Abstract
Ricinus communis is a traditional medicinal plant which has been utilized for centuries for treatment of various conditions. Due to the presence of diverse phytochemicals, Ricinus is an outstanding natural resource to discover new drugs for various diseases such as diabetes, cancer, arthritis, ulcer and asthma. In this study, we performed whole-genome gene expression profiling using RNA-Seq to determine the differentially expressed genes in a mammalian cell line after exposure to Ricinus leaf extract and elucidate their pharmacological effects in order to support its ethnomedicinal uses. Various genes involved in cancer, inflammation, atherosclerosis and diabetes were found to be differentially regulated after exposure to sub-lethal concentrations of the Ricinus extract in MCF7 cells. An important gene involved in cancer progression and metastasis, that is, PIK3R3 (Phosphatidylinositol 3-kinase regulatory subunit gamma), was downregulated in MCF7 cells after treatment with Ricinus extract. PIK3R3 is an important component of the PI3K/AKT signalling pathway which is essential for cell proliferation, angiogenesis, inhibition of apoptosis and metastasis to distant organs. The Ricinus extract downregulated the expression of DPP4 (Dipeptidyl peptidase-4) and upregulated the expression of PPAR-γ (Peroxisome proliferator-activated receptor gamma) which are crucial in controlling blood glucose levels. Expression of TNFAIP6 (Tumor necrosis factor-inducible gene 6), which is shown to mediate anti-inflammatory and protective effects, was increased after treatment with Ricinus extract. We also analyzed the genes which might also confer toxicity. Our gene expression profiling data corroborate the potential therapeutic benefits of Ricinus communis plant.
Introduction
Ricinus communis, or castor-oil plant, is a traditional medicinal plant which is widely distributed in the Mediterranean region, the Middle East, Eastern Africa and the Indian sub-continent. The castor oil plant is a fast-growing, perennial flowering herb belonging to Euphorbiaceae family. The cultivation of castor plants dates backs to 6000 years ago and is believed to have originated in Tropical Africa [Citation1]. In Saudi Arabia, it is commonly known as Kherwa. It has been used as a therapeutic agent for about 4000 years, for treatment of various ailments and disorders. Every part of the plant has been used for medicinal purposes and it is a multipurpose folk herbal plant with a number of medicinal properties. Castor seeds have been found in Egyptian tombs dating back to 4000 BC, when castor seed oil was mainly used as body ointment and for improvement of hair growth and texture [Citation1]. The application of castor oil in India has been documented since 2000 BC as a laxative, purgative, cathartic and for curing arthritic diseases in the Ayurvedic ethnomedical system [Citation2]. Castor seed and its oil have also been used in China for centuries as traditional medicine for internal use or for use in dressings. In Greece and Rome, it has been used as a laxative for 2500 years [Citation1]. The seed oil of Ricinus has been used as folk therapy for tumours, warts, whitlows; an infusion from leaves is used as an eye lubricant, to treat jaundice and also for relief of stomach ache. The roots are used for various purposes, e.g. as a powerful purgative in the form of decoction, and for relief of toothache [Citation1].
The pharmacological effects of Ricinus are due to its diverse phytochemical composition. The presence of phenols and flavonoids contributes to the inflammatory responses, which is useful in the wound healing process. Earlier studies on Ricinus phytochemicals have demonstrated the presence of gallic acid, ellagic acid, rutin, epicatechin, ricinine, gentisic acid, thujone, kaempferol, lupeol and other bioactive therapeutic compounds which are proved to possess strong anticancer, anti-inflammatory, antidiabetic and antimicrobial activities [Citation3]. Due to the presence of diverse phytochemicals, Ricinus is very effective in the treatment of various diseases such as diabetes, cancer, arthritis, sciatica, ulcer, asthma and has been shown to possess antioxidant, immune modulatory, laxative, larvicidal, anti-inflammatory and antimicrobial properties [Citation1, Citation3].
