine overexpression only partially participates in the pain pathophysiology. Both epilepsy and neuropathic pain are pathological conditions with abnormal electrical discharges [Citation54]. At the molecular level, most if not all AEDs exert their activity by receptor or voltage-gated ion channels involved in normal neurotransmission. It is not surprising that substances with antiseizure activity, such as our aroylhydrazone hybrids, might be associated with suppression of inflammatory or neuropathic pain.
Could the predicted HMGCS2 enhancer activities of the tested substances be a plausible explanation of our findings related to the antiseizure or analgesic effects? It is known that the ketogenic diet is an established approach for the treatment of some cases of epilepsy in children. Ketone bodies (acetoacetate and β-hydroxybutyrate) are the primary sources of energy for most tissues during starvation, prolonged physical activity or ketogenic diet, and the brain utilizes them as a main metabolic substrate when glucose is sparse. They induce fundamental changes in the brain metabolism of glutamate, the primary excitatory neurotransmitter [Citation55]. Usually, ketone bodies are produced by the liver. Astrocytes, however, can generate ketone bodies, to protect the mitochondrial metabolism of neurons. This biological process is known as ‘neuron–glia metabolic coupling’ [Citation56]. Deficiency of the HMGCS2 enzyme is linked with genetic predisposition to epilepsy [Citation57]. In addition to the effects on neurotransmitters, recent studies broadly discuss the modulatory effect of ketone bodies on the mitochondrial function in cases of a cell danger, that is the so-called cell danger response [Citation58], that is adenosine triphosphate production, free radical generation and sequestration of calcium in the cytosol. For the brain, epilepsy is viewed as an example. The neuroprotective effects of the ketone bodies could be related to the activation of potassium channels (KATP) located both at the cellular surface and in mitochondria [Citation59]. Additionally, β-hydroxybutyrate has been demonstrated to inhibit histone deacetylases (EC 3.5.1.98, HDECs), enzymes which remove acetyl moieties from the lysine residues of the histones, transcription factors and others, that is to epigenetically transform the epileptogenesis [Citation60].
It has recently been discovered that β-hydroxybutyrate could also modulate another essential mechanism implicated in ictogenesis and epileptogenesis, the inflammation [Citation61] via two different targets – activation of the G-protein coupled hydroxycarboxylic acid receptor 2 (HCA2) expressed on the surface of monocytes and macrophages and through the inhibition of the inflammasome receptor protein NLRP3. The link between HMGCS2, peroxisome proliferator-activated receptors (PPARs) and cytokine production is also a strong temptation for further evaluations [Citation62]. Stimulation of autophagy is a mechanism by which ketone bodies increase the survival of the neurons [Citation63], generally impaired in epileptic seizures.
Conclusions
Recently synthesized aroylhydrazone hybrid molecules with antiseizure activity decreased the cytokine expression in the brain homogenates as compared both with MES and with formalin testing. Some of the tested substances also demonstrated analgesic potential. In silico evaluation predicted a possible HMGCS2 enhancer activity as highly plausible, based on the chemical structure. To prove this, we need to perform further experimental studies. Careful evaluation of the toxicity risk is also required. The other predicted activities, namely MAO inhibition and perhaps membrane integrity agonism as an explanation of their neuropharmacological activity, also seem to merit additional assessment.
Disclosure statement
No potential conflict of interest was reported by the authors.
Additional information
Funding
References
- National Institute for Health and Care Excellence [Internet]. Epilepsies: diagnosis and management. Clinical guideline [CG137]. 2018. Available from: https://www.nice.org.uk/guidance/cg137
- Ngugi AK, Kariuki SM, Bottomley C, et al. Incidence of epilepsy: a systematic review and meta-analysis. Neurology. 2011;77:1005–1012.
- Wilner AN. Comorbidities common even in newly diagnosed epilepsy. Medscape, C. 2016. Available from: https://www.medscape.com/viewarticle/872119
- Keezer MR, Sisodiya SM, Sander JW. Comorbidities of epilepsy: current concepts and future perspectives. Lancet Neurol. 2016;15:106–115.
- Aaberg KM, Bakken IJ, Lossius MI, et al. Comorbidity and childhood epilepsy: a nationwide registry study. Pediatrics. 2016;138:e20160921.
- Kerr MP. The impact of epilepsy on patients’ lives. Acta Neurol Scand. 2012;194:1–9.
- St Germaine-Smith C, Liu M, Quan H, et al. Development of an epilepsy-specific risk adjustment comorbidity index. Epilepsia. 2011;52:2161–2167.
- Goldberg DS, McGee SJ. Pain as a global public health priority. BMC Public Health. 2011;11:770.
- Sidhu HS, Sadhotra A. Current status of the new antiepileptic drugs in chronic pain. Front Pharmacol. 2016;7:276.
- Sörensen J, Graven-Nielsen T, Henriksson KG, et al. Hyperexcitability in fibromyalgia. J Rheumatol. 1998;25:152–155.
