Abstract
This review provides data on the possibilities for commercial use of algae of the green streptophyte genus Klebsormidium. It is one of the basal algal progenitors of land plants and shows many mechanisms and features which allow its adaptation to different environmental stresses associated with land colonization. This has led to formation of structures and compounds with countless possible applications in biotechnology and nanotechnology, starting from food to pharmaceuticals and biofuels. Therefore, the present work aims to provide: (1) a review on this yet neglected topic for the first time; (2) reliable identification using the polyphasic taxonomic approach of three local Klebsormidium strains isolated from soils of two protected areas in Bulgaria (Kresnendko Defile and National park Rila). It also outlines the cultivation advantages of Klebsormidium broad tolerance to harsh conditions and its reproductive features (easy disintegration and possibility to produce resting stages). The possibility to use inoculum of akinetes in soil algalization as biofertilizers is proposed. The results from standard microscopical identification and molecular data (ITS and rbcL, used separately and in combination), combined in the applied polyphasic approach led to distinguishing between geographically distinct strains collected at different altitudes. After identification of two strains (ACUS 00001 and ACUS 00014) as belonging to Klebsormidium dissectum and of ACUS 00207 as belonging to Klebsormidium klebsii, the similarity of the latter taxon with K. nitens is discussed.
Introduction
The interest in phycoprospecting, or searching for local algal strains of commercial interest, is rapidly increasing parallel to the need for reliable identification of discovered algae, based on the polyphasic approach [Citation1]. Algae have become a central focus of bioeconomy and biotechnology in particular due to their countless applications starting from food and pharmaceuticals to biofuels [Citation2–4]. Currently algae are considered crucial organisms of advancing nanotechnology [Citation5]. Most biotechnological and nanotechnological studies are oriented towards fast-replicating unicellular microalgae or large seaweeds [Citation2, Citation5–9]. Less attention has been paid to the group of medium-sized filamentous algae, which – despite their microscopical dimensions – become well-visible when developing in mats or crusts. Amongst this group is the green non-branched filamentous genus Klebsormidium, which grows in different types of habitats with preference to the aeroterrestrial mode of life [Citation10–14]. The genus is described as cosmopolitan, yet it is mainly distributed in temperate regions with less records from the tropics [Citation10, Citation11, Citation15–18], and a decrease in species richness towards the poles [Citation19]. Klebsormidium has attracted the attention of scientists mainly due to its complicated taxonomy (e.g. [Citation12, Citation20, Citation21]) and unusual plastid genome with low content of protein-coding genes [Citation22] combined with its evolutionary significance based on its position among the streptophyte lineage of potential algal land plant progenitors [Citation23, Citation24]. Last but not least, the interest in the ecology, physiology, biochemistry and genetics of Klebsormidium is related to its important contribution to soil, its crusts and other diverse aeroterrestrial communities which survive the harsh environmental conditions in natural and urban habitats [Citation16–18, Citation25–42] with ability for endolithic growth [Citation43–45]. Furthermore, the species of this genus can grow at a broad range of pH (e.g. [Citation13, Citation46–49]), have high photophysiological plasticity [Citation50] and demonstrate viability after long air-drying periods [Citation51–53].
Current investigations revealed that Klebsormidium contains phototropins [Citation54] and its genome has acquired genes (some of them similar to land plants) which allow specific adaptation to terrestrial life by providing protection against the detrimental effects of high-intensity light [Citation55]. These results are in accordance with the suggested gradual colonization of land habitats by descendents of streptophyte algae, for which the major advantage was the physiological pre-adaptation to terrestrial existence by their primary freshwater life style [Citation56]. The transition from water to terrestrial life was thought to be connected with frequently occurring abiotic stress scenarios like desiccation, freezing, as well as high photosynthetically active radiation (PAR) and ultraviolet (UV) radiation [Citation57], combined with the competition for nutrients and need to escape from poisons and predators, which also accompanied the aquatic life [Citation58]. Studies of the tolerance strategies of green algae and of Klebsormidium particularly revealed interesting survival mechanisms in vegetative or resting cells [Citation57, Citation59]. An example is the repeatedly shown self-protective role of their growth in mats or multi-layered biofilms, which ensures self-shading as a passive photoprotective mechanism [Citation31, Citation32, Citation36, Citation37] and contributes to the protection from drying through minimizing the individual water loss. The strategies to survive stress involve phytohormones, ultrastructural changes, biochemical pathways and various protective compounds which are of biotechnological and nanotechnological interest. Therefore, the present work provides for the first time a review on this yet neglected topic and describes the reliable identification using the polyphasic taxonomic approach of three local Klebsormidium strains isolated from soils in Bulgaria. These strains attracted our attention due to their high protein content (more than 30% dry weight - DW; data not shown) recorded during our ongoing experiments and their ability to form resting stages.
