Abstract
Recently, scientists are focused on the discovery of alternative sources of high-value products such as proteins, lipids and carbohydrates. Microalgal cells have great potential for accumulation of bioactive components important in food, feed, pharmaceutical and cosmetics industry, as sources of biofuel, for waste water remediation, etc. The main advantages of microalgae as producers of bioactive components are fast biomass growth; short reproduction cycle; resistance to harsh conditions and contaminations; smaller area for growing in comparison with agriculture crops, etc. However, the production of high-value components from microalgae biomass is still associated with high costs, so efforts are focused on cost reduction. This study evaluated the possibilities for production of low-cost high-value products from two microalgal strains, Chlorela vulgaris CAUP H1993 and Desmodesmus communis CAUP H522. Microalgal biomass was grown under simple low-cost media supplemented with urea as nitrogen source for extraction of crude protein. To completely utilize the harvested biomass, we applied two consecutive steps of the extraction procedure for protein and lipids accumulated in the exhausted biomass. The media supplemented with urea was promising, especially for growing C. vulgaris H1993 CAUP. The results showed that after two consecutive extraction steps, it is possible to obtain 8–9.0% crude protein and 21–24% lipids from the exhausted dry biomass of D. communis and C. vulgaris, respectively.
Introduction
Microalgae produce proteins, oils and carbohydrates as major intracellular compounds [Citation1–3], but it is important to find the optimal conditions for the production of components of interest. There are several large-scale production systems for microalgae, but they are associated with high costs. That is why the selection of cheap and promising media to improve microalgae production yield is of great interest [Citation4].
Nitrogen is one of the most important factors for optimal algal metabolism and growth. Most marine and freshwater microalgae can utilize nitrate or urea as nitrogen sources. Urea can serve as a nitrogen source in dinoflagellates and Chlamydomonas [Citation5–7]. Urea has a positive influence on the growth of Spirulina [Citation8], and a recent study reported cost-effective medium using urea as a nitrogen source for Arthrospira platensis [Citation9]. Among all organic nitrogenous sources, urea has gained greater importance generally in large-scale algal cultivation, because urea has lower cost than other alternative nitrogen sources [Citation10,Citation11]. The price of urea is lower than the price of KNO3, since both were widely used as supplement in the artificial media for cultivation of algae [Citation12,Citation13]. The choice of nitrogen source and growth media is important for the biochemical composition of the microalgal cells: chlorophylls, proteins, carbohydrates and lipids with implications for their nutritional value [Citation14–16].
Another way to reduce the cost is by co-extraction of more than one bioactive component, which gives the possibility for complete utilization of the harvested algal biomass. Therefore, Kulkarni and Nikolov [Citation17] suggested a co-extraction procedure for evaluation of the protein and carotenoid content of C. vulgaris.
The green alga Chlorella vulgaris is a unicellular microalgal species with a rapid growth rate. It is resistant to harsh conditions and contamination, and has a variety of highly value-added components. The results from the comparison of its protein content and quality with reference food proteins – eggs, soya and meat, recommended by World Health Organization [Citation18] and Food and Agriculture Organization have been highly favourable [Citation19]. D. communis is another microalgal species of a great potential for high protein accumulation, with good proportion of indicators related to protein bioavailability (high biological value, digestibility coefficient, net protein utilization and protein efficiency ratio) [Citation20]. Therefore, the aim of this study was (i) to find the fast-growing microalgae strain able to grow in urea supplemented medium, and (ii) to achieve complete utilization of the harvested algae biomass. A procedure for extraction of the two main groups of components – proteins and lipids, from a small batch of algae biomass by two consecutive steps is suggested.