- Ferrari MD, Klever RR, Terwindt GM, et al. Migraine pathophysiology: lessons from mouse models and human genetics. Lancet Neurol. 2015;14:65–80.
- Ottman R, Lipton RB, Ettinger AB, et al. Comorbidities of epilepsy: results from the Epilepsy Comorbidities and Health (EPIC) survey. Epilepsia. 2011;52:308–315.
- Cianchetti C, Avanzini G, Dainese F, et al. The complex interrelations between two paroxysmal disorders: a headache and epilepsy. Neurol Sci. 2017;38:941–948.
- Velioglu SK, Gedikli O, Yıldırım M, et al. Epilepsy may cause increased pain sensitivity: evidence from absence epileptic WAG/Rij rats. Epilepsy Behav. 2017;75:146–150.
- de Freitas RL, Bolognesi LI, Twardowschy A, et al. Neuroanatomical and neuropharmacological approaches to postictal antinociception-related prosencephalic neurons: the role of muscarinic and nicotinic cholinergic receptors. Brain Behav. 2013;3:286–301.
- Szűcs A, Horváth A, Rásonyi G, et al. Ictal analgesia in temporal lobe epilepsy – the mechanism of seizure-related burns. Med Hypotheses. 2015;85:173–177.
- Wiffen PJ, Derry S, Moore RA, et al. Antiepileptic drugs for neuropathic pain and fibromyalgia – an overview of Cochrane reviews. Cochrane Database Syst Rev. 2013;CD010567.
- Hoffmann J, Akerman S, Goadsby PJ. Efficacy and mechanism of anticonvulsant drugs in migraine. Expert Rev Clin Pharmacol. 2014;7:191–201.
- Jackson JL, Cogbill E, Santana-Davila R, et al. A comparative effectiveness meta-analysis of drugs for the prophylaxis of migraine headache. PLoS One. 2015;10:e0130733.
- Ibrar A, Shehzadi SA, Saeed F, et al. Developing hybrid molecule therapeutics for diverse enzyme inhibitory action: the active role of coumarin-based structural leads in drug discovery. Bioorg Med Chem. 2018;26:3731–3762.
- Gateva P, Tchekalarova J, Angelova VT, et al. Preclinical screening of coumarin and 2 h –chromene substituted hydrazide-hydrazone derivatives, as potential anticonvulsants. Clin Ther. 2017;39:e78–e79.
- Chaur MN. Aroylhydrazones as potential systems for information storage: photoisomerization and metal complexation. Rev Colomb Quim. 2012;41:349–358.
- Torres FC, Brucker N, Andrade SF, et al. New insights into the chemistry and antioxidant activity of coumarins. Curr Top Med Chem. 2014;14:2600–2623.
- Hiragi T, Ikegaya Y, Koyama R. Microglia after seizures and in epilepsy. Cells. 2018;7:26.
- de Vries EE, van den Munckhof B, Braun KP, et al. Inflammatory mediators in human epilepsy: a systematic review and meta-analysis. Neurosci Biobehav Rev. 2016;63:177–190.
- Ramesh G, MacLean AG, Philipp MT. Cytokines and chemokines at the crossroads of neuroinflammation, neurodegeneration, and neuropathic pain. Mediators Inflamm. 2013;2013:1.
- Bannon AW, Malmberg AB. Models of nociception: hot-plate, tail-flick, and formalin tests in rodents. Curr Protoc Neurosci. 2007;41:891–896.
- Eddy NB, Leimbach DJ. Synthetic analgesics. II. Dithienylbutenyl- and d dithienylbutylamines. J Pharmacol Exp Ther. 1953;107:385–393.
- Riedel W, Neeck G. Nociception, pain, and antinociception: current concepts. Z Rheumatol. 2001;60:404–415.
- Stanley AC, Lacy P. Pathways for cytokine secretion. Physiology (Bethesda). 2010;25:218–229.
- de Oliveira CM, Sakata RK, Issy AM, et al. Cytokines and pain. Rev Bras Anestesiol. 2011;61:255–259.
- Okuneva O, Li Z, Körber I, et al. Brain inflammation is accompanied by peripheral inflammation in Cstb -/- mice, a model for progressive myoclonus epilepsy. J Neuroinflammation. 2016;13:298.
- Strong JA, Xie W, Coyle DE, et al. Microarray analysis of rat sensory ganglia after local inflammation implicates novel cytokines in pain. PLoS One. 2012;7:e40779.
- Luo J, Wang W, Xi Z, et al. Concentration of soluble adhesion molecules in cerebrospinal fluid and serum of epilepsy patients. J Mol Neurosci. 2014;54:767–773.
- Luchting B, Hinske LC, Rachinger-Adam B, et al. Soluble intercellular adhesion molecule-1: a potential biomarker for pain intensity in chronic pain patients. Biomarkers Med. 2017;11:265–276.
