Abstract
Genetic diversity studies of endangered plants help to understand the endangering causes and evolutionary history of species. To provide reliable data on the protection of the endangered species Glehnia littoralis, we assessed its genetic diversity and genetic structure based on amplified fragment length polymorphism (AFLP). A total of 127 individuals from 9 wild populations were detected by 10 pairs of AFLP primers. The results showed high levels of genetic diversity in G. littoralis. Ten primer combinations produced a total of 1929 DNA bands with a polymorphic frequency of 100%. The overall gene diversity (Ht) was 0.182, average gene diversity within populations (Hw) was 0.168, Nei’s genetic diversity (Hj) of different populations ranged from 0.142 (Zh15) to 0.1908 (Gld15), and Shannon’s information index (I) was 0.238. The analysis of molecular variance (AMOVA) revealed that the variation within populations accounted for 84.2% of the total variation, indicating the differentiation mainly existed within populations. The gene flow (Nm) among populations was 2.665, revealing more gene communication between populations. The UPGMA cluster analysis, the principal coordinate analysis (PCoA), and the result of mantel test (r = −0.0898, p = 0.259) all showed that there was no obvious correlation between genetic distance and geographical distribution. In conclusion, we speculate that the endangered status of this species could be attributed to the destruction of wildlife habitats rather than to the loss of genetic diversity. Therefore, we suggest strengthening the germplasm resources conservation and doing ecological conservation and restoration work to protect the wild populations of G. littoralis.
Introduction
The species Glehnia littoralis Fr. Schmidt ex Miq, belonging to the family Apiaceae, is a perennial diploid (2n = 22) herb usually growing on sandy seasides [Citation1,Citation2]. This habitat is favourable for initial easy root and later rhizome development, which makes this species an economically important tool for preventing sand erosion. In addition, in China, the roots of G. littoralis called Beishashen are used widely as an important traditional medicine for a relatively strong inhibitory effect against immune-related diseases [Citation3]. However, in the past decades, the wild populations of G. littoralis have underwent large-scale reduction because of harvesting activities and beach destruction. As a result, G. littoralis was listed in the Endangered Plants Chinese Chronicles, and was even called plant Panda in China [Citation4]. Due to its endangered condition, G. littoralis has attracted the attention of many researchers, and some research has been conducted, including germination of seeds, genetic diversity analysis, etc. [Citation5–11].
Genetic diversity of endangered plants has been considered to be helpful to understand the evolutionary history of species, and then to formulate targeted conservation strategies of endangered species [Citation11,Citation12]. Genetic diversity studies are currently supported by molecular methods, such as molecular markers and gene sequence analysis. In this study, amplified fragment length polymorphism (AFLP) markers [Citation13] were chosen as a very reliable molecular method [Citation14–16] to assess the genetic diversity among different populations of G. littoralis. AFLP has been proved as a powerful marker for accurate estimation of the genetic structures of plant populations, with high reproducibility [Citation17–19]. Based on this molecular marker, we aimed to investigate the main factors (e.g. breeding system, habitat fragmentation, geographic distribution, gene flow and genetic drift, etc.) that have affected the genetic variation among and within populations, and provide some useful references for the protection policy.
Materials and methods
Plant material
In this study, a total of 127 individual plants of 9 populations of G. littoralis Fr. Schmidt ex Miq from China were examined (). All samples were collected from natural populations and fresh leaf tissues were dried rapidly in the field using silica gel. The origins of each population are listed in . Voucher specimens of G. littoralis are deposited in the herbaria of the Department of Plant and Ecology, School of Life and Sciences, Ludong University.