Materials and methods
Strains and growth conditions
Thirteen different Chlorella and Scenedesmus s.l. strains from the collections of Charles University in Prague (CAUP) and University of Gdansk (CCBA) were grown under conditions described in . In preliminary experiments, the growth conditions shown in variant 2 were chosen as more suitable and economically appropriate. All experiments were conducted under these conditions. The strains C. vulgaris H1993 and D. communis H522, from the CAUP collection showed fast growth in Bold’s Basal medium (BBM) and were selected for the subsequent experiments. The strains were grown in medium supplemented with 118.5 mg/L KH2PO4; 55.0 mg/L CaCL2 × 2H2O; 109.0 mg/L MgSO4 × 7H2O and 550 mg/L (9 mmol/L) Co(NH2)2 [12]. The biomass growth was compared in BBM [Citation21]. Biomass accumulation was monitored by optical density measurement at 595 nm (EZ Read 400 Microplate Reader Biochrom) and microscopically at 40x magnification (Zeiss Imager.A2, camera Axio CamERc 5s). Biomass was harvested at stationary phase, washed with distillated water, freeze dried at −50 °C and 0.51 mbar (Christ GmbH) and stored at −80 °C for further analysis. Biomass dry weight was measured according to Takeshita et al. [Citation22].
Table 1. Growth conditions tested for biomass accumulation of C. vulgaris H1993, CAUP and D. communis H522, CAUP.
Data analysis
All experiments were conducted in triplicate. The results were statistically analysed using one-way analysis of variance (ANOVA) (MS Office, 2010).
Cell disruption methods and crude protein recovery
Three different methods for cell disruption were tested: sonication for 10 min (5 min on/5 min off), autolysis according to Kightlinger et al. [Citation23] and a combination of freeze-drying followed by bead milling with 3 mm stainless steel beads in a TissueLyser (Retsch, Qiagen). Dry biomass was subjected to a food friendly protein extraction protocol according to Meijer and Wijffels [Citation24] with some modifications: the extraction buffer (25 mmol/L phosphate buffer, pH = 7.0) was used without detergents. The extracted crude protein content was measured by Bradford assay [Citation25].
Co-extraction of exhausted biomass
The fatty acids were extracted from exhausted dry biomass after crude protein recovery from both strains C. vulgaris H1993 and D. communis H522 according to Moyankova et al. [Citation26] with the following modifications: 0.03 mg dry biomass was homogenized with 1.0 mL methanol. As internal standards, 40.0 µg/mL nonadecanoc acid methyl ester and 20.0 µg/mL ribitol were added. The samples were incubated for 30 min at 70 °C, centrifuged at 6000g/10 min, and 2.0 mL chloroform was added to the supernatant. After vortexing for 5 min, 500.0 µL of water were added. The polar and apolar phasess were collected separately and evaporated in a Centrivap Concentrator (Labconco). The apolar phase was transesterificated with 1.0 mL 2% H2SO4, incubated at 90 °C for 50 min, and 1.0 mL n-hexane was added after cooling. The sample was homogenized and the upper phase passed trought anhydrous Na2SO4. The collected samples were dried (under vacuum). The samples were desolved with 50.0 µL pyridine and derivatized with BSTFA at 70 °C for 45 min. After cooling, 100.0 µL chloroform were added and the samples were analysed by gas chromatography-mass spectrometry (GC-MS).
GC-MS analysis was carried out on a 7890 A gas chromatograph (Agilent Technologies) interfaced with a 5975C mass selective detector (Agilent Technologies). Separations were performed using a 30 m × 0.25 mm (i.d.) DB-5 ms silica-fused capillary column coated with 0.25 µm film of poly(dimethylsiloxane) as the stationary phase. The flow rate of the carrier gas (helium) was maintained at 1.0 mL/min. The injector and the transfer line temperature were kept at 250 °C. The oven temperature program used was 100 °C for 2 min, then 15 °C/min to 180 °C for 1 min, then 5 °C/min to 300 °C for 10 min. The injection volume was 1 µL. The injections were carried out in a split mode 10:1. The mass spectrometer was scanned from 50 to 550 m/z. All mass spectra were acquired in electron impact mode with 70 eV. A mixture of aliphatic hydrocarbons (C8–C40) (Sigma) was injected into the system under the above temperature programme in order to calculate the retention index RI (as Kovàts index) of each compound. Compounds were identified by comparing the RI and the spectral data from NIST’08 (National Institute of Standards and Technology, USA).
All chemicals for lipid extraction were purchased from Sigma-Aldrich company.