Ricinus and its phytochemicals are known for their anticancer activity against different types of cancers. Its anticancer activity is attributed to the presence of various phytochemicals such as quercitin, rutin, ricin, thujone, lectins etc. In vitro studies using Ricinus extract have revealed its cytotoxic effects on various cancer cell lines such as colon, liver, breast, cervix, melanoma and prostate cancer [Citation4]. Agglutinin 1, isolated from Ricinus plants, has been shown to promote apoptosis of endothelial cells in tumour blood vessels by inhibiting the expression of VEGFR-2 [Citation5]. Several studies have indicated the potential of Ricinus in curing diabetes. In vivo studies using ethanolic extract of Ricinus leaves on streptozocin-induced diabetic rats demonstrated that there was significant decrease in the glucose levels in diabetic rats after the treatment and moreover, the insulin levels were dramatically increased in the treated rats as compared to control animals [Citation6,Citation7]. Anti-inflammatory activity of Ricinus has been demonstrated in two animal models, that is, the carrageenan-induced paw edema model and the cotton pellet granuloma model. Ricinus methanolic extract significantly reduced edema in both the animal models [Citation8]. Topical application of ricinoleic acid isolated from Ricinus plant has been shown to exhibit significant analgesic and anti-inflammatory effects. After induction of acute inflammation by intradermal injection of carrageenan in the mouse or by histamine in the guinea-pig eyelid, ricinoleic acid was topically applied for 8 days. Ricinoleic acid was able to reduce the edema in both the animal models, suggesting its application as an anti-inflammatory agent [Citation9]. Ricinus and its phytochemicals have also been shown to possess potent anti-bacterial and anti-fungal activities. Various studies have reported the antimicrobial activity of the crude extract against several pathogens such as Staphylococcus aureus, Escherichia coli, Streptococcus mutans, Enterococcus faecalis, MRSA (methicillin-resistant Staphylococcus aureus), Candida albicans, Aspergillus niger and Aspergillus fumigatus [Citation10–12]. A randomized clinical study demonstrated the efficacy of Ricinus compounds in inhibiting biofilm formation. The anti-bacterial activity was found to be due to the presence of sodium ricinoleate in Ricinus oil, which disrupts cell walls and causes cell death by loss of cytoplasmic content [Citation13]. Besides this therapeutic potential, the Ricinus plant has also been shown to possess anti-oxidant, anti-fertility, anti-helminthic, mosquitocidal, bone regenerative and wound healing activities [Citation14–18]. In this study, we performed whole-genome gene expression profiling using RNA-Seq to identify the differentially regulated genes in a mammalian cell line after exposure to Ricinus extract and elucidate their pharmacological effects in order to support the ethnomedicinal uses of the Ricinus plant.
Materials and methods
Preparation of plant extract
Ricinus communis leaves were collected from KAU farm, Jeddah, Saudi Arabia. The leaves were washed thoroughly with tap water and distilled water to remove any kind of microbes and dust particles from the surface. The plant leaves were dried under shade for 8 days and then were powdered using an electric blender. Twenty-five grams of powdered leaves were dissolved in 100 mL of hexane and were incubated at room temperature in a shaking incubator at 150 rpm for 24 hours, followed by incubation with ethanol (100%) for 48 hours in a shaking incubator. The solution was filtered with Whatman No. 1 filter paper followed by centrifugation at 1000 g for 15 minutes at 4 °C and was further filtered. After filtration, the solvent was subjected to rotary evaporator (BUCHI, Switzerland) under pressure and at 40 °C to obtain the extract. The Ricinus leaf extract was stored at 4 °C until further use and a stock was kept at −20 °C.
Cell culture
MCF7, a breast cancer cell line, was procured from the American Type Culture Collection (ATCC) and propagated in DMEM medium supplemented with 10% (v/v) fetal bovine serum (FBS), 100 μg/mL streptomycin and 100 U/mL penicillin at 37 °C in a humidified incubator with 5% CO2.
Cell viability assay
MCF7 cells (2 × 104 cells/well) were sub-cultured in 24-well plates and incubated at 37 °C in a humidified incubator with 5% CO2 for 20 h. Cells were treated with different doses of Ricinus leaf extract: that is, 10, 50, 100, 200 and 500 μg/mL, and further incubated at 37 °C in a humidified incubator with 5% CO2 for 24 h. After the treatment with the extract, cells were analyzed under a phase contrast microscope (Nikon, Japan).
Apoptosis assay
Hoechst 33342 staining was performed to detect the nuclear morphological changes in MCF7 cells after exposure to the Ricinus leaf extract. Briefly, MCF7 cells (2 × 104 cells/well) were cultured on coverslips placed in 24-well plates and treated with 50 μg/mL of Ricinus extract for 24 h. The cells were washed with Dulbecco’s phosphate buffer saline (DPBS) and fixed by treating with 100% methanol for 10 min. Cells were washed three times with DPBS and stained with Hoechst 33342 for 20 min at room temperature. The cells were washed three times with DPBS and were observed under a fluorescent microscope (Nikon, Japan).