Materials and methods
Strains – collecting and cultivation
The present study was based on three Bulgarian strains of Klebsormidium. Two of them were isolated from samples collected in April 2007 in the protected area Kresnensko Defile, a gorge of the river Struma situated between the mountains Pirin and Malashevska Planina [Citation60]. The soil sampling was done by standard methods from the maroon forest soil with pH = 7 at one site at altitude of 227 m a.s.l., where strain ACUS 00001 was isolated from the sample taken at a depth of 2 cm and strain ACUS 00014 was isolated from the sample taken at 10 cm depth [Citation60]. The third strain (ACUS 00207) was isolated from a sample collected in Rila Mountain in August 2015, by direct collection [Citation61] from 10 cm depth at a site with mountain forest dark meadow soils, pH = 7.30, situated at 2132 m a.s.l. in the Rila National Park.
The algae were stored in the Algal Collection of Sofia University (ACUS) on BBM agar with subsequent isolation in clone cultures [Citation62]. The strains were identified by light microscopy (LM) after a short cultivation period (2–4 weeks). In January 2019, the algae were studied by molecular-genetic methods and checked again by LM after a long cultivation period (12 and 5 years, respectively). In parallel, part of the cultures was transferred to new agar and investigated by LM after 1 week of cultivation.
To grow sufficient biomass necessary for biochemical and molecular-genetic analyses, the strains were incubated in a bottle-type bioreactor with stirring and aeration (controlled aeration rate and CO2 concentration) in liquid BBM. This was done in the cultivation room of the AgroBioInstitute (ABI) of the Agricultural Academy of Bulgaria. The temperature was 22–23 °C and a 16/8 light/dark photoperiod was maintained with cool white fluorescent lamps [Citation1, Citation3].
Light microscopy (LM)
LM identification was done using a Motic BA400 microscope and the microphotographs were taken on the same microscope using a Moticam 2 camera and the Image Plus Program. The identification follows standard taxonomical guides [Citation10–12, Citation63–68]. To outline the pyrenoid, iodine solution was used [Citation10, Citation11] and to check for the presence/absence of a tiny gelatinous envelope around the filaments, the Indian ink was used [Citation12].
Phylogenetic analysis
For the purpose of the phylogenetic analysis, samples of the studied algal strains were taken after centrifugation of liquid cultures and storage of pellets at −80 °C. The frozen samples were dried and milled using Tissue Lyser II (Qiagen). Genomic DNA was isolated using GeneJET Plant Genomic DNA Purification Kit (Thermo Fisher Scientific). The ITS rDNA region was PCR amplified using forward ITS1 (5′-GTAGTCATATGCTTGTCTC) and reverse ITS4 (5′-CTTCCGTCAATTCCTTTAAG) primers [Citation69] with annealing at 53 °C. To amplify the region corresponding to the rbcL gene, the primers rbcLKF590 (5′GAT GAA AACGTA AAC TCT CAG C-3′) and rbcLKR2 (5′-GGT TGC CTT CGC GAG CTA-3′) [Citation49] were used. Following agarose gel electrophoresis, the amplified DNA fragments were purified according to the manufacture’s protocol (GeneJET Gel Extraction Kit, Thermo Fisher Scientific) and cloned into pJET1.2 plasmid vector, CloneJET PCR Cloning Kit (Thermo Fisher Scientific). Four clones from each strain were sequenced (Macrogen Inc.) and the DNA sequences were assembled using Vector NTI v. 10 software package (Thermo Fisher Scientific). The obtained ITS and rbcL sequences were compared to the known sequences by BLAST search, and phylogenetic analysis was conducted by MEGA version 4.1 [Citation70].