Results and discussion
Microalgal growth under urea supplemented medium
The growth of both strains, C. vulgaris (CAUP H1993) and D. communis (CAUP H522), in BBM and urea medium was recorded (). The results showed that the medium supplemented with urea was more suitable for C. vulgaris H1995 than for D. communis H522, confirmed by all growth conditions tested (data now shown). The strain D. communis grew better in BBM supplemented with all macro- and micronutrients. The exponential growth phase of C. vulgaris H1993 in urea medium started from day 7 and continued until the end of our experiment, at day 35, when the increase in biomass was 7-fold in urea supplemented medium vs. 5-fold in BBM medium (). D. communis increased its biomass 4-fold at the end of our experiment in BBM medium and nearly 3-fold in urea supplemented medium. The cultures of both strains in urea medium and BBM, with pictures taken on day 15, are demonstrated in . The cell morphology, monitored microscopically (), showed that in urea medium, the cells of D. communis became larger. Many dead cells were also visible in comparison with C. vulgaris. Both effects became stronger in the course of time ().
Figure 1. Dynamics of biomass growth of C. vulgaris H1993 and D. communis H552 in urea supplemented medium and Bold’s Basal medium (BBM).
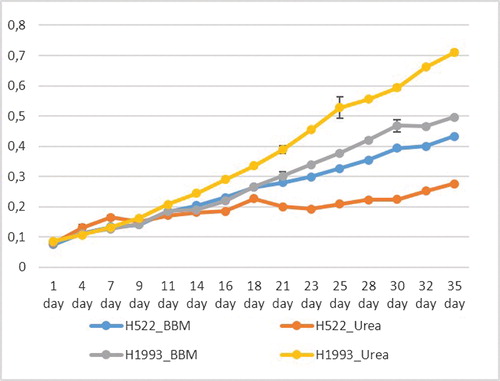
Cell disruption methods and crude protein recovery
The algal cells were harvested at the stationary phase and used for crude protein recovery. For optimal protein extraction, three different methods for cell disruption were applied: sonication, autolysis and a combination of freeze-drying followed by beads milling. Freeze-drying followed by beads milling gave better results, so this technique was used in all subsequent experiments. The biomass yield and crude protein content of C. vulgaris H1993 and D. communis H522 grown in urea supplemented medium were higher than those obtained in BBM, indicating that urea medium provided better substrate for the growth of the C. vulgaris strain than for D. communis (). As a result, higher biomass and protein content were produced from Chlorella strain H1993 ().
The protein content obtained from the cultures grown in urea supplemented medium was similar to the data reported by Safi et al. [Citation27] after ultrasonocation pretreatment of C. vulgaris cells. The authors reported also 15% protein content after chemical pretreatment of algal cells from the same strain, which was not applied in our experiments because the severe extraction conditions (low pH = 3 and application of NaOH and HCI) would require additional costs for protein extract purification. A similar total protein content was obtained by application of a high pressure homogenization system [Citation17]. On the other hand, it noteworthy that, in general, the protein extraction from Chlorella cells is associated with the inconvenience of the low intracellular components availability due to the rigid cell wall of this alga [Citation28]. To our knowledge, most published data on the concentration of algal proteins were obtained based on calculation of the total nitrogen content after hydrolysis of the algal biomass. This, in our opinion, could result in overestimation of the true protein content, since other algal cell components, such as nucleic acids, amines and glucosamides, or cell walls also contain nitrogen [Citation20]. For example, the non-protein nitrogen amount was 12% in Scenedesmus obliquus, 11.5% in Spirulina and 6% in Dunaliella [Citation20].
Future studies need to focus on further optimization of the cell disruption procedure and the medium used. For example, the phosphate content could also affect the protein accumulation [Citation29] (as cited in [Citation4]). The author showed that Chlorella sp., cultured in a medium containing 0.25 mg/L phosphate, had 15.7% protein content, while with a higher phosphate concentration of 0.50 mg/L, it had 37% protein content per dry weight. We believe that an increase in the phosphate concentration in the culture medium would not considerably increase the cost of algal growth.
