Abstract
Tonoplast sugar transporters (TSTs) usually act as antiporters to import sugars across the tonoplast and play essential roles in regulating diverse growth and developmental processes as well as in response to various abiotic stresses in plants. In this study, a putative TST gene named CsTST2 was isolated and characterized from cucumber (Cucumis sativus L.). CsTST2 possesses a 2202-bp open reading frame encoding a highly hydrophobic protein of 733 amino acids with a molecular weight (MW) of 78.30 kDa and an isoelectric point of 5.13. Multiple sequence alignment and phylogenetic analysis suggested that CsTST2 has the highest identity and closest phylogenetic relationship with CmTST2 and ClTST2. Expression analysis with transcriptome data revealed that CsTST2 has differential expression patterns in various tissues and developmental stages. Quantitative reverse transcription polymerase chain reaction (qRT-PCR) showed that CsTST2 is responsive to various abiotic stresses, inducing salt, drought and cold. Yeast complementation assays indicated that CsTST2 can mediate the uptake of fructose, galactose, mannose and sucrose. These findings suggest that CsTST2 is a functional TST in cucumber, and lay a foundation for revealing the relationship of carbohydrate distribution and abiotic stress in the growth and development of cucumber.
Introduction
Sugars (predominantly sucrose) are synthesized in ‘source’ tissues (photosynthesizing leaves) via photosynthesis and loaded into the phloem for long-distance transport, and finally released into non-photosynthetic ‘sink’ tissues as the source of carbon and energy for plant growth and development. The assimilated sugars can also be allocated into storage pools and then stored as starch in plastids, oil in oil bodies, or sugars in the vacuoles [Citation1–3]. The vacuole is the largest organelle in plant cells, and a set of transporters are present in the vacuolar membrane (tonoplast) to mediate the import and export of sugars and other metabolites, which play vital roles in controlling cellular pH levels, carbohydrate distribution, sugar signalling and so on [Citation4,Citation5].
The transport of sugars across the tonoplast depends on several types of sugar transporters including sucrose transporters (SUTs), monosaccharide transporters (MSTs) and sugars will eventually be exported transporters (SWEETs) [Citation6,Citation7]. For example, many members of the SUT4 clade from different plant species were revealed to function as H+/sucrose symporters to export sucrose from the vacuole and regulate the intracellular sucrose partitioning [Citation8–11]. In addition, early response to dehydration 6 (ERD 6)-like transporters (ERD6 or ERDL6 or ESL1) can act as H+/glucose symporters to facilitate the export of glucose from the vacuole to the cytosol [Citation12–14], whereas sugars are imported into vacuoles primarily by two types of MSTs: tonoplast monosaccharide transporters (TMTs) and vacuolar glucose transporters (VGTs) [Citation15–18]. Besides, some SWEET proteins from Clade IV are also involved in sucrose and/or monosaccharide transport across the tonoplast, such as AtSWEET16 [Citation19], AtSWEET17 [Citation20,Citation21], AtSWEET2 [Citation22], and CsSWEET17a [Citation23].
Considering that some TMTs (such as AtTMT1, AtTMT2 and BvTST2.1) can also function as H+/sucrose antiporters to deliver sucrose into the vacuole, these transporter family proteins were renamed as tonoplast sugar transporters (TSTs) [Citation18,Citation24]. TSTs belong to the major facilitator superfamily (MFS) and usually possess 12 transmembrane (TM) helices separated by a central cytosolic loop between helices 6 and 7 [Citation25–27]. In recent years, many TST family genes have been identified and characterized at genome-wide level in various plant species, such as tomato [Citation28], sweet orange [Citation29], sweet sorghum [Citation30], melon [Citation31], watermelon [Citation27], and pear [Citation25,Citation32,Citation33]. In addition, several TST genes have been functionally characterized for their roles in sugar transport across the tonoplast. For example, BvTST2.1 acts as a H+/sucrose antiporter and plays an essential role in vacuolar sucrose uptake in sugar beet [Citation24]. The expression of PbTMT4 is correlated with glucose and fructose contents during pear fruit development, and PbTMT4 could mediate the transport of glucose and fructose when expressed in a yeast mutant strain EBY.VW4000, suggesting that PbTMT4 is involved in the control of sugar accumulation in pear fruit [Citation32]. These findings indicate that TST proteins can act as important sugar transporters and are responsible for sugar accumulation during plant growth and development.