- Kodangattil JN, Möddel G, Müller M, et al. The inflammatory chemokine CXCL10 modulates synaptic plasticity and neuronal activity in the hippocampus. Eur J Inflamm. 2012;10:311–328.
- Vazirinejad R, Ahmadi Z, Kazemi Arababadi M, et al. The biological functions, structure and sources of CXCL10 and its outstanding part in the pathophysiology of multiple sclerosis. Neuroimmunomodulation. 2014;21:322–330.
- Wang Y, Gehringer R, Mousa SA, et al. CXCL10 controls inflammatory pain via opioid peptide-containing macrophages in electroacupuncture. PLoS One. 2014;9:e94696.
- Walker DG, Lue LF. Immune phenotypes of microglia in human neurodegenerative disease: challenges to detecting microglial polarization in human brains. Alzheimers Res Ther. 2015;7:56.
- Martinez FO, Gordon S, Locati M, et al. Transcriptional profiling of the human monocyte-to-macrophage differentiation and polarization: new molecules and patterns of gene expression. J Immunol. 2006;177:7303–7311.
- Cerri C, Caleo M, Bozzi Y. Chemokines as new inflammatory players in the pathogenesis of epilepsy. Epilepsy Res. 2017;136:77–83.
- Zhang JM, An J. Cytokines, inflammation, and pain. Int Anesthesiol Clin. 2007;45:27–37.
- McNamara CR, Mandel-Brehm J, Bautista DM, et al. TRPA1 mediates formalin-induced pain. Proc Natl Acad Sci USA. 2007;104:13525–13530.
- Fischer M, Carli G, Raboisson P, et al. The interphase of the formalin test. Pain. 2014;155:511–521.
- Abbott FV, Franklin KB, Westbrook RF. The formalin test: scoring properties of the first and second phases of the pain response in rats. Pain. 1995;60:91–102.
- Yin ZY, Li L, Chu SS, et al. Antinociceptive effects of dehydrocorydaline in mouse models of inflammatory pain involve the opioid receptor and inflammatory cytokines. Sci Rep. 2016;6:27129.
- Naegele JR. Neuroprotective strategies to avert seizure-induced neurodegeneration in epilepsy. Epilepsia. 2007;48:107–117.
- Akgul C. Mcl-1 is a potential therapeutic target in multiple types of cancer. Cell Mol Life Sci. 2009;66:1326–1336.
- Thomas LW, Lam C, Edwards SW. Mcl-1: the molecular regulation of protein function. FEBS Lett. 2010;584:2981–2989.
- Sima X, Xu J, Li J, et al. Expression of β-amyloid precursor protein in refractory epilepsy. Mol Med Rep. 2014;9:1242–1248.
- Löscher W, Lehmann H, Teschendorf HJ, et al. Inhibition of monoamine oxidase type A, but not type B, is an effective means of inducing anticonvulsant activity in the kindling model of epilepsy. J Pharmacol Exp Ther. 1999;288:984–992.
- Svob Strac D, Pivac N, Smolders IJ, et al. Monoaminergic mechanisms in epilepsy may offer innovative therapeutic opportunity for monoaminergic multi-target drugs. Front Neurosci. 2016;10:492.
- Villarinho JG, Oliveira SM, Silva CR, et al. Involvement of monoamine oxidase B on models of postoperative and neuropathic pain in mice. Eur J Pharmacol. 2012;690:107–114.
- Barrot M. Tests and models of nociception and pain in rodents. Neuroscience. 2012;211:39–50.
- Yudkoff M, Daikhin Y, Nissim I, et al. Ketogenic diet, brain glutamate metabolism and seizure control. Prostaglandins Leukot Essent Fatty Acids. 2004;70:277–285.
- Guzmán M, Blázquez C. Is there an astrocyte-neuron ketone body shuttle? Trends Endocrinol Metab. 2001;12:169–173.
- Wang J, Lin ZJ, Liu L, et al. Epilepsy-associated genes. Seizure. 2017;44:11–20.
- Naviaux RK. Metabolic features of the cell danger response. Mitochondrion. 2014;16:7–17.
- Kim DY, Abdelwahab MG, Lee SH, et al. Ketones prevent oxidative impairment of hippocampal synaptic integrity through KATP channels. PLoS One. 2015;10:e0119316.
- Shimazu T, Hirschey MD, Newman J, et al. Suppression of oxidative stress by β-hydroxybutyrate, an endogenous histone deacetylase inhibitor. Science. 2013;339:211–214.
- Marchi N, Granata T, Janigro D. Inflammatory pathways of seizure disorders. Trends Neurosci. 2014;37:55–65.
- Grabacka M, Pierzchalska M, Dean M, et al. Regulation of ketone body metabolism and the role of PPARα. IJMS. 2016;17:2093.
- Rojas-Morales P, Tapia E, Pedraza-Chaverri J. β-Hydroxybutyrate: a signaling metabolite in starvation response? Cell Signal. 2016;28:917–923.