Abstract
Chitosan-based particles are desirable materials for drug delivery because of their muco-adhesiveness in tissues, biocompatibility, low toxicity and effectiveness in antigen transport. It is still a challenge to prepare chitosan-based particles with high stability and by an effective method. Here we developed chitosan-based nanoparticles for the delivery of toll-like receptor 9 ligands, cytosine-phosphate-guanine oligodeoxynucleotides (CpG ODNs), which can induce the change from Th-2 type to Th-1 type immune response. Because of the low tolerance to DNase and less uptake into the cells of the free CpG ODNs, the development of positively charged carriers is necessary for the effective delivery of CpG ODNs into immune cells. Chitosan nanoparticles were prepared by ionic gelation, and the nanoparticles size and surface charge were measured by dynamic light spectroscopy and zeta potential analyzer, respectively. The synthesized chitosan nanoparticles showed two dispersion peaks, at 196 ± 29 nm and 1.33 ± 0.36 μm, with a zeta potential of +3.3 ± 0.4 mV. The toxicity of the chitosan nanoparticles to murine RAW264 macrophages was measured by formazan dye assay utilizing the water-soluble tetrazolium salt WST-8. Our chitosan nanoparticles exhibited no cytotoxicity to RAW264 cells. Finally, we evaluated the immunostimulatory activity of CpG ODNs loaded on chitosan nanoparticles using human peripheral blood mononuclear cells. CpG ODNs significantly enhanced the secretion level of interleukin-6 and interferon-γ by cells compared to the free CpG ODNs. These results indicated that chitosan nanoparticles could be a good candidate for the delivery of CpG ODNs.
Introduction
An immunostimulatory sequence of cytosine-phosphate-guanine oligodeoxynucleotides (CpG ODNs) induces the change from Th-2 type to Th-1 type immune response via the toll-like receptor-9 (TLR-9) [Citation1]. Modification of the phosphodiester (PD) backbone of CpG ODN results in a potent activator of Th-1 type response, while avoiding the phosphorothioate (PS) backbone side effect [Citation2]. CpG ODN 2006x3-PD has been reported as a potentially safe agonist of hTLR9 because of its increased resistance to nuclease degradation [Citation3].
Nasal delivery is very attractive as an important choice for immunostimulatory molecules because of the non-invasive procedure and ease of use. However, there are some limitations of the nasal route administration including the limited halftime of clearance in nasal cavity [Citation4]. Therefore, we require a suitable viscous polymer for longer contact time with nasal mucosa which can induce the mucosa-associated lymphoid tissue.
Nanotechnology has been applied in the development of molecules for drug delivery. The use of nanoparticles could be a suitable method for the delivery of small molecules including peptides and genes [Citation5]. An ideal carrier should be able to protect the active compound in drugs against degradation and promote the uptake by antigen presenting cells. For this purpose, several types of nanoparticles have been developed including organic, inorganic and biological nanoparticles. Organic nanoparticles are considered as interesting materials because of their biodegradability, biocompatibility and ease of preparation. Polylactic-co-glycolic acid (PLGA), chitosan and liposomes are organic nanoparticles that have been widely investigated recently. A nanoformulation of chitosan has been reported to induce the immune response upon nasal administration [Citation6]. Chitosan is one of the good candidates as adjuvant for nasal application because of its muco-adhesiveness in nasal tissue, biocompatibility, low toxicity and effectiveness in antigen transport, as it is able to open the tight junctions of the nasal membrane [Citation4, Citation7].
Low molecular weight chitosan shows a better effect of solubility, biocompatibility, biodegradability and less toxicity compared to high molecular weight chitosan. One attractive technique among the various methods available for chitosan nanoparticle preparation is the ionic gelation technique, which is based on ionic interaction between the positive charges on chitosan and the negative charges of polyanions including tripolyphosphates. This combination was reported as being non-toxic to organisms and prevented the possibility of drug degradation [Citation8, Citation9]. Here we prepared and characterized chitosan nanoparticles as an adjuvant for CpG ODN 2006x3-PD and examined the effects of this combination on PBMC cells and the induction of cytokines and immunoglobulin secretion.