Free fatty acids recovery from exhausted biomass
Free fatty acids from the exhausted dry biomass harvested after protein extraction were recovered from the non-polar phase collected during the lipid extraction procedure. After protein extraction, it was possible to obtain about 35 mg free fatty acids per liter culture of D. communis H522 exhausted biomass. A similar amount was obtained from the control sample – unexhausted biomass (). The amount of total lipids recovered from C. vulgaris H1993 exhausted biomass was 34.0 mg/L versus 37.5 mg/L from the control sample ().
Table 2. Free fatty acids obtained from non-exhausted biomass and from exhausted biomass collected after total protein extraction from D. communis H522 and C. vulgaris H1993.
The lipid profile of the control sample of C. vulgaris H1993 showed that palmitic acid (C 16:0) ranked first with 42.0% of the total lipid content, followed by stearic acid (C 18:0) with 26.9%. The next positions were taken by polyunsaturated fatty acids: linolenic acid with 12.8%, oleic acid with 9.1% and linoleic acid with 4.12% of the total lipid content. In the lipid profile of D. communis H522, palmitic acid (C 16:0) was 30.7%, linolenic and stearic acid were presented with similar amounts of 20.7% and 18.9%, respectively, and linoleic acid and oleic acid comprised, respectively, 8.3% and 7.3% of the total lipid content ().
The ratio of polysaturated to polyunsaturated fatty acids was 68.80:26.0% in the lipid profile of D. communis. This ratio was 49.5%:36.2% in the profile of C. vulgaris.
The method for total lipid extraction applied here is similar to the classical procedure of Folch et al. [Citation30] (as cited in [Citation31]). The authors reported a similar total lipid content from C. vulgaris (19.9%), and 29.7% from S. obliquus. According to Minhas et al. [Citation32], the lipid content of C. vulgaris was 24.97% of the dry weight. Overall, we could suggest that the procedure suggested here was more effective for free fatty acids recovery than for total protein recovery from both studied algal strains.
Conclusions
The obtained results suggest that the microalgal strain C. vulgaris H1993 CAUP is of industrial interest, as it can utilize nitrogen from low-cost urea supplemented medium. Following the proposed co-extraction procedure, two high-value products, i.e. crude proteins and lipids, can be consecutively obtained from a small batch of culture volume. We recommend further testing the two strains studied by us, C. vulgaris and D. communis, for potential purification of organic waste water containing urea.
Acknowledgements
This work was supported by the America for Bulgaria Foundation under Grant AGR.0050.20160121.
Disclosure statement
No potential conflict of interest was reported by the authors.
References
- John R, Anisha G, Nampoothiri K, et al. Micro and macroalgal biomass: a renewable source for bioethanol. Bioresour Technol. 2011;102:186–193.
- Perez-Garcia O, Escalante FME, de-Bashan LE, et al. Heterotrophic cultures of microalgae: metabolism and potential products. Water Res. 2011;45:11–36.
- Choix F, De-Bashan L, Bashan Y. Enhanced accumulation of starch and total carbohydrates in alginate-immobilized Chlorella spp. induced by Azospirillum brasilense: II. Heterotrophic. Enzyme Microb Technol. 2012;51:294–299.
- Chia MA, Lombardi AT, Melão MDGG. Growth and biochemical composition of Chlorella vulgaris in different growth media. An Acad Bras Cienc. 2013;85:1427–1438.
- Healey FP. Inorganic nutrient uptake and deficiency in algae. CRC Crit Rev Microbiol. 1973;3:69–113.
- Syrett PJ. Uptake and utilization of nitrogen compounds. In: Rogers LJ, Gallon JR, editors. Proceedings of the biochemistry of the algae and cyanobacteria. Oxford. Clarendon Press; 1988. p. 23–39.
- Solomon CM, Glibert PM. Urease activity in five phytoplankton species. Aquat Microb Ecol. 2008;52:149–157.
- Affan MA, Lee DW, Al-Harbi SM, et al. Variation of Spirulina maxima biomass production in different depths of urea-used culture medium. Braz J Microbiol. 2015;46:991–1000.
- Puganeswary S, Rosimah N, Normala H, et al. formulation of cost-effective medium using urea as a nitrogen source for Arthrospira platensis cultivation under real environment. Ann Res Rev Biol. 2018;22:1–12.