Although many TST genes have been isolated and characterized to be involved in sugar transport across the tonoplast, no TST homolog has been reported for cucumber (Cucumis sativus L.), one of the most important vegetable crops consumed worldwide. In this study, a putative TST gene named CsTST2 was cloned and characterized from cucumber, and its expression profiles in different tissues and under various abiotic stresses were examined. In addition, the transport capacities of CsTST2 for various sugars were determined by heterologous expression in two yeast mutants (EBY.VW4000 and SUSY7/ura). Our results will facilitate a better understanding of the sugar transport across the tonoplast in cucumber and the mediation of stress response by sugar transporters in plants.
Materials and methods
Plant material and yeast strains
Cucumber (C. sativus L. var. sativus cv. 9930) was used in this study, and the plants were grown in trays containing soil mixture at 24/18 °C (day/night) temperature with a 16 h light/8 h dark photoperiod. The yeast strains (Saccharomyces cerevisiae EBY.VW4000 and SUSY7/ura) were used for yeast complementation assays.
Cloning of the CsTST2 gene
Total RNA was isolated from the leaves at the 20 main-stem node stage using TransZol reagent (TransGen Biotech, Beijing, China) according to the manufacturer’s protocol. The first-strand cDNA was synthesized using TransScript II First-Strand cDNA Synthesis SuperMix kit (TransGen Biotech, Beijing, China) as described in the user instructions. A pair of gene-specific primers (CsTST2-1F: 5′-CCCGGGATGAGTGGTTCTGTTTTGG-3′ and CsTST2-1R: 5′-GTCGACTCAACCATTTTTGGCAGATA-3′) were designed based on the open reading frame (ORF) of CsTST2 (Gene ID: Csa6G118280) available in the cucumber genome database (http://cucurbitgenomics.org/organism/2). Semi-quantitative reverse transcription polymerase chain reaction (RT-PCR) was performed with KOD FX DNA Polymerase (TOYOBO, Japan) on an ABI 9700 thermal cycler (Thermo Fisher Scientific, USA), and the thermal cycling conditions were as follows: 98 °C denaturation for 5 min, followed by 30 cycles of 98 °C for 10 s, 58 °C for 30 s, 68 °C for 30 s, and a final extension of 68 °C for 5 min. The resulting PCR product was ligated into a Blunt vector of pEASY-Blunt3 Cloning Kit (TransGen Biotech, Beijing, China), and the resulting Blunt3-CsTST2 clone was sequenced (Sangon, Shanghai, China).
Bioinformatics analysis of putative CsTST2 protein
The primary and secondary structures of CsTST2 were analyzed by the programs ProtParam (http://web.expasy.org/protparam/) and SOPMA (https://npsa-prabi.ibcp.fr/cgi-bin/npsa_automat.pl?page=/NPSA/npsa_sopma.html), respectively. The SMART tool (http://smart.embl-heidelberg.de/) was employed to determine the MFS domains of the CsTST2 protein. The TM analysis was performed using the full-length CsTST2 protein sequence with the TMHMM 2.0 tool (http://www.cbs.dtu.dk/services/TMHMM/). Multiple sequence alignments of CsTST2 and other identified TST proteins were performed with Clustal Omega (https://www.ebi.ac.uk/Tools/msa/clustalo/) with default parameters, and then the alignments were modified with GeneDoc software. For phylogenetic analysis, the full-length protein sequences of CsTST2 and TST family proteins from various plant species including Cucumis melo, Citrullus lanatus, Arabidopsis thaliana, Vitis vinifera, Solanum lycopersicum and Beta vulgaris were aligned with Clustal Omega, and then a phylogenetic tree was generated using the MEGA 5.0 software with the neighbour-joining (NJ) method. The bootstrap replicates were set at 1000.
Expression analysis of CsTST2
To study the in silico tissue expression patterns of CsTST2 in cucumber, online transcriptome data were obtained from the NCBI (National Center of Biotechnology Information) database (https://www.ncbi.nlm.nih.gov/sra/?term=sra046916) and the FPKM values of CsTST2 were analyzed according to previous studies [Citation34–36].