RNA extraction and RNA-Seq analysis
MCF7 cells (1.5 × 105 cells) were exposed to different doses (sub-lethal) of Ricinus leaf extract, that is, 10 μg/mL and 50 μg/mL, in triplicates, for 24 h at 37 °C. Total RNA was extracted from the treated cells using TRIzol reagent by following the manufacturer’s instructions. RNA-Seq analyses was performed using Illumina HiSeq. Each RNA sample was ribo-depleted (Ribo-Zero, Epicentre) before cDNA library preparation (ScriptSeq epicenter). Each cDNA thus prepared, was separately barcode tagged during library preparation and sequenced using Illumina HiSeq, generating approximately >40 million 100-bp reads per sample for the treated MCF7 cells. The Illumina reads were mapped to the respective assembled reference transcriptome sequence using the mapping software TopHat and Cufflinks [Citation19,Citation20]. Illumina read abundances mapping to each reference contig was normalized using the RPKM calculation (reads per Kb per million reads). Differentially expressed transcripts were identified and functionally annotated using different statistical analysis tools available via Bioconductor in R [Citation21]. Clusters of differentially expressed and cycling transcripts were annotated to identify over-represented GO/KEGG pathways [Citation22]. The common differentially expressed genes at the two different doses of Ricinus extract, in both the upregulated and the downregulated gene lists, were identified by using Venny online tool. The common differentially modulated genes were selected and subjected to ClustVis generating Heat map. Further bioinformatics analysis was performed by using OMIM, Lincs-NIH and other tools available for human diseases and their associated genes.
Results and discussion
Cytotoxic activity of Ricinus extract
The leaves of Ricinus plants contain various phytochemicals with diverse structure and pharmacological activities. To evaluate the pharmacological properties of R. communis, we prepared an ethanolic extract of Ricinus leaves. Prior to the extraction with ethanol, the leaves were treated with hexane in order to remove the lipids and oil content. The leaf extract was tested at varying doses for its cytotoxic potential against MCF7, a breast cancer cell line. The exposure of MCF7 cells to the Ricinus extract led to cell shrinkage, cell detachment and loss of adherent phenotype, at a dose of 100 µg/mL and above (). Apoptosis assay using Hoechst staining revealed nuclear fragmentation and chromatin condensation in MCF7 cells after treatment with the Ricinus extract, which are important features of apoptosis ().
Figure 1. Cytotoxic potential of Ricinus leaf extract in MCF-7 breast cancer cell line. Control cells in DMSO (A) and cells treated with 100 µg/mL (B), 200 µg/ml (C) and 500 µg/ml (D) of Ricinus extract (scale bar: 10 μm).
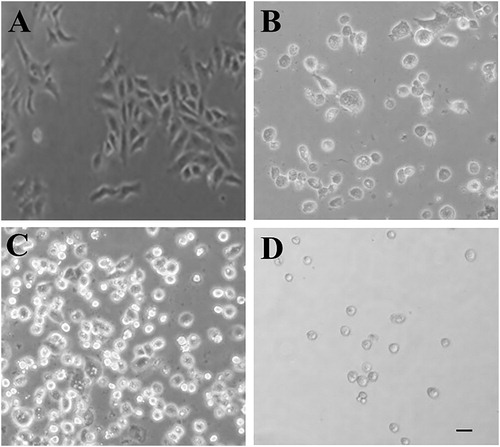
Figure 2. Apoptotic cell death induced by Ricinus leaf extract in MCF7 cells. Fluorescent images from Hoechst 33342 staining. Control MCF7 cells treated with DMSO alone (A) vs. MCF7 cells treated with 50 μg/mL of Ricinus leaf extract (B). Arrows indicate chromatin condensation and nuclear fragmentation (scale bar: 10 μm).
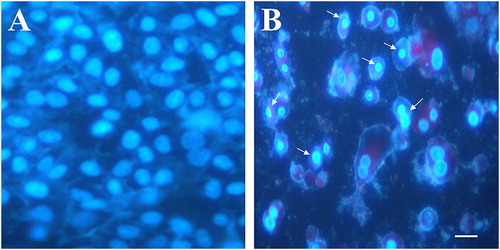
Differential gene expression in MCF7 cells exposed to Ricinus extract
Gene expression profiling using RNA-Seq was carried out to determine the differentially regulated genes after treatment with Ricinus extract. MCF7 cells were exposed to sublethal doses of 10 µg/mL and 50 µg/mL of the Ricinus extract. At these particular doses, cytotoxicity was not detected. Total RNA was purified from DMSO-treated control cells and Ricinus extract treated MCF7 cells and RNA-Seq was carried out using Illumina HiSeq. Approximately >40 million 100-bp reads per sample for the treated MCF7 cells were generated. The Cufflinks suite [Citation19,Citation20] was applied to assemble the transcriptome from the RNA-seq data and to quantify the expression levels. The human hg38 reference transcriptome assembly was used for guidance. The program Cuffdiff was employed for analysis of the transcript expression levels to recognize genes which were differentially regulated in MCF7 cells treated with the Ricinus extract as compared to the DMSO treated control cells. Differential gene expression analysis revealed that, as compared to the DMSO treated control MCF7 cells, there were 245 upregulated genes (fold change > 1; P < 0.05) and 134 downregulated genes (fold change < −1; P < 0.05) in MCF7 cells treated with 10 µg/mL of Ricinus extract. In the case of MCF7 cells treated with 50 µg/mL of Ricinus extract, there were 168 upregulated genes (fold change > 1; P < 0.05) and 73 downregulated genes (fold change < −1; P < 0.05) in comparison to the DMSO treated control cells ( and Citation2). The common differentially expressed genes at the two different doses of Ricinus extract, in both the upregulated and the downregulated gene lists, were identified by using Venny online tool. As shown in , a total of 37 genes (21.8%) were downregulated and 107 genes (35%) were upregulated in MCF7 cells at the two tested doses of Ricinus extract in a dose-dependent manner. The common differentially modulated genes were selected and subjected to ClustVis generating Heat map ().