Materials and methods
Nanoparticles synthesis
Following the method reported earlier [Citation9] with slight modification, low molecular weight chitosan (MW ∼15,000, polyscience, Warrington, PA, USA) was dissolved in an aqueous solution of acetic acid (0.02% v/v) to obtain 0.5 mg/mL chitosan solution. The chitosan solution was stirred overnight at room temperature using a magnetic stirrer. The solution pH was adjusted to be 4.7–4.8. Then, the chitosan solution was passed through a syringe filter (0.45 μm, Millipore). Sodium tripolyphosphate (TPP) (Wako Pure Chemical Industries, Osaka, Japan) was dissolved in ultrapure water at a concentration of 0.5 mg/mL and then passed through a syringe filter (0.22 μm, Millipore). A magnetic stirrer was placed at 2–4 °C, then 10 mL of chitosan solution in a 25-mL round bottom flask, preheated in a water bath at 60 °C for 10 min, was placed on the magnetic stirrer with stirring at 700 rpm. Three millilitres of ice-cold sodium TPP solution (2–4 °C) were quickly added to the chitosan solution and kept for 15 min at 60 °C before being cooled down at 4 °C. The resulting suspension was dialyzed in phosphate buffered saline (PBS) using 3.5 K Slide A-Lyzer Dialysis Cassette (Thermo Scientific) and filtered through a 0.22 μm syringe filter. The synthesized chitosan nanoparticles were kept at 4 °C until further experiments.
Characterization of chitosan nanoparticles
The particle size was measured by dynamic light scattering technique (DLS-8000, Otsuka Electronics, Osaka, Japan) with a He-Ne laser. The particle charge was measured using a zeta potential instrument (ELS-Z version 3500/2.13, Otsuka Electronics). The particle shape was measured using a transmission electron microscope (TEM, Microscope Tecnai 200 kV D2360 Super Twin, Thermo Fisher Scientific, Waltham, MA, USA).
Cell culture
The murine macrophage cell line RAW-Blue (InvivoGen, San Diego, CA, USA), which stably expresses the transcription factor nuclear factor-kappa B (NF-κB)/activator protein 1 (AP-1)-inducible secreted embryonic alkaline phosphatase (SEAP) reporter gene, was maintained in high-glucose DMEM supplemented with 10% (v/v) fetal bovine serum (FBS), 100 U/mL penicillin and 100 µg/mL streptomycin at 37 °C in a humidified incubator containing 5% CO2.
Cytotoxicity of chitosan nanoparticles in mouse RAW-blue cells
RAW-Blue cells at a density of 5 × 104 cells/mL in DMEM medium were applied to 96-well plates at 100 μL per well and incubated at 37 °C in a humidified incubator containing 5% CO2 overnight. Then 10 μL of serial dilutions of chitosan nanoparticle solution were added to the wells. After an additional overnight incubation, 10 μL of CCK-8 solution was added to each well, followed by incubation for 1 to 4 h. The absorbance at 450 nm was measured using a microplate reader (MTP-880Lab; Corona Electric, Ibaraki, Japan).
Preparation of CpG ODN-loaded chitosan nanoparticles
PD-based class B CpG oligodeoxynucleotides, referred to as CpG ODN 2006x3-PD[3] (5′-TCGTCGTTTTGTCGTTTTGTCGTT-TCGTCGTTTTGTCGTTTTGTCGTT-TCGTCGTTTTGTCGTTTTGTCGTT-3′), were synthesized by Fasmac Inc (Kanagawa, Japan). The CpG ODN was dissolved in sterile deionized water to a concentration of 100 μmol/L and stored at −20 °C. To prepare the CpG ODN/chitosan nanoparticle conjugate molecule, 50 μL of 20 μmol/L CpG ODN 2006x3-PD was mixed with an equal volume of chitosan nanoparticle solution and then incubated at room temperature for 10 min with gentle shaking.
NF-κB activation in mouse RAW-blue cells
NF-κB/AP-1 activation linked to SEAP was determined using the QUANTI-Blue reagent (InvivoGen). RAW-Blue cells suspended in DMEM medium which contain heat-inactivated FBS were applied as 190-µL cell suspensions to 96-well plates at a density of 5 × 105 cells/mL. Then, 10 µL of CpG ODN/chitosan nanoparticle conjugate or free CpG ODNs were applied to each well and incubated in a humidified incubator containing 5% CO2 overnight. After the incubation period, the supernatant was collected; then, 10 µL of the supernatant was mixed with 90 µL of QUANTI-Blue reagent and incubated at 37 °C in a humidified incubator containing 5% CO2 for 1 to 3 h. The absorbance at 630 nm was measured using a microplate reader. PBS was used as a negative control.