To examine the effects of various abiotic stresses on the expression of CsTST2, treatments of various stresses, including salt, drought and cold, were applied to cucumber seedlings as described previously [Citation35], and the total RNA of leaves after 0, 3, 6 and 12 h of treatment was extracted. The RNA samples were reverse transcribed into cDNA using TransScript All-in-One First-Strand cDNA Synthesis SuperMix for qPCR (One-Step gDNA Removal) (TransGen Biotech, Beijing, China), and quantitative real-time PCR (qRT-PCR) was carried out in triplicate with the FastStar Universal SYBR Green Master (ROX) (Roche, USA) on the ABI 7500 system (Thermo Fisher Scientific, USA). The relative expression of CsTST2 was calculated using the CsAct3 gene as an endogenous reference with the 2–ΔΔCT method [Citation37]. The reaction conditions were 95 °C for 30 s, followed by 40 cycles of 95 °C for 5 s, and 60 °C for 30 s. The corresponding primers for expression analysis were as follows: CsTST2-2F: 5′-AGACCAGTTACAGGACAGAGTT-3′ and CsTST2-2R: 5′-CGAAGAGAGTGACGAGAGGAT-3′; CsAct3F: 5′-GGCAGTGGTGGTGAACAT-3′ and CsAct3R: 5′-GATTCTGGTGATGGTGTGAGTC-3′.
Functional analysis of CsTST2 in yeast
The yeast complementation assays were carried out using S. cerevisiae strains EBY.VW4000 (hexose uptake deficient) [Citation38] and SUSY7/ura (sucrose uptake deficient) [Citation39], for which maltose and glucose should be added into the medium as the sole carbon source, respectively. The positive Blunt3-CsTST2 clone was cleaved by Xma I and Sal I, and inserted into the Xma I/Sal I cleaved pDR196 vector. The resulting pDR196-CsTST2 vector and the empty pDR196 vector (negative control) were introduced into yeast strains EBY.VW4000 and SUSY7/ura, and the positive transformants were grown and selected as previously described [Citation40].
For complementation assays of hexose, the positive transformants were grown overnight in liquid minimal medium containing 2% maltose to an optical density at 600 nm (OD600) of 0.6. Then, serial dilutions (10-, 100- and 1000-fold) of transformants were performed, and 3 μL dilution was dropped on solid minimal medium containing either 2% maltose (as positive control), or 2% fructose, 2% glucose, 2% galactose, 2% mannose, 2% arabinose and 2% xylose, respectively. For complementation assays of sucrose, the positive transformants were grown overnight in liquid minimal medium containing 2% glucose until OD600 reached 0.6. After the same serial dilutions, 3 μL dilution was dropped on solid minimal medium containing 2% sucrose. All the transformant-containing plates were placed at 30 °C until yeast growth and then were documented by pictures.
Results and discussion
Cloning and sequence analysis of CsTST2
A recent study has reported that a TST CmTST2 plays a crucial role in the accumulation of fructose, glucose and sucrose in fruit, indicating that CmTST2 can mediate the transport of these sugars into the vacuole [Citation31]. To identify the functional TSTs in cucumber, a BlastP search was performed against the cucumber genome database (http://cucurbitgenomics.org/organism/2) using the protein sequence of CmTST2 as a query. As a result, one candidate (Csa6G118280) displaying the highest identity with CmTST2 was identified and was designated as CsTST2. Gene-specific primers were designed to amplify the ORF of CsTST2 by RT-PCR. The sequencing results indicated that the ORF of CsTST2 consists of 2202 bp that encode a putative protein of 733 amino acids with a calculated molecular weight (MW) of 78.30 kDa and a theoretical isoelectric point (pI) of 5.13. SMART analysis showed that the CsTST2 protein contains two MFS domains, which are located at the N- and C-terminus, respectively (), indicating that CsTST2 belongs to the MFS. In addition, the predicted grand average of hydropathicity (GRAVY) value of CsTST2 was 0.418, implying that the CsTST2 protein is highly hydrophobic.