Review of the biotechnological potential of Klebsormidium
The present review is based on a few studies on the possibilities for commercial exploitation of Klebsormidium [Citation71–80] and on the current knowledge of its stress tolerance and bioindicator properties obtained in a series of eco-physiological, ecological, taxonomical and OMICS studies [Citation17, Citation31–34, Citation37, Citation46, Citation49, Citation50, Citation52, Citation53, Citation57, Citation59, Citation81–104]. These studies were conducted mainly with regard to the terrestrial colonization by algal land-plants progenitors and to the common mechanisms, genes, compounds and structures related to land-plants origins. However, the obtained results also reveal the valuable biotechnological and nanotechnological potential of the genus.
Klebsormidium phytohormones
Phytohormones are widely recognized to play a critical role in stress responses in plants; yet the data on their role and distribution in algae are quite scarce. Genomic studies of Klebsormidium flaccidum (strain NIES-2285, recently reclassified to K. nitens [Citation102]) revealed candidates for most of the genes required for biosynthesis of auxin, abscisic acid (ABA), cytokinin (CK) and jasmonic acid (JA), and genes predicted to encode counterparts of the plant hormone receptors ABP1 (auxin), GTG (ABA), GRE1 (CK) and ETR (ethylene) [Citation55]. Mass spectrometry proved the presence of auxin indole-3-acetic acid (Aux/IAA), ABA, the CK isopentenyladenine, JA and salicylic acid in this alga [Citation55]. The almost complete pathways in CK and ABA signalling, and ethylene response in Klebsormidium crenulatum subjected to strong desiccation stress, indicated that phytohormones and ABA in particular are implicated in the desiccation tolerance of Klebsormidium [Citation57, Citation97]. By contrast, the application of ABA did not change the freezing tolerance of K. flaccidum [Citation88].
Apart from the participation in stress reactions, phytohormones are important in many aspects of the regulation of growth and development in land plants [Citation105]. Genomic studies showed the presence of a set of auxin biosynthesis genes and their participation in growth regulation in some red, brown and green algae (from the phylum Chlorophyta). Recently, part of the auxin system that regulates transcription and cell growth (without the requirement of the central players that govern auxin signalling in land plants) was discovered in the green streptophyte species Klebsormidium nitens [Citation102].
Protective compounds in Klebsormidium – organic osmolytes and UV/PAR screeners
The studies on Klebsormidium protection against different environmental stresses (desiccation, freezing, strong insolation and PAR/UV radiation, hypertonic stress) revealed presence of two main types of protective compounds: organic osmolytes and UV/PAR screeners.
Organic osmolytes are chemically diverse, comprising sugars, polyols, amino acids and their derivatives [Citation106]. Besides controlling the cytoplasmic osmotic pressure, organic osmolytes as compatible solutes can protect cell proteins and stabilize cell membranes and can act as antioxidants, heat- or cryoprotectants. Being rapidly degraded substances, they can be used as respiratory substrates [Citation88, Citation107–109]. The main osmolytes of Klebsormidium (K. flaccidum, K. sterile, Klebsormidium sp.) in relation to freezing, desiccation and hypertonic salt-stress are sugars (mostly sucrose and glucose, as well as raffinose, xylose, mannose, inositol, fructose), sugar alcohols, or polyols (glycerol, sorbitol, mannitol), and amino acids (including the non-essential glutamic acid and g-aminobutyric acid/GABA/), and unknown glycosides [Citation31, Citation82, Citation88, Citation90]. Exceptionally, polyols are lacking in K. crenulatum and related species, which contain sucrose instead [Citation32, Citation88]. Although trehalose is strongly involved in desiccation tolerance in many biological systems [Citation108], in Klebsormidium it was found only in trace amounts [Citation90].