Figure 3. Venn diagram depicting the common genes differentially regulated in MCF7 cells after treatment with different doses of Ricinus extract. Downregulated (A) and upregulated (B) genes in MCF7 cells after exposure to Ricinus extract at 10 μg/mL dose (1) or 50 μg/mL dose (2).
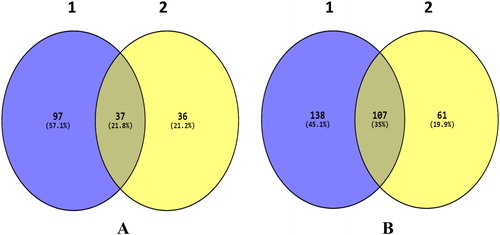
Figure 4. Cluster analysis of genes from MCF7 cells after treatment with Ricinus extract at 10 μg/mL dose and 50 μg/mL dose as compared to DMSO treated control cells.
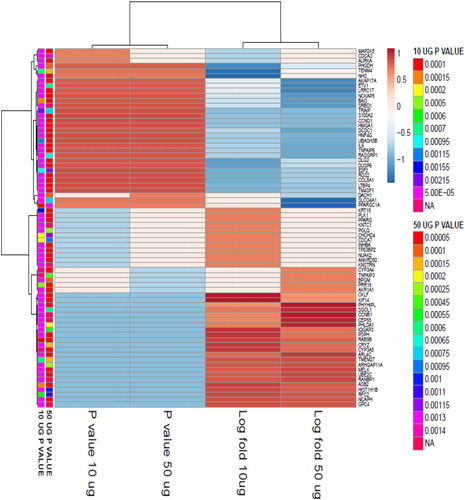
Table 1. List of significantly down-regulated genes in MCF7 cells after exposure to Ricinus extract.
Various genes involved in cancer, inflammation and diabetes were differentially regulated after exposure to the sub-lethal concentrations of Ricinus extract in MCF7 cells (). One of the important genes involved in cancer progression and metastasis, that is, PIK3R3 (phosphatidylinositol 3-kinase regulatory subunit gamma), was downregulated in MCF7 cells after treatment with the Ricinus extract. The inhibition of PIK3R3 expression was significantly higher at a dose of 50 µg/mL as compared to 10 µg/mL of Ricinus extract. PIK3R3 is an important component of the PI3K/AKT signalling pathway, which is essential for cell proliferation, angiogenesis, inhibition of apoptosis, escape of cells from the primary tumour site and invasion of these tumour cells into distant organs (). PI3K signalling is highly activated in several human cancers [Citation23,Citation24]. There are three isoforms of phosphatidylinositol 3-kinase (PI3Ks) and each isoform comprises of a catalytic subunit (i.e. p110α, p110β or p110δ) and a regulatory subunit (i.e. p85α, p85β or p55γ). PIK3R3 encodes the p55γ regulatory subunit [Citation25]. The PIK3R3 regulatory unit is involved in tumour growth and development and is overexpressed in various cancers [Citation26,Citation27]. Overexpression of PIK3R3 has been shown to induce Epithelial-to-Mesenchymal Transition (EMT) and to promote metastasis in colorectal cancer [Citation26]. The observed downregulation of PIK3R3 in MCF7 by the Ricinus extract could suggest that the phytochemicals present in the leaves might mediate its anti-cancer activity by targeting the PI3K signalling pathway. MAF oncogene (v-maf avian musculoaponeurotic fibrosarcoma oncogene homolog) was also found to be downregulated in MCF7 cells after treatment with Ricinus extract in a dose dependent manner. Overexpression of MAF has been detected in primary breast tumors and is associated with high risk of metastasis to bones [Citation28]. Our data clearly suggested that the phytochemicals present in the leaves of Ricinus plants have a potential to prevent tumuor growth and metastasis.
Figure 5. KEGG pathway analysis of differentially regulated genes in MCF7 cells after treatment with Ricinus leaves extract depicting the affected genes in the PI3K/AKT signalling pathway. Modulated genes are marked with a red star on top.