Figure 1. Nucleotide sequence of CsTST2 and its deduced amino acids. The N- and C-terminal MFS domains are underlined by purple and blue, respectively. The termination codon is marked by an asterisk.
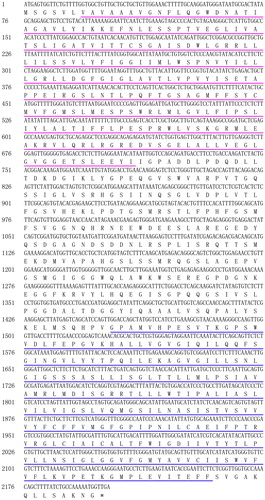
We also performed secondary structure analysis of CsTST2 by the SOPMA program. The results showed that the CsTST2 protein possesses 42.43% of random coil, 34.79% of alpha helix, 17.46% of extended strand and 5.32% of beta turn (). Most studied MFS sugar transporters possess a typical structure with 12 putative TM helices and a central hydrophilic region [Citation16,Citation26,Citation41]. TM analysis by TMHMM revealed that CsTST2 also contains 12 predicted TM helices, and there is a large hydrophilic loop between TM helices 6 and 7 (), which is similar to the characteristics of previously identified TST proteins, such as CmTST2, ClTST2 and AtTMT2 [Citation17,Citation27,Citation31].
Protein sequence alignment and phylogenetic analysis of CsTST2
Multiple sequence alignment was performed using the full-length amino acid sequences of CsTST2 and previously identified tonoplast-localized TST proteins from various plant species, including Arabidopsis (AtTMT2) [Citation17], rice (OsTMT2) [Citation42], grape (VvTMT2) [Citation43], tomato (SlTMT2) [Citation28], sugar beet (BvTST2.1) [Citation24], sweet sorghum (SbTST2) [Citation30], melon (CmTST2) [Citation31] and watermelon (ClTST2) [Citation27]. The alignment results revealed that CsTST2 shares high identity (70.30–98.50%) with its homologous proteins in amino acid sequences (), such as BvTST2.1 (70.30%), OsTMT2 (72.64%), SbTST2 (72.74%), SlTMT2 (73.97%), VvTMT2 (77.67%), AtTMT2 (78.66%), ClTST2 (95.62%) and CmTST2 (98.50%). In addition, all of these proteins possess two sets of six TM helices separated by an extended hydrophilic loop, and the structural similarity indicated that CsTST2 is very likely a functional tonoplast-localized TST homolog in cucumber.
Figure 3. Sequence alignment of amino acid sequences of CsTST2 and TST proteins from various plant species. The accession numbers and sources of the TST proteins are as follows: Cucumis melo CmTST2 (XP_008448165.1), Citrullus lanatus ClTST2 (Cla000264, http://cucurbitgenomics.org/organism/1), Arabidopsis thaliana AtTMT2 (At4g35300, https://www.arabidopsis.org/), Vitis vinifera VvTMT2 (HQ323283.1), Solanum lycopersicum SlTMT2 (Solyc04g082700, https://phytozome.jgi.doe.gov/Slycopersicum), Sorghum bicolor SbTST2 (Sobic.004G099300, https://phytozome.jgi.doe.gov/Sbicolor), Oryza sativa OsTMT2 (LOC_Os02g13560, http://rice.plantbiology.msu.edu/) and Beta vulgaris BvTST2.1 (XP_010678631). The predicted TM helices are numbered and underlined.
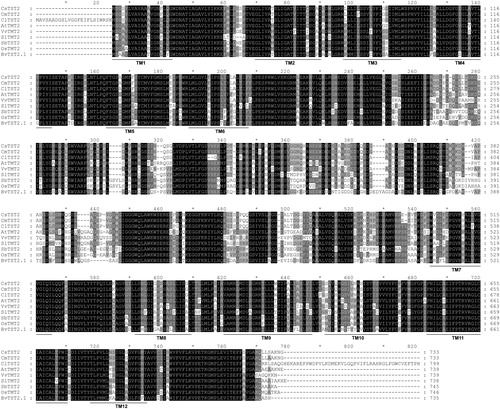
We also generated a phylogenetic tree based on the alignment of CsTST2 and TST proteins from various plant species. These TST proteins could be grouped into three clades, and CsTST2 was more closely related to CmTST2 and ClTST2 in Clade 2 (), indicating that they may be functionally similar.