- Danesi EDG, Rangel-Yagui CO, Carvalho JCM, et al. An investigation of effect of replacing nitrate by urea in the growth and production of chlorophyll by Spirulina platensis. Biomass Bioenerg. 2002;23:261–269.
- Matsudo MC, Bezerra RP, Sato S, et al. Repeated fed-batch cultivation of Arthrospira (Spirulina) platensis using urea as nitrogen source. Biochem Eng J. 2009;43:52–57.
- Crofcheck CL, E X, Shea A, et al. Influence of media composition on the growth rate of Chlorella vulgaris and Scenedesmus acutus utilized for CO2 mitigation. J Biochem Technol. 2012;4:589–594.
- Kong W, Song H, Cao Y, et al. The characteristics of biomass production, lipid accumulation and chlorophyll biosynthesis of Chlorella vulgaris under mixotrophic cultivation. Afr J Biotechnol. 2011;10:11620–11630.
- Griffiths MJ, Harrison STL. Lipid productivity as a key characteristic for choosing algal species for biodiesel production. J Appl Phycol. 2009;21:493–507.
- Pittman JK, Dean AP, Osundeko O. The potential of sustainable algal biofuel production using wastewater resources. Biores Technol. 2011;102:17–25.
- Fabregas J, Muñoz A, Llovo J, et al. Differentiation of Candida guilliermondii varieties by lectin-like substances from marine algae. Res Microbiol. 1989;140:373–378.
- Kulkarni S, Nikolov Z. Selective extraction of carotenoids and proteins from Chlorella vulgaris. An ASABE Meeting Presentation; 2017. Paper Number 1701229. Available from:: https://doi.org/10.13031/aim.201701229
- WHO. Protein and amino acid requirements in human nutrition. Geneva: World Health Organisation; 2007; 284.
- FAO. A Review on culture, production and use of Spirulina as food for humans and feeds for domestic animals and fish. Rome: Food and Agriculture Organization of the United Nations. 2008; 20:
- Becker EW. Micro-algae as a source of protein. Biotechnol Adv. 2007;25:207–210.
- Andersen RA. Algal Culturing Techniques. Burlington (NJ): Elsevier Academic Press; 2005.
- Takeshita т, Ota S, Yamazaki T, et al. Starch and lipid accumulation in eight strains of six Chlorella species under comparatively high light intensity and aeration culture conditions. Biores Technol. 2014;158:127–134.
- Kightlinger W, Chen K, Pourmir A, et al. Production and characterization of algae extract from Chlamydomonas reinhardtii. Electron J Biotechnol. 2014;17:14–18.
- Meijer FA, Wijffels RH. Development of a fast reproducible and effective method for the extraction and quantification of proteins of micro-algae. Biotechnol Tech. 1998;12:353–358.
- Bradford MM. A rapid and sensitive method for the quantitation of microgram quantities of protein utilizing the principle of protein-dye binding. Anal Biochem. 1976;72:248–254.
- Moyankova D, Mladenov P, Berkov S, et al. Metabolic profiling of the resurrection plant Haberlea rhodopensis during desiccation and recovery. Physiol Plant. 2014;152:675–687.
- Safi C, Ursu AV, Laroche C, et al. Aqueous extraction of proteins from microalgae: effect of different cell disruption methods. Algal Res. 2014;3:61–65.
- Ursu A-V, Marcati A, Sayd T, et al. Extraction, fractionation and functional properties of proteins from the microalgae Chlorella vulgaris. Biores Technol. 2014;157:134–139.
- Mahasneh IA. Production of single cell protein from five strains of the microalga Chlorella spp. (Chlorophyta) ). Cytobios. 1997;90:153–161.
- Folch J, Lees M, Stanley GHS. A simple method for the isolation and purification of total lipids from animal tissues. J Biol Chem. 1957;226:497–509.
- Ryckebosch E, Muylaert K, Foubert I. Optimization of an analytical procedure for extraction of lipids from microalgae. J Am Oil Chem Soc. 2012;89:189–198.
- Minhas AK, Hodgson P, Barrow CJ, et al. The isolation and identification of new microalgal strains producing oil and carotenoid simultaneously with biofuel potential. Biores Technol. 2016;211:556–565.