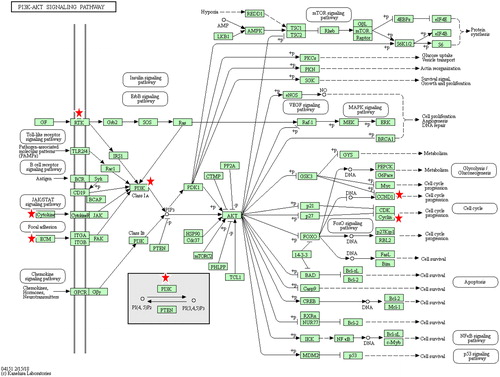
Table 3. List of the differentially regulated genes with potential therapeutic implications.
The TNFRSF19 gene (Tumor necrosis factor receptor superfamily, member 19) also termed as TROY, was found to be downregulated in MCF7 cells after exposure to the Ricinus extract. TNFRSF19 has been shown to play key role in glioblastoma invasion and resistance to chemotherapy [Citation29].
An anti-inflammatory potential of the Ricinus extract was indicated by the upregulation of the TNFAIP6 gene (Tumour necrosis factor-inducible gene 6) in MCF7 cells after treatment with the Ricinus extract. TNFAIP6 was upregulated in MCF7 cells after treatment with Ricinus extract at both doses used in this study. TNFAIP6, also termed as TNF-stimulated gene 6 (TSG-6), has been shown to mediate anti-inflammatory and protective effects in disease models by lowering neutrophil infiltration [Citation30]. It has been shown that TNFAIP6 binds and interacts with multiple chemokines and mediates the anti-inflammatory response.
The antidiabetic potential of Ricinus extracts has been revealed by various studies. Treatment of alloxan diabetic rats with an ethanolic extract of Ricinus roots resulted in significant lowering of fasting blood sugar and an increase in insulin levels [Citation6,Citation7]. One of the important phytochemicals present in the leaves and roots of Ricinus plants is rutin, which is a flavonoid and is also found in other medicinal plants. Rutin has been shown to possess anti-diabetic, anti-inflammatory, neuroprotective and hepatoprotective activities [Citation31]. Administration of rutin in streptozotocin induced diabetic rats, significantly reduced the fasting blood glucose and improved glucose tolerance. Moreover, treatment with rutin caused a decrease in serum levels of triglyceride and low-density lipoprotein (LDL) in diabetic rat models [Citation32,Citation33]. It has been demonstrated that rutin causes an increase in the expression of PPARγ, which improves the insulin resistance and enhances the glucose uptake in muscle and adipose tissue [Citation34]. Our gene expression profiling data revealed that treatment with the Ricinus extract, increased the expression of PPAR-γ (Peroxisome proliferator-activated receptor gamma), which is involved in the regulation of fatty acid storage and glucose metabolism. PPAR-γ agonists have been used for treatment of diabetes as these compounds improve the insulin resistance and lower the serum glucose levels [Citation35,Citation36]. Besides controlling hyperglycemia and hyperlipidaemia, PPAR-γ decreases the inflammatory response in cardiovascular cells and also prevents atherosclerosis. Moreover, PPAR-γ agonists have been shown to inhibit the estrogen synthesis in breast adipose tissue and to prevent the proliferation of tumour cells [Citation37]. Exposure to the Ricinus extract also resulted in upregulation of PPARGC1A or PGC-1α (Peroxisome proliferator-activated receptor gamma coactivator 1-alpha), which interacts with PPAR-γ and regulates various genes involved in energy metabolism (). The Ricinus extract was found to downregulate the expression of DPP4 (Dipeptidyl peptidase-4), which is also known to play an important role in glucose metabolism by degrading incretins such as GLP-1 [Citation38]. It has been demonstrated that treatment with DPP-4 inhibitors could lead to an increase in GLP-1 levels which inhibit glucagon release and stimulate insulin secretion and subsequently decrease the blood glucose levels [Citation38,Citation39]. Our data suggested that the leaves of Ricinus plants contain a phytochemical which has potential to treat diabetes.
Figure 6. Schematic diagram depicting the differentially regulated genes in MCF7 cells after treatment with Ricinus leaves extract and their pharmacological effect. Arrows denote downregulation (↓) and upregulation (↑) in gene expression.