Figure 4. Phylogenetic analysis of CsTST2 and TST proteins from various plant species. The phylogenetic tree was generated using the NJ method with MEGA 5.0 software, with bootstrap support of 1000 replicates. The names and accession numbers of the TST proteins are provided in Table S1.
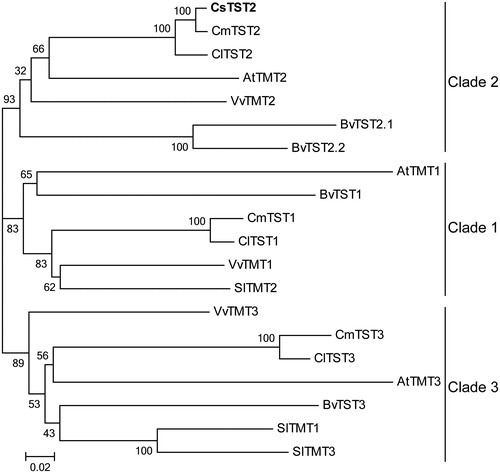
Tissue expression profiles of CsTST2 in cucumber
To examine the possible functions of CsTST2 during the development of cucumber, the raw transcriptome data were downloaded and analyzed according to previous studies [Citation34–36]. As a result, the highest expression of CsTST2 was observed in stems while significantly lower transcript levels were found in tendril bases and tendrils (). Sucrose can move into the stems through an apoplastic transport step, and many TST genes exhibit high expression levels in stems, such as PbTMT5 [Citation25], CmTST1 and CmTST2 [Citation31], indicating that they may function in importing sugars for storage in the vacuole within the stem parenchyma cells. In addition, the transcription of CsTST2 was higher in unexpanded ovaries than in fertilized ovaries and was the lowest in unfertilized ovaries (), indicating that CsTST2 may participate in the ovary development of cucumber. Sugars are always accumulated in the vacuoles as glucose and fructose during ripening, and both of the homologs of CsTST2 (CmTST2 and ClTST2) are located in vacuoles and their expression is positively related to sugar accumulation in fruits [Citation27,Citation31]. Some pear TST genes (PbTMT2, PbTMT3 and PbTMT4) have also been found to be correlated with the levels of fructose and glucose during pear fruit development [Citation32,Citation33]. Moreover, CsTST2 showed relatively high expression in male and female flowers (), suggesting that it may also be involved in the transport of sugars for flower development in cucumber. Similar results have also been found for TST members in other plant species, such as CmTST2 [Citation31], PbTMT4 and PbTMT5 [Citation25]. Overexpression of PbTMT4 in tomato resulted in early flowering and ripening phenotypes compared with wild-type plants [Citation32]. Furthermore, CsTST2 also displayed moderate expression levels in leaves and roots (), implying that it may also play a role in these organs.
Expression analysis of CsTST2 under various abiotic stresses
Source-to-sink transport of sugars can modify the carbohydrate homeostasis in plant cells, which is important for plant abiotic stress tolerance [Citation44,Citation45], and many sugar transporter genes are responsive to various biotic and abiotic stresses. For example, SWEETs are a class of H+-independent hexose and sucrose transporters and play essential roles in pathogen nutrition and abiotic stress tolerance [Citation46,Citation47]. The expression of both AtTMT1 and AtTMT2 is induced by salt, drought and cold stress [Citation17]. To determine whether CsTST2 is responsive to various abiotic stresses, we performed qRT-PCR to measure the transcript levels of CsTST2 under cold, salt and drought stress conditions. Under cold stress conditions, the transcription of CsTST2 was markedly induced and reached the highest level (3.47-fold) at 3 h, and then gradually decreased at 6 h and 12 h (). Under salt treatment, the transcription of CsTST2 increased at 3 h, followed by a decrease at 6 h and increase again at 12 h (). Under drought conditions, the expression of CsTST2 was induced at 3 h and continued to increase at 6 h to reach the maximum (1.94-fold), followed by a decline at 12 h (). These findings suggested that CsTST2 also plays key roles in response to various abiotic stresses.