Most of the osmolyte compounds found in Klebsormidium, and the polyols in particular, are broadly used in different aspects of human life. Some important examples include the food industry, cosmetics and health care products as sweeteners and sugar substitutes in reduced-sugar foods, humectants and solvents, and helpers in food preservation (like cryoprotectants, e.g. [Citation71]); as well as medicine and pharmaceutics as medications like mannitol for lowering of the pressure in the eyes (as in glaucoma) or for lowering increased intracranial pressure [Citation110]. The biotechnological interest in natural osmolytes will doubtlessly increase since a study showed that they can be utilized as therapeutic targets for genetic diseases attributed to the problems associated with protein misfolding [Citation111]. Despite the low relative amount of osmolytes in Klebsormidium cells (ca. 1.2% DW according to [Citation90]), we have included them in the present review with the belief that this content could be regulated by cultural conditions and controlled relevant stoichiometry, as it has been proved for many other cell compounds of microalgae (e.g. [Citation112]).
The UV and PAR cell protection is strongly connected with the production and accumulation of mycosporine-like aminoacids (MAAs) [Citation113]. Apart from their sunscreen function, these low-molecular-weight water-soluble molecules are involved also in osmotic regulation as compatible solutes following salt stress and can act as novel antioxidants scavenging toxic oxygen radicals [Citation113, Citation114]. Their formation is induced by desiccation or by thermal stress in certain organisms, and they have been suggested to function as an accessory light-harvesting pigment in photosynthesis, and as an intracellular nitrogen reservoir [Citation113]. Currently MAAs are recognized as potential health and beauty ingredients [Citation115–117]. They are considered to be novel, fully safe and highly efficient natural UV-absorbing compounds that could be used in sun care products as a promising alternative which can effectively compete with commercially available filters for skin protection from UV radiation [Citation117]. MAAs can act also as a new wound healing agent and might be a novel biomaterial for wound healing therapies [Citation116]. MAAs are known as accumulating in different organisms, including prokaryotic and eukaryotic algae [Citation118] and, due to their function as a primary sunscreen to reduce short-wavelength light, are thought to have been greatly important to ancient forms of life on Earth [Citation114]. However, within Streptophyta their production has been proved only in three genera of Klebsormidiophyceae – Klebsormidium, Hormidiella and Interfilum [Citation37, Citation94, Citation98]. The MAAs found in Klebsormidiophyceae are not the same as other known MAAs like palythine, asterina-330, mycosporine-glycine, shinorin and porphyra-334 [Citation98]. The MAA in Klebsormidium and Interfilum has an absorption maximum (AM) at 324 nm and retention time (RT) of 4.5 min, unlike the MAA of Hormidiella (AM of 325 nm and RT of 6.5 min) – [Citation98], but similar to the putative MAA found in an earlier study of K. flaccidum [Citation37, Citation94]. Its steady-state content ranged broadly in the investigated strains, but showed a strong increase after UV exposure: an almost 4- and 11-fold increase [Citation37, Citation94, Citation98].
The dissipation of excess excitation energy as heat, called non-photochemical quenching (NPQ), is among the important mechanisms for avoiding oxidative damage. In algae, the NPQ-activation is related with LHCSR (Lhc-like protein Stress Related; previously called Li818, Light Induced protein 818), which was discovered in Klebsormidiales too [Citation91]. It differs from the NPQ-activation protein of land plants and of higher streptophyte algae like Charales, Coleochaetales and Zygnematales [Citation91].
Biotechnological potential of the biochemical and structural changes during drought, cold and heat-acclimation with emphasis on cell-wall composition and formation of resting stages in Klebsormidium
Current biotechnology is interested in both heat- and cold-acclimation mechanisms in regard to their possible application for better food production and preservation [Citation71, Citation119–121]. Besides the stress-protective compounds described above, many algae and land plants evolve protective structural and biochemical changes and develop stress-tolerant dormant cells, which are also of biotechnological interest. Biochemical and physiological changes (chlorophyll a content, fluorescence and viability test) of 12 Klebsormidium strains from different geographical regions showed their similar response to freezing and desiccation [Citation87]. However, there were variations in the desiccation tolerance and physiological responses of two species of Klebsormidium, coexisting in alpine soil crust communities of the Alps (K. dissectum and K. crenulatum), which were explained by differences in their morphological features [Citation17, Citation32–34, Citation37].