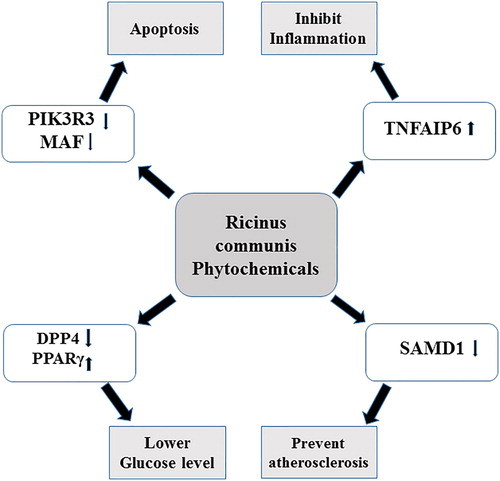
Another important gene, SAMD1 (Sterile alpha motif domain-containing protein 1), was downregulated in MCF7 cells after treatment with the Ricinus extract. SAMD1, also known as Atherin, has been shown to play a role in atherosclerosis by immobilizing LDL in the arterial wall [Citation40]. Our data indicated that Ricinus leaves can be a good source to isolate new compounds to prevent atherosclerosis.
Besides, the above-mentioned potential therapeutic applications, Ricinus plant extracts have also been shown to possess potent anti-bacterial activity [Citation41]. In addition to its application in medicine, R. communis is applied in cosmetics, animal feed and in the manufacturing of biodiesel, plastics and lubricants [Citation42]. A recent study demonstrated the potential of Ricinus plants for phytoremediation of cadmium in hydrophonic conditions, suggesting its utility in phytoremediation of various heavy metal contaminants [Citation43]. Despite of the wide range of application of Ricinus plants, their toxicity remains a major concern. The toxicity of R. communis has been known for centuries and it is now attributed to a protein known as ricin which is mainly present in seeds. Ricin is considered highly toxic to humans and symptoms of ricin toxicity include nausea, abdominal pain, bloody diarrhea, tachycardia and low-blood pressure, potentially causing death if not treated [Citation44]. Since ricin is found in low amounts in leaves, it can be easily removed during the extraction process. The leaves of Ricinus plants, however, contain another toxin, ricinine, which is an alkaloid and has shown toxicity against various insect pests [Citation45]. Our gene expression profiling data revealed the induction of genes which can cause inflammation. The IL-6 gene, which encodes a pro-inflammatory protein, was found to be highly upregulated in Ricinus extract treated cells (), indicating that some of the phytochemicals present in the Ricinus extract may have potential to cause inflammation [Citation46]. Treatment with the Ricinus extract induced the expression of heat shock proteins such as HSP90AB2P, which act as molecular chaperons and assist in the maturation and structural maintenance of cellular proteins during stress conditions. The Ricinus extract was also found to induce the expression of the gene encoding Cyp3A4, an important drug-metabolizing enzyme.
Table 2. List of the significantly upregulated genes in MCF7 cells after exposure to Ricinus extract.
For centuries, different parts of Ricinus communis plants including leaves, seeds and roots have been used as therapeutic agents for treatment of various ailments all over the world. The Ricinus plant contains several phytochemicals with diverse pharmacological activities. These compounds are found to be responsible for various therapeutic effects including anticancer, anti-diabetic, anti-microbial, antioxidant, anti-inflammatory, hepatoprotective, bone regenerative and analgesic activity. The results from the whole-genome gene expression profiling using RNA-Seq obtained in this study revealed some differentially expressed genes in a mammalian cell line after treatment with Ricinus extract. The analysis of their potential biological effects can be used to correlate the affected genes and biological pathways with the ethnomedicinal uses and potential therapeutic benefits of Ricinus plants.
Conclusions
The data obtained in this study corroborate the various potential therapeutic benefits of Ricinus communis. Genes associated with cancer, inflammation, atherosclerosis and diabetes were found to be differentially modulated after exposure to the Ricinus extract in MCF7 cells. These data would be very useful while isolating and characterizing new compounds from Ricinus leaves which specifically target these conditions.
Acknowledgements
The authors, therefore, acknowledge with thanks, the Deanship of Scientific Research (DSR), King Abdulaziz University, Jeddah, for technical and financial support.
Funding
This project was funded by the Deanship of Scientific Research (DSR), King Abdulaziz University, Jeddah, under Grant number 62-130-35-HiCi.
Disclosure Statement
The authors declare that there are no conflicts of interests.
References
- Scarpa A, Guerci A. Various uses of the castor oil plant (Ricinus communis L.) a review. J Ethnopharmacol. 1982;5:117–137.
- Dastur JF. Medicinal plants of India and Pakistan. Bombay (India). Mumbai: D. B. Taraporevala Sons and Co.; 1962. p. 142.
- Ribeiro PR, de Castro RD, Fernandez LG. Chemical constituents of the oilseed crop Ricinus communis and their pharmacological activities: A review. Ind Crops Prod. 2016;91:358–376.
- Prakash E, Gupta DK. In vitro study of extracts of Ricinus communis Linn on human cancer cell lines. J Med Sci Pub Health. 2014;2:15–20.