Heterologous expression and substrate specificity analysis of CsTST2 in yeast
CsTST2 shares high identity with other identified TST proteins, indicating that CsTST2 may also be involved in sugar transport. To determine whether CsTST2 acts as a functional sugar transporter, the ORF of CsTST2 was cloned into the yeast expression vector pDR196 and introduced into the yeast mutants EBY.VW4000 and SUSY7/ura3, which showed hexose and sucrose uptake deficiency, respectively. As shown in , compared with the EBY.VW4000 transformants containing pDR196, the transformants containing CsTST2 showed restored growth on the medium containing fructose, galactose and mannose, but not on those containing glucose, arabinose or xylose, indicating that CsTST2 contributes to the uptake of fructose, galactose and mannose. However, heterologous expression of CsTST2 was unable to restore the growth on the medium containing glucose (). The hexose transport capacity of CsTST2 is different from that of other identified TSTs, such as VvTMT1 [Citation48], PbTMT4 [Citation32], ClTST2 [Citation27], AtTMT1 and AtTMT2 [Citation17,Citation18], implying that there might be other sugar transporters to import glucose into the vacuoles of cucumber. In addition, the growth of the SUSY7/ura3 transformants was restored to some extent on the medium containing sucrose, suggesting that CsTST2 also enables sucrose uptake (). Similarly, AtTMT1, AtTMT2 and BvTST2.1 can also facilitate the loading of sucrose into the vacuoles [Citation18,Citation24]. These results indicate that CsTST2 may also be responsible for sugar accumulation in cucumber.
Conclusion
In this study, we identified a TST gene named CsTST2 from cucumber. The protein alignment and phylogenetic analysis suggested that CsTST2 shares high identity with other reported TST proteins from Cucumis and Citrullus species. The expression of CsTST2 varies among different tissues, and is altered under various abiotic stresses, including salt, drought, and cold. CsTST2 enables the uptake of fructose, galactose, mannose and sucrose in yeast, suggesting that it may participate in the import of these sugars into the vacuoles for sugar accumulation in cucumber. This is the first report of a functional TST in cucumber, and further studies are needed to reveal its roles in the growth and development as well as abiotic stress responses of cucumber.
Acknowledgements
We thank Dr. Xiaolei Sui (China Agricultural University) for generously providing the pDR196 vector and yeast strains EBY.VW4000 and SUSY7/ura.
Disclosure statement
The authors declare that they have no competing financial interests.
Funding
This research was funded by the Key Project of Youth Science Foundation of Jiangxi Province under grant number 20171ACB21025 and the National Natural Science Foundation of China under grant number 31760074.
References
- Durand M, Mainson D, Porcheron B, et al. Carbon source–sink relationship in Arabidopsis thaliana: the role of sucrose transporters. Planta. 2018;247:587–611.
- Zhou Y, Liu L, Huang W, et al. Overexpression of OsSWEET5 in rice causes growth retardation and precocious senescence. PLoS One. 2014;9:e94210. DOI: 10.1371/journal.pone.0094210
- Ludewig F, Flugge UI. Role of metabolite transporters in source-sink carbon allocation. Front Plant Sci. 2013;4:231. DOI: 10.3389/fpls.2013.00231
- Martinoia E, Meyer S, De Angeli A, et al. Vacuolar transporters in their physiological context. Annu Rev Plant Biol. 2012;63:183–213.
- Martinoia E. Vacuolar transporters - companions on a longtime journey. Plant Physiol. 2018;176:1384–1407.
- Doidy J, Grace E, Kuhn C, et al. Sugar transporters in plants and in their interactions with fungi. Trends Plant Sci. 2012;17:413–422.
- Chen LQ, Hou BH, Lalonde S, et al. Sugar transporters for intercellular exchange and nutrition of pathogens. Nature. 2010;468:527–532.
- Eom JS, Cho JI, Reinders A, et al. Impaired function of the tonoplast-localized sucrose transporter in rice, OsSUT2, limits the transport of vacuolar reserve sucrose and affects plant growth. Plant Physiol. 2011;157:109–119.
- Reinders A, Sivitz AB, Starker CG, et al. Functional analysis of LjSUT4, a vacuolar sucrose transporter from Lotus japonicus. Plant Mol Biol. 2008;68:289–299.