Stimulation of human PBMCs with CpG ODN/chitosan nanoparticle conjugate
Uncharacterized human PBMC (Cellular Technology Limited, Shaker Heights, OH, USA) was thawed according to the manufacturer’s protocol and resuspended in RPMI 1640 medium supplemented with 10% heat-inactivated FBS, 10 μmol/L HEPES, 50 U/mL penicillin, and 50 µg/mL streptomycin. Then, 180 µL of human PMBCs at a density of 2 × 106 cells/mL were applied to a 96-well plate. Then, 20 μL CpG ODN/chitosan nanoparticle conjugate was added to the cells and incubated in a humidified incubator containing 5% CO2. After incubation for 48 h, the supernatant of PBMCs was collected by centrifugation at 10,000×g for 10 min at 4 °C, and then kept in a −20 °C freezer for later use in the enzyme-linked immunosorbent assay (ELISA) measurement.
The quantity of interferon (IL-6) and interferon-gamma (IFN-γ) in the medium was measured by Ready-Set-Go! ELISA kits (eBiosciences, San Diego, CA, USA) following the manufacturer’s protocol.
Results and discussion
Chitosan was synthesized and characterized as nanoparticles
Chitosan is one of the polysaccharides that originate from chitin. Polysaccharides are natural compounds which are non-toxic and biocompatible. Polysaccharides possess a wide range of molecular weights and a significant number of functional groups for chemical modification. Polysaccharides increase the aqueous solubility of the conjugated hydrophobic moiety for drug delivery or similar biomedical purposes [Citation10]. Chitosan has gained interest recently and has been used in many applications, including drug delivery [Citation11]. Chitosan is a non-toxic muco-adhesive biopolymer which is positively charged, biocompatible and biodegradable. Chitosan contains a primary amino group in the main backbone that causes a positive charge in biological fluids. Nano/microparticles can be prepared by treatment with sulphate, citrate and tripolyphosphate. The uniqueness of chitosan facilitates the development of delivery systems for various biological agents [Citation12]. The nanoparticles developed in this study were prepared by the ionic gelation method. Chitosan at 0.5 mg/mL in acidic solution was added dropwise to the 0.05 mg/dL sodium tripolyphosphate solution while stirring continuously. Chitosan undergoes gelation due to the complexation with oppositely charged materials to form spherical particles.
Using the ionic gelation method, we synthesized chitosan nanoparticles with average size of 6.5 nm, 73.6 nm (non-dialyzed), 970.3 nm (dialyzed with PBS) and 196.8–1331.2 nm (dialyzed and filtered in 0.22 μm) as summarized in . We also characterized the positive zeta potential of these nanoparticles by dynamic light scattering technique. shows the zeta potential of the chitosan nanoparticles and the CpG ODN/chitosan nanoparticle conjugate. The zeta potential of the chitosan nanoparticles was 3.3 ± 0.4 mV and became a negative charge of −11.3 ± 1.5 mV after binding with CpG ODN. This result suggested that the negatively charged CpG ODNs was bound to the surface of the positively charged chitosan nanoparticles. The spherical shape of the chitosan nanoparticles was confirmed in .
Figure 1. Zeta potential of the chitosan nanoparticles and CpG ODN/chitosan nanoparticle conjugate. Note: Each sample was measured three times to obtain the average size and standard deviation (SD) values.
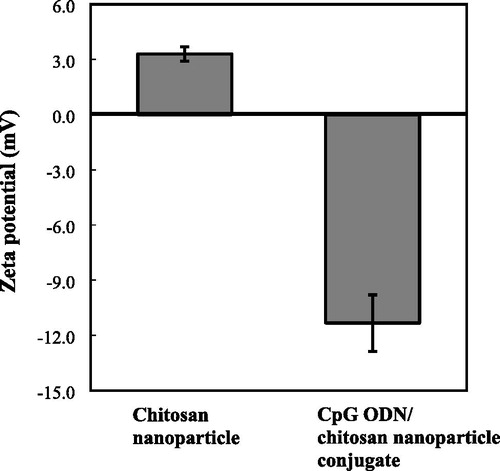
Figure 2. Chitosan nanoparticles observed by transmission electron microscopy (TEM) (magnification 71,000×).