Several studies of cold acclimation of green algae revealed an increase of free fatty acids, starch and accumulation of group 3 late embryogenesis abundant proteins (which protect the freeze-labile protein lactate dehydrogenase) and lipid bodies [Citation88]. After desiccation of the drought-tolerant species Klebsormidium rivulare, its carbohydrate and lipid contents increased whereas the protein content decreased [Citation52]. Under different culture temperatures the fatty acid composition and protein content of the Antarctic Klebsormidium strain UMACC 227 varied in contrast to the carbohydrate content (which did not change markedly), although the freezing tolerance of the alga was not examined [Citation86]. After desiccation stress, electron-dense particles with diameters of 0.4–0.8 μm were observed in the cytoplasm of Klebsormidium crenulatum, likely representing lipid droplets [Citation33]. A study on the fatty acid composition of a benthic strain of Klebsormidium in different culture conditions indicated it as a potential source of polyunsaturated fatty acids (PUFAs) and of C18:2ω6 (5.4% of DW) in particular [Citation77].
Although the molecular mechanisms and pathways behind the stress tolerance of streptophyte algae are poorly investigated, a recent transcriptomic study of severe desiccation stress of K. crenulatum demonstrated that most of the highest upregulated transcripts do not show any similarity to known proteins [Citation93].
The broad tolerance to harsh conditions of Klebsormidium (which has low light requirements) is reflected in its growth patterns and photosynthetic activity and explains well its abundance in alpine soil crusts [Citation17, Citation32, Citation34, Citation37, Citation49] and can be outlined as advantageous for maintenance of algal cultures for biotech and nanotech purposes. Ultrastructural changes with decreased vacuole volume and increased cytoplasmic volume have been recorded. This may alter the distribution of compatible solutes in plant cells during cold acclimation (for details, see [Citation88]). The gene expression patterns of streptophyte algae in response to cold, drought and high light stress showed that some of the strongest differentially regulated genes were those associated with plastid biology and relevant ultrastructure [Citation102]. The significant role of ultrastructure in desiccation tolerance was suggested by Karsten et al. [Citation32] and afterwards the integrity of cellular organelles (like nucleus, plastid and mitochondria) together with the flexible cell walls able to regulate their shrinkage were shown to be important structural features in the desiccation tolerance of Klebsormidium [Citation34, Citation37, Citation92].
Mikhailyuk et al. [Citation95] demonstrated that Klebsormidium species differ in their ultrastructure and texture of cell walls. According to the review by Popper et al. [Citation89], the cell-wall polysaccharide composition reflects the terrestrialization, and Klebsormidiaceae contain cellulose in their cell wall together with arabinogalactan proteins and homogalacturonan [Citation89, ]. We note these cell wall components due to their economic value for paper, food and fiber industries, and for projected future use for biofuels, nutraceuticals and pharmaceuticals [Citation89]. However, further studies demonstrated that the cell wall of Klebsormidium lacks most of the epitopes and glycosidic linkages that are typical of the embryophyte wall [Citation122] and is relatively poor in cellulose but contains callose instead, which explains its flexibility upon desiccation [Citation33, Citation34, Citation92]. Callose is incorporated in repairing the local disruptions of the cell walls during severe water loss and allows walls to expand due to the turgor pressure, which may enhance fragmentation of the filaments [Citation96], typical of most species of Klebsormidium. Detachment of filaments in small and more metabolically active parts seems to be a fast and economical way to gain dispersal under changed environmental conditions, which logically explains the worldwide distribution of the genus [Citation50, Citation96, Citation100]. Earlier, Karsten and Holzinger [Citation34] showed that the steadily occurring fragmentation of filaments into smaller units in K. dissectum leads to improved self-protection and thus may represent a life strategy to better survive longer periods of drought in exposed alpine soil crusts. We could add that the fast defragmentation can be regarded also as a biotechnological advantage of Klebsormidium strains (including its strains presented in this study) comparable with the fast reproduction by autospores of the coccal green and yellow-green algae, used in microalgal mass cultures [Citation123].