- Schneider S, Hulpke S, Schulz A, et al. Vacuoles release sucrose via tonoplast-localised SUC4-type transporters. Plant Biol (Stuttg). 2012;14:325–336.
- Payyavula RS, Tay KH, Tsai CJ, et al. The sucrose transporter family in Populus: the importance of a tonoplast PtaSUT4 to biomass and carbon partitioning. Plant J. 2011;65:757–770.
- Yamada K, Osakabe Y, Mizoi J, et al. Functional analysis of an Arabidopsis thaliana abiotic stress-inducible facilitated diffusion transporter for monosaccharides. J Biol Chem. 2010;285:1138–1146.
- Poschet G, Hannich B, Raab S, et al. A novel Arabidopsis vacuolar glucose exporter is involved in cellular sugar homeostasis and affects the composition of seed storage compounds. Plant Physiol. 2011;157:1664–1676.
- Klemens PA, Patzke K, Trentmann O, et al. Overexpression of a proton-coupled vacuolar glucose exporter impairs freezing tolerance and seed germination. New Phytol. 2014;202:188–197.
- Aluri S, Buttner M. Identification and functional expression of the Arabidopsis thaliana vacuolar glucose transporter 1 and its role in seed germination and flowering. Proc Natl Acad Sci USA. 2007;104:2537–2542.
- Buttner M. The monosaccharide transporter(-like) gene family in Arabidopsis. FEBS Lett. 2007;581:2318–2324.
- Wormit A, Trentmann O, Feifer I, et al. Molecular identification and physiological characterization of a novel monosaccharide transporter from Arabidopsis involved in vacuolar sugar transport. Plant Cell. 2006;18:3476–3490.
- Schulz A, Beyhl D, Marten I, et al. Proton-driven sucrose symport and antiport are provided by the vacuolar transporters SUC4 and TMT1/2. Plant J. 2011;68:129–136.
- Klemens PA, Patzke K, Deitmer J, et al. Overexpression of the vacuolar sugar carrier AtSWEET16 modifies germination, growth, and stress tolerance in Arabidopsis. Plant Physiol. 2013;163:1338–1352.
- Chardon F, Bedu M, Calenge F, et al. Leaf fructose content is controlled by the vacuolar transporter SWEET17 in Arabidopsis. Curr Biol. 2013;23:697–702.
- Guo WJ, Nagy R, Chen HY, et al. SWEET17, a facilitative transporter, mediates fructose transport across the tonoplast of Arabidopsis roots and leaves. Plant Physiol. 2014;164:777–789.
- Chen HY, Huh JH, Yu YC, et al. The Arabidopsis vacuolar sugar transporter SWEET2 limits carbon sequestration from roots and restricts Pythium infection. Plant J. 2015;83:1046–1058.
- Li Y, Feng S, Ma S, et al. Spatiotemporal expression and substrate specificity analysis of the cucumber SWEET gene family. Front Plant Sci. 2017;8:1855. DOI: 10.3389/fpls.2017.01855
- Jung B, Ludewig F, Schulz A, et al. Identification of the transporter responsible for sucrose accumulation in sugar beet taproots. NPLANTS. 2015;1:14001. DOI: 10.1038/nplants.2014.1
- Cheng R, Zhang HP, Cheng YS, et al. In silico and expression analysis of the tonoplast monosaccharide transporter (TMT) gene family in Pyrus bretschneideri. J Hortic Sci Biotech. 2018;93:366–376.
- Yan N. Structural biology of the major facilitator superfamily transporters. Annu Rev Biophys. 2015;44:257–283.
- Ren Y, Guo S, Zhang J, et al. A tonoplast sugar transporter underlies a sugar accumulation QTL in watermelon. Plant Physiol. 2018;176:836–850.
- Reuscher S, Akiyama M, Yasuda T, et al. The sugar transporter inventory of tomato: genome-wide identification and expression analysis. Plant Cell Physiol. 2014;55:1123–1141.
- Zheng QM, Tang Z, Xu Q, et al. Isolation, phylogenetic relationship and expression profiling of sugar transporter genes in sweet orange (Citrus sinensis). Plant Cell Tiss Organ Cult. 2014;119:609–624.