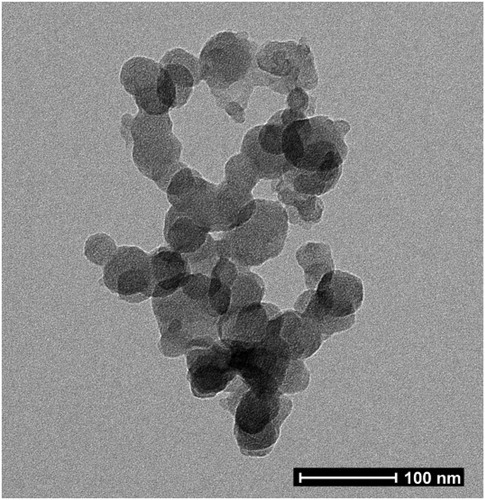
Table 1. Chitosan nanoparticle size as measured by dynamic light scattering methods.
Fan et al. [Citation9] found that low concentrations of chitosan could form stable nanoparticles. In this study, we obtained nanoparticles with two distributed size peaks, while the zeta potential of the nanoparticles in this study was +3.3 (Table 1 and ). This positive charge of zeta potential is in line with previous studies. The lower value of zeta potential in this study explained the formation of the second peak with higher size due to the merger of some nanoparticles. The molecular interactions of ionic cross-linking chitosan-TPP and interaction energies have been investigated in a previous study [Citation13]. The primary cross-linking configurations were defined as H-link, T-link, and M-link, while the interaction energy ranged from 12.3 to 68.3 kcal/mol depending on the tripolyphosphate protonation [Citation13].
Studies have investigated the physical stability of chitosan nanoparticles cross-linked with TPP. The different ionic strengths, chitosan chloride concentration and TPP-to-chitosan ratio have been shown to affect the stability [Citation14]. The particles prepared without NaCl showed higher zeta potential average than the particles that were developed in the presence of NaCl. There were no significant changes in the zeta potential between 1-day to 1-month period of storage. Small or no changes in the hydrodynamic radius (Rh) average were found at low chitosan concentration (0.05%) and TPP:chitosan ratios of 5:95 and 10:90, whereas at the TPP:chitosan ratios of 15:85 and 20:80, sedimentation was observed after 1 month of storage. In our study, we used 0.05% chitosan concentration and TPP:chitosan ratio around 20:80. According to the previous study, this formula formed sedimentation after one month of storage.
Chen et al. [Citation15] reported that the chitosan/CpG conjugate resulted in agglomeration with a size greater than 1 μm. Use of high NaCl and low TPP concentration could decrease the formation of chitosan/TPP micro- and nanogel [Citation16], and moderate amounts of NaCl enhanced the colloidal stability of the chitosan/TPP microgel during formation [Citation17]. The molecular weight of the chitosan used was reported to affect the zeta potential as well. Low molecular weight chitosan had higher zeta potential compared to moderate molecular weight chitosan [Citation18]. Binding and stability of CpG ODN on particles could not be shown in this study because of the negative band of 1, 2, 4 and 24 h incubation time (data not shown). Considering the natural CpG ODN half-life of 10–15 minutes in vivo [Citation19], it is suggested to observe the binding and stability during a period of less than 1 h in future studies.
Chitosan nanoparticles maintain cells viability
Analysis of the cytotoxicity of the carrier in drug delivery systems is essential to confirm the safety of nanoparticles. In this study, the cytotoxicity of chitosan nanoparticles to RAW-Blue cells was evaluated using the CCK-8 assay as shown in , after incubation for 4 h with several dilutions of the nanoparticle solution. The nanoparticles did not render any serious impact to the cells, as shown by the increase in cell viability proportional to the nanoparticle dilution. This suggested that the chitosan nanoparticles are safe and have high potential as a carrier for CpG ODN delivery. It was reported that a chitosan/silica CpG ODN nanohybrid showed cell viability similar to the control group after 24 h and 48 h incubation by using WST-1 assay, which indicates good biocompatibility and low toxicity [Citation20]. Ionic chitosan gels prepared with tripolyphosphate were reported as biocompatible with low toxicity in mouse fibroblast-like NIH-3T3 cells during the 24 h and 72 h incubation as well [Citation21].