Kondo et al. [Citation101] identified wax-related genes in K. flaccidum and investigated aliphatic substances in its cell wall fraction. They proved the presence of a cuticle-like hydrophobic layer composed of lipids and glycoproteins different from the cutin polymer typically found in land plants. The biotechnological interest lies in the bioconversion of aliphatic substances to products such as biopolymers and emulsifiers for cosmetics [Citation124].
Thickening of cell walls as adaptation to withstand desiccation was manifested in the aquatic Klebsormidium rivulare [Citation52]. Akinetes with thick, lamellate and sculptured cell walls were seen on a drying piece of BBM agar in an alpine strain of K. dissectum [Citation59]. Commonly these vegetative reproductive cells may function as resting stages enhancing survival under unfavourable environmental conditions [Citation58], but up to now they have been seldom documented in Klebsormidium ([Citation53, Citation59] and references therein). In addition, generally, the formation of akinetes may contribute to coping with enhanced UV radiation [Citation125]. We underline the ability of Klebsormidium to form such dormant cells since they provide a great biotechnological advantage, providing a possibility for long-term storage of commercially interesting strains in a viable state in normal air-dry conditions. Thus, considering the data on long-term algal survival in soils [Citation126], combined with the biochemical content of Klebsormidium described above and the important role of green algae in soil neogenesis [Citation127, Citation128], we propose the possibility to use inoculums of Klebsormisium akinetes for soil fertilization. This application is similar to the currently actively investigated and applied use of cyanoprokaryote akinetes in the algalization process designed by Venkataraman [Citation129–135]. The increasing interest in algae as soil biofertilizers and biostimulants in the biotechnological toolbox lies in the benefits which they have shown in the development of green agriculture and nature farming with possibilities for coupling with waste water treatment, bioremediation and energy production [Citation128]. Thus, algae play an important role in achieving a more ecofriendly approach for sustainable agriculture and healthy environment with economic profitability and socio-economic equity [Citation128].
Commercially valuable compounds and biofuel production from Klebsormidium
Searching for low-cost novel algal sources for biofuel, despite receiving contradictory evaluation, is among the hot topics of recent phycological studies and has been documented in countless papers and reviews [Citation2, Citation136]. It is widely known that the main investigated sources belong to the ecological group of aquatic algae and less to the aeroterrestrial algae. Current experiments based on 62 aeroterrestrial algae, including Klebsormidium, showed that it can be used for bioethanol production by growing in polluted or waste water and enhanced fermentation by the yeast Saccharomyces cerevisiae [Citation79]. Moreover, the same authors reported on a gradual increase of the protein content of Klebsormidium from the 3rd to the 21st day of anaerobic fermentation. Klebsormidium was amongst the top five of all studied algae and showed relatively high protein, amylase enzyme and total chlorophyll content [Citation80]. However, these data should be taken in a precautious way since the authors indicate presence of chlorophyll c in most studied green terrestrial algae in addition to the chlorophylls a and b! In the same Klebsormidium, high 2,2-diphenyl-1-picrylhydrazyl (DPPH) scavenging capacity and high activity of peroxidase (POX) and superoxide dismutase (SOD) reflected their role in protecting plastids from reactive oxygen species (ROS) and as protectors of cells against aging effects [Citation80]. A recent investigation on the localization and specificity of enzymes acting on hemicelluloses in different developmental stages of Klebsormidium discovered novel transglycosylation activities between xyloglucan and xylan, and xyloglucan and galactomannan with possible effect on the cell growth [Citation104].