Figure 1. Distribution and sampling sites for nine populations of Glehnia littoralis. Source: https://map.baidu.com/search/%E5%85%A8%E5%9B%BD/@12959219.6,4825334.63,5z?querytype=sda_src=shareurl&wd=%E4%B8%AD%E5%9B%BD&c=236&src=0&pn=0&sug=0&l=15&b=(13430840,432417;13441080,4328521)&from=webmap&biz_forward=%7B%22scaler%22:1,%22styles%22:%22pl%227D&device_ratio=1
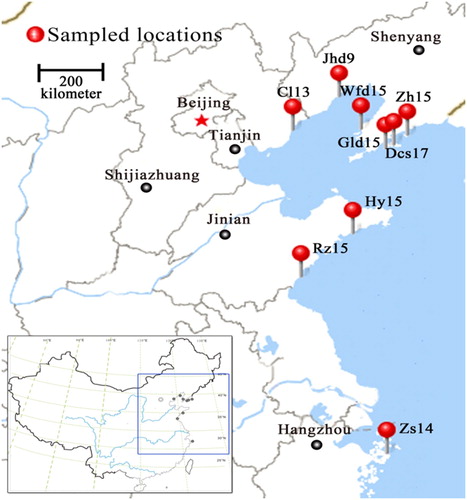
Table 1. Origin of sampling populations.
AFLP analysis
Genomic DNA was extracted from silica-gel–dried leaf tissue using a modified CTAB (cetyltrimethylammonium bromide) method [Citation20]. The DNA concentration in the samples was determined by gel electrophoresis and was adjusted to 50 ng/μL for polymerase chain reactions (PCRs). The AFLP program was performed by Beijing Dingguo Changsheng Biotechnology Co. (BDCB) according to the protocol of Vos et al. [Citation13] with minor modifications. Genomic DNA was digested using the restriction enzymes HindIII (New England Biolabs Ltd, NEB) and MseI (NEB). DNA fragments were linked with HindIII and MseI adapters using T4 Ligase (NEB) to generate template DNA for PCR amplification.
Polymerase chain reaction consisted of two consecutive reactions: the pre-amplification and the selective amplification. In the pre-amplification step, genomic DNA was amplified with AFLP pre-amplification primers, Hind-A (5′-AGACTGCGTACCAGCTTA-3′) and Mse-C (5′-GATGAGTCCTGAGTAAC-3′), each having one selective nucleotide. The PCR products were diluted 1:20 with distilled deionized water and were used as a template for the selective amplification. Ten selective primer pairs with three selective nucleotides were chosen for the selective PCR amplification to detect polymorphisms among nine populations of G. littoralis. The primer sequences are shown in .
Table 2. AFLP primer sequences, number of loci and number of polymorphic loci.
The selective PCR reaction was performed in a 25-μL reaction mixture, containing 17.5 μL sterile double-distilled water, 2.5 μL of 10× reaction buffer, 0.5 μL of 10 mmol/L dNTP mixtures (BDCB), 1 μL of each primer (5 pm) (BDCB), 2 units of Taq DNA polymerase (BDCB) and 2 μL of DNA template (50 ng/μL). The program began with a touch-down cycle by 13 cycles of 94 °C for 30 s, 65 °C for 30 s (decrease of 0.7 °C per cycle) and 72 °C for 80 s; and then 23 cycles of 94 °C for 30 s, 55 °C for 30 s, and 72 °C for 80 s, plus a final elongation step of 72 °C for 10 min.
Polymerase chain reaction products were examined for capillary electrophoresis by an ABI377 DNA Analyzer. Bands between 70 and 500 bp were scored using GENESCAN 3.1.2 analysis software (Perkin Elmer Applied Biosystems).