- Bihmidine S, Julius BT, Dweikat I, et al. Tonoplast sugar transporters (SbTSTs) putatively control sucrose accumulation in sweet sorghum stems. Plant Signal Behav. 2016;11:e1117721. DOI: 10.1080/15592324.2015.1117721
- Cheng J, Wen S, Xiao S, et al. Overexpression of the tonoplast sugar transporter CmTST2 in melon fruit increases sugar accumulation. J Exp Bot. 2018;69:511–523.
- Cheng R, Cheng Y, Lü J, et al. The gene PbTMT4 from pear (Pyrus bretschneideri) mediates vacuolar sugar transport and strongly affects sugar accumulation in fruit. Physiol Plantarum. 2018;164:307–319.
- Li JM, Zheng DM, Li LT, et al. Genome-wide function, evolutionary characterization and expression analysis of sugar transporter family genes in pear (Pyrus bretschneideri Rehd). Plant Cell Physiol. 2015;56:1721–1737.
- Li Z, Zhang Z, Yan P, et al. RNA-Seq improves annotation of protein-coding genes in the cucumber genome. BMC Genomics. 2011;12:540. DOI: 10.1186/1471-2164-12-540
- Zhou Y, Hu L, Jiang L, et al. Genome-wide identification, characterization, and transcriptional analysis of the metacaspase gene family in cucumber (Cucumis sativus). Genome. 2018;61:187–194.
- Baloglu MC, Eldem V, Hajyzadeh M, et al. Genome-wide analysis of the bZIP transcription factors in cucumber. PLoS One. 2014;9:e96014. DOI: 10.1371/journal.pone.0096014
- Livak KJ, Schmittgen TD. Analysis of relative gene expression data using real-time quantitative PCR and the 2-ΔΔCT method. Methods. 2001;25:402–408.
- Wieczorke R, Krampe S, Weierstall T, et al. Concurrent knock-out of at least 20 transporter genes is required to block uptake of hexoses in Saccharomyces cerevisiae. FEBS Lett. 1999;464:123–128.
- Riesmeier JW, Willmitzer L, Frommer WB. Isolation and characterization of a sucrose carrier cDNA from spinach by functional expression in yeast. EMBO J. 1992;11:4705–4713.
- Li Y, Wang Y, Zhang H, et al. The plasma membrane-localized sucrose transporter IbSWEET10 contributes to the resistance of sweet potato to Fusarium oxysporum. Front Plant Sci. 2017;8:197. DOI: 10.3389/fpls.2017.00197
- Yan N. Structural advances for the major facilitator superfamily (MFS) transporters. Trends Biochem Sci. 2013;38:151–159.
- Cho JI, Burla B, Lee DW, et al. Expression analysis and functional characterization of the monosaccharide transporters, OsTMTs, involving vacuolar sugar transport in rice (Oryza sativa). New Phytol. 2010;186:657–668.
- Çakir, B, Giachino RRA, VvTMT2 encodes a putative tonoplast monosaccharide transporter expressed during grape berry (Vitis vinifera cv. Sultanine) ripening. Plant Omics. 2012;5:576–583.
- Pommerrenig B, Ludewig F, Cvetkovic J, et al. In concert: orchestrated changes in carbohydrate homeostasis are critical for plant abiotic stress tolerance. Plant Cell Physiol. 2018;59:1290–1299.
- Lemoine R, La Camera S, Atanassova R, et al. Source-to-sink transport of sugar and regulation by environmental factors. Front Plant Sci. 2013;4:272. DOI: 10.3389/fpls.2013.00272
- Eom JS, Chen LQ, Sosso D, et al. SWEETs, transporters for intracellular and intercellular sugar translocation. Curr Opin Plant Biol. 2015;25:53–62.
- Chandran D. Co-option of developmentally regulated plant SWEET transporters for pathogen nutrition and abiotic stress tolerance. IUBMB Life. 2015;67:461–471.
- Zeng L, Wang Z, Vainstein A, et al. Cloning, localization, and expression analysis of a new tonoplast monosaccharide transporter from Vitis vinifera L. J Plant Growth Regul. 2011;30:199–212.