- You W, Kasman I, Hu-Lowe DD, et al. Ricinus communis Agglutinin I leads to rapid down-regulation of VEGFR-2 and endothelial cell apoptosis in tumor blood vessels. Am J Pathol. 2010;176:1927–1940.
- Shokeen P, Anand P, Murali YK, et al. Antidiabetic activity of 50% ethanolic extract of Ricinus communis and its purified fractions. Food Chem Toxicol. 2008;46:3458–3466.
- Matthew OO, Olusola L, Matthew OA. Preliminary study of hypoglycaemic and hypolipidemic activity of aqueous root extract of Ricinus communis in alloxan-induced diabetic rats. J Phys Pharm Adv. 2012;2:354–359.
- Valderramas AC, Moura SHP, Couto M, et al. Anti-inflammatory activity of Ricinus communis derived polymer. Brazilian J Oral Sci. 2008;7:1666–1672.
- Vieira C, Evangelista S, Cirillo R, et al. Effect of ricinoleic acid in acute and subchronic experimental models of inflammation. Mediators Inflamm. 2000;9:223–228.
- Jeyaseelan EC, Jashothan PTJ. In vitro control of Staphylococcus aureus (NCTC 6571) and Escherichia coli (ATCC 25922) by Ricinus communis L. Asian Pac J Trop Biomed. 2012;2:717–721.
- Naz R, Bano A. Antimicrobial potential of Ricinus communis leaf extracts in different solvents against pathogenic bacterial and fungal strains. Asian Pac J Trop Biomed. 2012; 2:944–947.
- Vandita P, Amin N, Khyati P, et al. Effect of phytochemical constituents of Ricinus Communis, Pterocarpus santalinus, Terminalia belerica on antibacterial, antifungal and cytotoxic activity. Int J Toxicol Pharmacol Res. 2013; 5:47–54.
- Salles MM, Badaro MM, Ferraz de Arruda CN, et al. Antimicrobial activity of complete denture cleanser solutions based on sodium hypochlorite and Ricinus communis – a randomized clinical study. J Appl Oral Sci. 2015; 23:637–642.
- Beloti MM, de Oliveira PT, Tagliani MM, et al. Bone cell responses to the composite of Ricinus communis polyurethane and alkaline phosphatase. J Biomed Mater Res. 2008; 84:435–441.
- Nath S, Choudhury MD, Roychoudhury S, et al. Male contraceptive efficacy of Ricinus communis L. extract. J Ethnopharmacol. 2013; 149:328–334.
- Rana M, Kumar H, Parashar B. In vitro anti-helmintic activity of bark of Ricinus communis Linn. J Chem Pharm Res. 2013; 5:40–42.
- Ibraheem O, Maimako RF. Evaluation of alkaloids and cardiac glycosides contents of Ricinus communis linn. (castor) whole plant parts and determination of their biological properties. Int J Toxicol Pharmacol Res. 2014; 6:34–42.
- Wachira SW, Omar S, Jacob JW, et al. Toxicity of six plant extracts and two pyridine alkaloids from Ricinus communis against the malaria vector Anopheles gambiae. Parasit Vectors. 2014;7:312.
- Trapnell C, Pachter L, Salzberg SL. TopHat: discovering splice junctions with RNA-Seq. Bioinformatics. 2009; 25:1105–1111.
- Trapnell C, Williams BA, Pertea G, et al. Transcript assembly and abundance estimation from RNA-Seq reveals thousands of new transcripts and switching among isoforms. Nat Biotechnol. 2010;28:511–515.
- Durinck S, Moreau Y, Kasprzyk A, et al. BioMart and Bioconductor: a powerful link between biological databases and microarray data analysis. Bioinformatics. 2005;21:3439–3440.
- Kanehisa M, Goto S, Sato Y, et al. KEGG for integration and interpretation of large-scale molecular data sets. Nucleic Acids Res. 2012; 40:D109–D114.
- Courtney KD, Corcoran RB, Engelman JA. The PI3K pathway as drug target in human cancer. J Clin Oncol. 2010; 28:1075–1083.
- Jiang BH, Liu LZ. PI3K/PTEN signaling in angiogenesis and tumorigenesis. Adv Cancer Res. 2009;102:19–65.
- Backer JM. The Regulation of class IA PI 3-kinases by inter-subunit interactions. Curr Top Microbiol Immunol. 2010;346:87–114.
- Wang G, Yang X, Li C, et al. PIK3R3 induces epithelial- to- mesenchymal transition and promotes metastasis in colorectal cancer. Mol Cancer Ther. 2014;13:1837–1847.
- Zhang L, Huang J, Yang N, et al. Integrative genomic analysis of phosphatidylinositol 3-kinase family identifies PIK3R3 as a potential therapeutic target in epithelial ovarian cancer. Clin Cancer Res. 2007;13:5314–5321.