Data analysis
All bands were treated as a locus ranging from 70 to 500 bp. The data were transformed into a binary character (0, 1) matrix for further data analyses, value 1 for presence of the band and 0 for absence. Several genetic diversity related parameters, Hj (Nei’s gene diversity) [Citation21], Ht (the total gene diversity) and Hw (the average gene diversity within populations), were computed by the software AFLP-SURV v.1.0 [Citation22]. The total number of bands, the number of polymorphic loci, percentage of polymorphic loci (%), observed number of alleles per loci (Na), effective number of alleles per locus (Ne) [Citation23], Shannon’s information index (I) [Citation24] and analysis of molecular variance (AMOVA, Gst) [Citation25], were calculated using the software GenAlEx 6.5 [Citation26, Citation27]. The gene flow (Nm) between populations was estimated using the equation Nm = 0.5(1−Gst)/Gst. Cluster analysis of populations was conducted to construct the dendrogram using UPGMA (unweighted pair group method with arithmetic mean) based on Nei’s genetic identity [Citation28] and the software NTSYS-pc ver. 2.10 [Citation29]. Principal coordinate analysis (PCoA) of all individuals was carried out using the software GenAlEx 6.5 [Citation26,Citation27]. To estimate the correlation between genetic and geographical distances, the Mantel test was performed using TFPGA [Citation30].
Results and discussion
Polymorphic analysis
Ten selective AFLP primer combinations produced a total of 1929 DNA bands from 127 representative samples of 9 populations of G. littoralis. The number of bands per primer ranged from 178 to 207 with a polymorphic frequency of 100% (). This result was in line with that obtained by SRAP (sequence-related amplified polymorphism) analysis (100%) and was higher than those reported by RAPD (random amplified polymorphic DNA, 82.28%) and ISSR (inter-simple sequence repeat, 84.21%) analyses [Citation9–11], indicating that AFLP markers are useful in investigating the genetic diversity of G. littoralis populations. The number of loci of 9 populations ranged from 918 to 1343, and the polymorphic frequency was from 45.31% to 69.41%. The maximum value existed in population Gld15 and the minimum value, in Jhd9 (). All these results indicated that high polymorphism existed in the populations of G. littoralis.
Table 3. Genetic diversity parameters of nine Glehnia littoralis populations.
Genetic diversity and genetic structure
The genetic diversity analysis showed that the overall gene diversity (Ht) was 0.182, the average gene diversity within populations (Hw) was 0.168, Nei’s genetic diversity (Hj) of different populations ranged from 0.142 (Zh15) to 0.1908 (Gld15) and Shannon's information index (I) was 0.238 (). Compared with previous results based on RAPD (Ht = 0.51, I = 0.56), ISSR (H = 0.3425, I = 0.8663) and SRAP (Ht = 0.2471, I = 0.5516) analyses [Citation9–11], the parameter values were relatively low. However, comparing with other endangered species, the populations of G. littoralis revealed a relatively high genetic diversity. For example other studies of endangered officinal plants based on AFLP analysis have been reported: He= 0.148, I = 0.220 for Dendrobium officinale [Citation31] and He = 0.143, I = 0.221 for Ranunculus cabrerensis [Citation32]. In view of this, we can consider that the results of this study were also consistent with those of previous studies in G. littoralis based on ISSR, RAPD and SRAP analyses [Citation9–11].
On the basis of AMOVA, the variation within populations accounted for 84.2% of the total variation, while among population variation contributed for only 15.8%, indicating that the differentiation mainly existed within populations. The gene flow (Nm) among the populations was 2.665, revealing more genetic communication between populations.
The factors affecting the genetic structure of a species include the breeding system, the distribution and the gene flow, etc. [Citation33,Citation34]. The species of G. littoralis is a perennial herb (2n = 22), mainly reproducing sexually via flowers by insect-pollination and wind-pollination, its seeds being propagated by wind or water [Citation6]. Long-distance propagation of pollen by wind and insects may exist and the growing environment of G. littoralis is mainly at the seaside. The connectivity of ocean waters might make the seeds of G. littoralis spread over a long distance by the water, both promoting gene exchange and enriching the gene pool in populations. This could have lead to an increase in the within-population genetic diversity of species and a reduction in the genetic differentiation between populations. The relatively high gene flow (Nm = 2.665) supports this view.