CpG ODN and TLR-9 interaction activated NF-κB/AP-1 in murine RAW-blue cells
CpG ODNs are taken up into mammalian cells via the endocytosis pathway and recognized by TLR9 in the endosome/lysosome compartment. The TLR9 signalling pathway leads to the activation of NF-κB, which induces the expression of inflammatory cytokine genes in mammalian cells [Citation22]. shows the expression level of the SEAP gene inducible by the NF-κB and AP-1 transcription factor in murine RAW-Blue cells. The absorbance at 630 nm in each sample increased depending on the incubation time, suggesting that free CpG ODN and also the CpG ODN/chitosan conjugate induced the NF-κB activation via TLR9 and as a result, the expression level of SEAP was improved. The CpG ODN/chitosan conjugate did not show significant difference in NF-κB activation compared to free the CpG ODN. This result suggested that the nanoparticle did not impart an undesirable effect on the CpG ODNs function.
Delivery of CpG ODN 2006x3_PD by chitosan nanoparticles increases inflammatory cytokine production
Multiple CpG motif-linked CpG ODN 2006x3-PD consisting only of a phosphodiester backbone induces the IL-6 secretion in PBMCs via TLR9 [Citation23]. To evaluate the delivery effectiveness of nanoparticles for the proper immune response, we measured the induction of cytokine production in PBMCs by CpG ODN 2006x3-PD, which was delivered by the chitosan nanoparticles. shows the secretion level of the inflammatory cytokine IL-6 and Th-1 type cytokine IFN-γ induced by the chitosan nanoparticles or CpG ODN/chitosan nanoparticle conjugate. The CpG ODN/chitosan nanoparticle conjugate exhibited higher ability to stimulate both IL-6 and IFN-γ induction compared to free CpG ODNs. Interestingly, chitosan nanoparticles also induce both IL-6 and IFN-γ production in human PBMCs, although the chitosan nanoparticles did not stimulate IL-6 production [Citation24].
Figure 5. IL-6 (a) and IFN-γ (b) induction in human PBMCs stimulated with chitosan nanoparticles. Note: The level of induction of each cytokine was measured with three different lots of chitosan nanoparticles. Data are presented as mean values with SD (n = 5). #IL-6 production level is lower than 313 pg/mL. NP, chitosan nanoparticles.
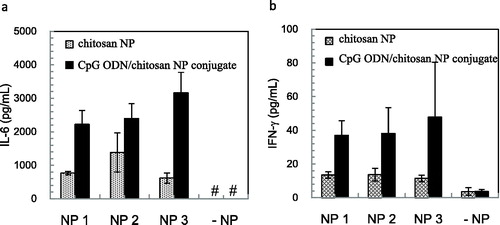
Conclusions
We prepared chitosan nanoparticles as a carrier for the delivery of CpG ODNs. The chitosan particles in this study showed dispersion peaks at 196 ± 29 nm and 1.33 ± 0.36 μm. The chitosan nanoparticles had positive zeta potential and spherical shape and did not show any cytotoxicity to murine macrophage cells. The CpG ODNs loaded on chitosan nanoparticles significantly enhanced the secretion of the inflammatory cytokine IL-6 and Th-1 type cytokine IFN-γ in human PBMCs. These results indicate that the chitosan nanoparticles are a good candidate for the delivery of CpG ODNs. In future studies, we will apply the CpG ODN/chitosan nanoparticle conjugate in nasal mucosa cell delivery systems, as chitosan has the potential to strongly interact with mucosa cells.
Acknowledgments
The authors would like to thank Ms. Satomi Kohara, Ms. Satomi Magae, for technical assistance with the experiments. Part of this work was conducted in NIMS Molecule & Material Synthesis platform, supported by Nanotechnology Platform Program of the Ministry of Education, Culture, Sports, Science and Technology (MEXT), Japan. We would like to thank Editage (www.editage.jp) for English language editing.
Disclosure Statement
The authors report no conflict of interest.
Additional information
Funding
References
- Kitagaki K, Jain VV, Businga TR, et al. Immunomodulatory effects of CpG oligodeoxynucleotides on established th2 responses. Clin Diagn Lab Immunol. 2002;9:1260–1269.
- Schmidt M, Anton K, Nordhaus C, et al. Cytokine and Ig-production by CG-containing sequences with phosphorodiester backbone and dumbbell-shape. Allergy. 2006;61:56–63.
- Meng W, Yamazaki T, Nishida Y, et al. Nuclease-resistant immunostimulatory phosphodiester CpG oligodeoxynucleotides as human toll-like receptor 9 agonists. BMC Biotechnol. 2011;11:88.