- Pavlovic M, Arnal-Estape A, Rojo F, et al. Enhanced MAF oncogene expression and breast cancer bone metastasis. J Natl Cancer Inst. 2015;107:djv256.
- Dhruv HD, Roos A, Tomboc PJ, et al. Propentofylline inhibits glioblastoma cell invasion and survival by targeting the TROY signaling pathway. J Neurooncol. 2016;126:397–404.
- Dyer DP, Salanga CL, Johns SC, et al. The Anti-inflammatory protein TSG-6 regulates chemokine function by inhibiting chemokine/glycosamino glycan interactions. J Biol Chem. 2016; 291:12627–12640.
- Ghorbani A. Mechanisms of antidiabetic effects of flavonoid rutin. Biomed Pharmacother. 2017;96:305–312.
- Niture NT, Ansari AA, Naik SR. Anti-hyperglycemic activity of rutin in streptozotocin- induced diabetic rats: an effect mediated through cytokines, antioxidants and lipid biomarkers. Ind J Exp Biol. 2014;52:720–727.
- Jadhav R, Puchchakayala G. Hypoglycemic and antidiabetic activity of flavonoids: boswellic acid, ellagic acid, quercetin, rutin on streptozotocin-nicotinamide induced type 2 diabetic rats. Int J Pharm Pharm Sci. 2012; 4:251–256.
- Ahmed OM, Moneim AA, Yazid IA, et al. Antihyperglycemic, anti- hyperlipidemic and antioxidant effects and the probable mechanisms of action of Ruta graveolens infusion and rutin in nicotinamide-streptozotocin-induced diabetic rats. Diabetol Croat. 2010;39:15–35.
- Kubota N, Terauchi Y, Kubota T, et al. Pioglitazone ameliorates insulin resistance and diabetes by both adiponectin-dependent and -independent pathways. J Biol Chem. 2006;281:8748–8755.
- Lehman JM, Moore LB, Smith-Oliver TA, et al. An antidiabetic thiazolidinedione is a high affinity ligand for peroxisome proliferator- activated receptor (PPAR gamma). J Biol Chem. 1995;270:12953–12956.
- Rubin GL, Ying Zhao Y, Kalus AM, et al. Peroxisome proliferator- activated receptor g ligands inhibit estrogen biosynthesis in human breast adipose tissue: Possible implications for breast cancer therapy. Cancer Res. 2000; 60:1604–1608.
- McIntosh C, Demuth H, Pospisilik J, et al. Dipeptidyl peptidase IV inhibitors: How do they work as new antidiabetic agents?. Regul Pept. 2005;128:159–165.
- Behme MT, Dupré J, McDonald TJ. Glucagon-like peptide 1 improved glycemic control in type 1 diabetes. BMC Endocr Disord. 2003;3:3.
- Lees AM, Deconinck AE, Campbell BD, et al. Atherin: a newly identified, lesion-specific, LDL-binding protein in human atherosclerosis. Atherosclerosis. 2005;182:219–230.
- Hajrah NH, Abdul WM, Sabir JSM, et al. Anti-bacterial activity of Ricinus communis L. against bacterial pathogens Escherichia coli and Klebsiella oxytoca as evaluated by Transmission electron microscopy. Biotech Biotechnol Equip. 2018;32:686–691.
- Lopes DC, Steidle Neto AJ. Potential crops for Biodiesel production in Brazil: A review. World J Agri Sci. 2011;7:206–217.
- Hadi F, Ul Arifeen MZ, Aziz T, et al. Phytoremediation of cadmium by Ricinus communis L. in hydrophonic condition. Am-Eura J Agri Environ Sci. 2015;15:1155–1162.
- Worbs S, Köhler K, Pauly D, et al. Ricinus communis intoxications in human and veterinary medicine-a summary of real cases. Toxins (Basel). 2011;3:1332–1372.
- Burgess EPJ, Koha EMWT, Hutchins RFN, et al. Toxicity of leaves from the castor oil plant, Ricinus communis L. (Euphorbiaceae), to adult grass grub, Costelytra zealandica (White) (Coleoptera: Scarabaeidae). N Z J Experi Agri. 1988;16:63–66.
- Ishihara K, Hirano T. IL-6 in autoimmune disease and chronic inflammatory proliferative disease. Cytokine Growth Factor Rev. 2002;13:357–368.
- Wei W, Jing ZX, Ke Z, et al. Sirtuin 7 plays an oncogenic role in human osteosarcoma via downregulating CDC4 expression. Am J Cancer Res. 2017; 7:1788–1803.
- Puigserver P, Spiegelman BM. Peroxisome proliferator-activated receptor-gamma Coactivator 1 alpha (PGC-1 alpha): transcriptional coactivator and metabolic regulator. Endocrine Rev. 2003;24:78–90.