Cluster analysis
The dendrogram () based on Nei’s genetic identity revealed two supported groups. The first group included Hy15, Cl13, Gld15 and Dcs17, in which, Hy15 and Cl13 clustered as a subgroup, whereas Gld15 and Dcs17 clustered as another subgroup. The second group consisted of Wfd15, Zh15, Zs14, Rz15 and Jhd9, in which Wfd15 and Zh15 grouped into one subgroup, and Zs14, Rz15 and Jhd9 grouped into another subgroup.
Figure 2. Phylogenetic dendrogram based on Nei’s genetic identity of nine Glehnia littoralis populations.
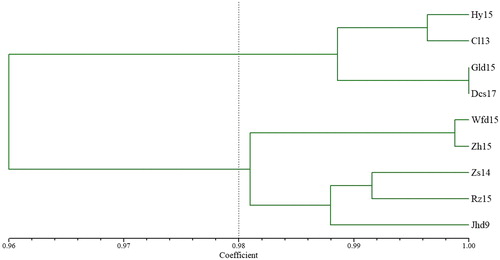
The PCoA based on the genetic distance matrix also revealed two main groups (). The first group included two subclades: the individuals of Hy15 and Cl13 gathered together, and those of Gld15 and Dcs17 constituted a cluster (). All individuals of Zh15 and Wfd15 clustered into one subclade, most individuals of Zs14 and all individuals of Rz15 and Jhd9 clustered into another subclade (); two subclades gathered into the second group (). The three principal vectors revealed 10.70%, 3.24% and 2.70% of genetic variation, respectively.
Figure 3. Principal coordinate analysis revealing clustering pattern of 127 Glehnia littoralis individuals: (a) principal coordinates (1 vs 2); (b) principal coordinates (1 vs 3).
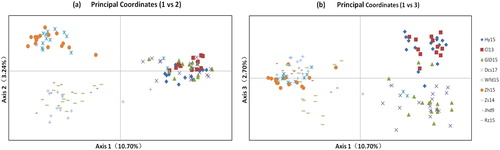
The above cluster analysis results were consistent with previous reports [Citation9–11], also showing a clear and similar topological structure: nine populations of G. littoralis were separated into two main groups and four subgroups ( and ). In them, Hy15 and Cl13, and Zs14 and Rz15, clustered as a subgroup respectively, in agreement with studies based on three other molecular markers, ISSR, RAPD and SRAP [Citation9–11]. Gld15 and Dcs17 clustered as one subgroup, in support of previous studies based on ISSR and RAPD [Citation9,Citation10]. Wfd15 and Zh15 grouped into one subgroup, supporting previous studies based on ISSR and SRAP molecular markers [Citation9, Citation11].
The Mantel test result with 1000 permutations suggested that the genetic divergence of the populations (Nei’s genetic distance) was not significantly correlated with the geographic distance (r = −0.0898, p = 0.259). Some geographically distant populations belonged to the same group, while some geographically closer groups did not cluster together, suggesting that their genetic distance is not necessarily related to geographical distances. For example, the genetic distance of population Cl13 and Jhd9 was the greatest (0.056), but the two populations were adjacent and their geographical distance was relatively small (); whereas five populations in Liaoning Province within a short geographical distance were divided into two groups.
One of the possible reasons is that G. littoralis grows on the seaside. The coastal ecological environment is relatively stable, therefore, the effect of habitat changes due to geographical distance is small, which reduces the influence of environmental factors on the genetic structure of the population. On the other hand, the long-distance transfer of seeds by water increases the gene flow and genetic exchange among different populations, thus reducing the differentiation of individuals between populations. Excluding the deviation of sampling, it is inferred that, in a certain spatial scale, the geographical distance has had little influence on the genetic structure of the studied populations, but the breeding system of G. littoralis played a major role in influencing the genetic structure of the populations.