- Boonyo W, Junginger HE, Waranuch N, et al. Chitosan and trimethyl chitosan chloride (TMC) as adjuvants for inducing immune responses to ovalbumin in mice following nasal administration. J Control Release. 2007;121:168–175.
- Mahapatro A, Singh DK. Biodegradable nanoparticles are excellent vehicle for site directed in-vivo delivery of drugs and vaccines. J Nanobiotechnol. 2011;9:55.
- Singha S, Shao K, Ellestad KK, et al. Nanoparticles for immune stimulation against infection, cancer, and autoimmunity. ACS Nano. 2018;12:10621–10635.
- Amidi M, Romeijn SG, Borchard G, et al. Preparation and characterization of protein-loaded N-trimethyl chitosan nanoparticles as nasal delivery system. J Control Release. 2006;111:107–116. PubMed PMID: 16380189; eng.
- Calvo P, Remuñán-López C, Vila-Jato JL, et al. Novel hydrophilic chitosan-polyethylene oxide nanoparticles as protein carriers. J Appl Polym Sci. 1997;63:125–132.
- Fan W, Yan W, Xu Z, et al. Formation mechanism of monodisperse, low molecular weight chitosan nanoparticles by ionic gelation technique. Colloids Surf B Biointerfaces. 2012;90:21–27. PubMed PMID: 22014934; eng.
- Basu A, Kunduru KR, Abtew E, et al. Polysaccharide-based conjugates for biomedical applications. Bioconjugate Chem. 2015;26:1396–1412.
- Froidevaux V, Negrell C, Caillol S, et al. Biobased amines: from synthesis to polymers; present and future. Chem Rev. 2016;116:14181–14224.
- Sonia TA, Sharma CP. Chitosan and its derivatives for drug delivery perspective. Chitosan for biomaterials I. In Jayakumar R, Prabaharan M, Muzzarelli R, editors. Advances in polymer science. Berlin: Springer; 2011. p. 23–53.
- Koukaras EN, Papadimitriou SA, Bikiaris DN, et al. Insight on the formation of chitosan nanoparticles through ionotropic gelation with tripolyphosphate. Mol Pharm. 2012;9:2856–2862. PubMed PMID: 22845012; eng.
- Jonassen H, Kjoniksen AL, Hiorth M. Stability of chitosan nanoparticles cross-linked with tripolyphosphate. Biomacromolecules. 2012;13:3747–3756. PubMed PMID: 23046433; eng.
- Chen S, Zhang H, Shi X, et al. Microfluidic generation of chitosan/CpG oligodeoxynucleotide nanoparticles with enhanced cellular uptake and immunostimulatory properties. Lab Chip. 2014;14:1842–1849.
- Huang Y, Lapitsky Y. Salt-assisted mechanistic analysis of chitosan/tripolyphosphate micro-and nanogel formation. Biomacromolecules. 2012;13:3868–3876.
- Huang Y, Lapitsky Y. Monovalent salt enhances colloidal stability during the formation of chitosan/tripolyphosphate microgels. Langmuir. 2011;27:10392–10399.
- Sullivan DJ, Cruz-Romero M, Collins T, et al. Synthesis of monodisperse chitosan nanoparticles. Food Hydrocolloids. 2018;83:355–364.
- Hanagata N. CpG oligodeoxynucleotide nanomedicines for the prophylaxis or treatment of cancers, infectious diseases, and allergies. Int J Nanomed. 2017;12:515.
- Chen S, Zhang H, Chinnathambi S, et al. Synthesis of novel chitosan-silica/CpG oligodeoxynucleotide nanohybrids with enhanced delivery efficiency. Mater Sci Eng C Mater Biol Appl. 2013;3:3382–3388.
- Sacco P, Brun F, Donati I, et al. On the correlation between the microscopic structure and properties of phosphate-cross-linked chitosan gels. ACS Appl Mater Interfaces. 2018;10:10761–10770.
- Gupta GK, Agrawal DK. CpG oligodeoxynucleotides as TLR9 agonists: therapeutic application in allergy and asthma. BioDrugs. 2010;24:225–235.
- Suwarti S, Yamazaki T, Svetlana C, et al. Recognition of CpG oligodeoxynucleotides by human Toll-like receptor 9 and subsequent cytokine induction. Biochem Biophys Res Commun. 2013;430:1234–1239.
- Pattani A, Patravale VB, Panicker L, et al. Immunological effects and membrane interactions of chitosan nanoparticles. Mol Pharm. 2009;6:345–352.