Protection strategies
The primary task in the protection of endangered species is to maintain their genetic diversity [Citation35,Citation36]. Our study based on AFLP analysis reconfirmed the high genetic diversity in populations of G. littoralis, which is the genetic basis for a species to adapt to a changing environment. The main reason for the endangered status of this species may be the destruction of wildlife habitats rather than the loss of genetic diversity. Therefore, it is necessary to strengthen the germplasm resources conservation of G. littoralis, and the populations with high level of genetic diversity should be protected in situ. At the same time, in consideration of the existence of rare genes in low level regions, ecological conservation and restoration work should be carried out in areas with a small number of individuals and serious habitat destruction, through artificial intervention and other measures to restore the size of wild population. In addition, studies on the physiology, ecology, pollination biology, etc., should also be carried out effectively to provide more data for the protection of G. littoralis.
Conclusions
A total of 127 individuals from nine wild populations of G. littoralis were collected to assess its genetic diversity and genetic structure based on AFLP. The results showed a higher level of genetic diversity in this species than in other endangered wild medicinal plants, which was due to strong variation within its populations (84.2%). In view of this, we speculated that the endangered status of G. littoralis could mainly be attributed to habitat destruction rather than to loss of genetic diversity, and proposed some targeted protection strategies. These results will be useful for future studies of the germplasm resources of G. littoralis.
Disclosure statement
No potential conflict of interest was reported by the authors.
Funding
This work was supported by the Natural Science Foundation of Shandong Province under grant number ZR2010CM056 and the National Science Foundation of China under grant number 31000104.
References
- Mathias ME. Studies in the Umbelliferae. I Ann Mo Bot Gard. 1928;15:91–109.
- Shan RH, She ML. Flora of China. Vol. 55. Beijing: Science Press; 1992. p. 77.
- Yoon T, Cheon MS, Lee AY, et al. Anti-inflammatory activity of methylene chloride fraction from Glehnia littoralis extract via suppression of NF-kappa B and mitogen-activated protein kinase activity. J Pharmacol Sci. 2010;112:46–55.
- Fu LG. Red data book of plants in China. Vol. 1. Beijing: Science Press; 1992. p. 698.
- Hui H, Liu QX, Liu MH. Allozyme variation and genetic diversity of Glehnia littoralis populations at the middle of seaboard in China. J Plant Resour Environ. 2001;10:1–6.
- Huh M, Choi J, Huh H, et al. Genetic diversity and population structure of Glehnia littoralis (Umbelliferae) in Korea. Korean Orient Phys Pathol. 2003;17:1519–1523.
- Liu QX, Hui H, Liu MH. Determination of seed vitality and germination rate of the endangered plant Glehnia littoralis. J Plant Resour Environ. 2004;13:55–56.
- Song CF, Liu QX, Zhou YF, et al. Genetic diversity analysis of Glehnia littoralis (Apiaceae) revealed by SRAP. Guihaia. 2014;34:15–18.
- Wang AL, Wang GL, Li WW. Genetic diversity of Glehnia littoralis populations revealed by ISSR molecular markers. Acta Bot Boreal Occident Sin. 2015;35:1541–1546.
- Wang AL, Li WW, Liu X. Genetic diversity analysis of Glehnia littoralis populations, an endangered medicinal plant, based on RAPD molecular markers. J Anhui Agr Univ. 2015;42:792–796.
- Wang AL, Zhang P, Liu X, et al. Genetic structure and diversity of Glehnia littoralis, an endangered medicinal plant in China. Biochem Syst Ecol. 2016;66:265–271.
- Brekke P, Bennett PM, Santure AW, et al. High genetic diversity in the remnant island population of hihi and the genetic consequences of re-introduction. Mol Ecol. 2011;20:29–45.
- Vos P, Hogers R, Bleeker M, et al. AFLP: a new technique for DNA fingerprinting. Nucleic Acids Res. 1995;23:4407–4414.
- Tohme J, Gonzalez DO, Beebe S, et al. AFLP analysis of gene pools of a wild bean core collection. Crop Sci. 1996;36:1375–1384.
- Meudt HM, Clarke AC. Almost forgotten or latest practice? AFLP applications, analyses and advances. Trends Plant Sci. 2007;12:106–117.
- Mohapatra KP, Sehgal RN, Sharma RK, et al. Genetic analysis and conservation of endangered medicinal tree species Taxus wallichiana in the Himalayan region. New Forest. 2009;37:109–121.
- Nag A, Ahuja PS, Sharma RK. Genetic diversity of high-elevation populations of an endangered medicinal plant. AoB Plants. 2014;7:1–15.
- Jian HY, Li SF, Guo JL, et al. High genetic diversity and differentiation of an extremely narrowly distributed and critically endangered decaploid rose (Rosa praelucens): implications for its conservation. Conserv Genet. 2018;9:761–776.
- Zhang CL, Sun M, Zhang XQ, et al. AFLP-based genetic diversity of wild orchardgrass germplasm collections from central Asia and western China, and the relation to environmental factors. PLoS ONE. 2018;13:e0195273.
- Doyle JJ, Doyle JL. A rapid DNA isolation procedure for small quantities of fresh leaf material. Phytochem Bull. 1987;19:11–15.
- Nei M. Analysis of gene diversity in subdivided populations. Proc Natl Acad Sci U S A. 1973;70:3321–3323.
- Vekemans X. Aflp-Surv Version 1.0. Distributed by the Author. Belgium: Laboratoire De Génétique Et Ecologie Végétale, Université Libre De Bruxelles; 2002.
- Kimura M, Crow JF. The number of alleles that can be maintained in a finite population. Genetics. 1964;69:725–738.
- Lewontin RC. The apportionment of human diversity. Evol Biol. 1972;6:1–398.
- Excoffier L, Laval G, Schneider S. Arlequin (version 3.0): an integrated software package for population genetics data analysis. Evol Bioinform. 2005;1:47–50.
- Peakall ROD, Smouse PE. GenAlEx 6: genetic analysis in Excel. Population genetic software for teaching and research. Mol Ecol Notes. 2006;6:288–295.
- Peakall R, Smouse PE. GenAlEx 6.5: genetic analysis in Excel. Population genetic software for teaching and research-an update. Bioinformatics. 2012;28:2537–2539.
- Nei M. Genetic distance between populations. Am Nat. 1972;106:283–292.
- Rohlf FJ. NTSYSpc: numerical taxonomy and multivariate analysis system. Version 2.10. New York, NY: Exeter Software; 2000.
- Miller MP. Tools for population genetic analysis (TFPGA) version 1.3: a windows program for the analysis of allozyme and molecular population genetic data, Computer Software Distributed by Author. Flagstaff: Northern Arizona University Press; 1997.
- Li X, Ding X, Chu B, et al. Genetic diversity analysis and conservation of the endangered Chinese endemic herb Dendrobium officinale Kimura et Migo (Orchidaceae) based on AFLP. Genetica. 2008;133:159–166.
- Cires E, Cuesta C, Prieto JAF. Genetic diversity and structure in fragmented populations of the endangered species Ranunculus cabrerensis (Ranunculaceae): implications for conservation. Biologia. 2013;68:30–40.
- Hamrick JL, Godt MW. Allozyme diversity in plant species. In: Brown AHD, Clegg MT, Kahler AL, Weir BS, editors. Plant population genetics, breeding and genetic resources. Sunderland: Sinauer Associates Inc.; 1990. p. 43–63.
- Liu YF, Huang HW. Gene flow dynamics and related adaptive evolution in plant populations. Chin Bull Bot. 2009; 44:351–362.
- Avise JC, Hamrick JL. Conservation genetics: case histories from nature. New York, NY: Chapman & Hall; 1996.
- Chen FJ, Wang AL, Chen KM, et al. Genetic diversity and population structure of the endangered and medically important Rheum tanguticum (Polygonaceae) revealed by SSR markers. Biochem Syst Ecol. 2009;37:613–621.