Klebsormidium in water purification, phycoremediation and bioindication
Bioremediation based on algae is currently known as phycoremediation [Citation137]. Benthic filamentous algae have evident advantages in wastewater treatment over unicellular microalgae, including the ease in harvesting and resistance to predation [Citation78]. The growth of Klebsormidium in polluted and waste water [Citation80] led to quick reduction of water pH from alkaline to neutral and also to the reduction of total dissolved solids (TDS), which indicates that it is possible to use these algae in the phycoremediation of alkaline water. By contrast, the experimental application of Klebsormidium in the treatment of horticultural wastewater under natural conditions in Belgium was not promising [Citation78]. Some phycoremediation potential could be suggested for the Klebsormidium strain UMACC 227, which originally grows at a former oil spill site [Citation86]. Klebsormidium (K. klebsii) was tested together with other algae in the treatment of acid mine drainage (AMD), which is considered one of the most severe surface water pollutants [Citation76]. The study proved that the AMD-treatment by algae is a significant low-cost alternative to complex expensive treatment systems, but warns on its disadvantage associated with the release of bioaccumulated metals when algae start to die off. At the same time, the algae from such AMD-recovery treatment pools, if hazardous as a feed source, could be used for biofuel production [Citation76]. Klebsormidium (K. rivulare, Klebsormidium sp.) show tolerance to Zn and Cu, and ability to grow in sites with heavy metal pollution [Citation81, Citation83–85]. A comparative investigation of Klebsormidium-dominated algal mats in AMD-waters [Citation46] indicated that their snapshot studies may be a good indicator of iron concentration in water, but not for content of aluminum, zinc or magnesium.
Cellulose nanofibers (CNFs, also known as whiskers or nanocrystals) are natural, abundant polymers with excellent mechanical properties and biodegradability, which can be obtained from a range of renewable biosources (such as cotton, wood, hemp, tunicin, straw, algae and microorganisms) and therefore provide a new material platform for the sustainable production of high-performance nano-enable products for various applications [Citation99]. However, given the increasing rates of CNF production, the risk of their release to the environment and the subsequent impact on ecosystem is becoming an increasing concern [Citation99]. Cotton CNFs inhibit the algal proliferation and induce morphological changes through direct and indirect toxicity mechanisms, suggesting that K. flaccidum could serve as a bioindicator of terrestrial and freshwater pollution [Citation99].
Klebsormidium in green nanotechnology and nanobioremediation
Nanotechnology – integrating the areas of science and technology – generally refers to the processing, separation, consolidation and deformation of materials by an atom or molecule [Citation5]. As nanotechnology is expanding to several commercial fields, there is a need of ecofriendly and energy-efficient methods for the synthesis of its products having dimensions of 1 to 100 nm, named nanomaterials, or nanoparticles (NPs) [Citation5]. Metal NPs, due to their unique optical, thermal, electrical, chemical and physical properties, have a wide range of applications in different fields like medical imaging, drug delivery, electronics, nanocomposites, biolabelling, biocide or antimicrobial agents, sensors, non-linear optics, hyperthermia of tumours, intercalation materials for electrical batteries, optical receptors, catalysis, as well as in the modification of textiles with a focus on their anti-microbial properties [Citation138–140]. Gold NPs in particular are biocompatible and can be used in disease diagnosis and therapy [Citation138]. Detailed reviews on the use of algal resources for the biosynthesis of NPs and noble metal NPs in particular are provided in [Citation5, Citation132, Citation141]. Sicard et al. [Citation73] were the first to use silica-encapsulated K. flaccidum for the synthesis of gold NPs (10–15 nm in size). They were formed in the thylakoids and the extracellular polysaccharide network of the cell. Dahoumane et al. [Citation74, Citation75, Citation142] demonstrated the ability of some green algae to perform sustained synthesis of stable colloidal gold in cell-based bioreactors with special emphasis on the ability of K. flaccidum to adapt to the toxicity of gold cations and handle larger amounts of these cations [Citation74]. The intracellular globular gold NPs obtained by Dahoumane et al. [Citation74] were ca. 9 nm in size. Iron NPs have been synthesized by various chemical and physical methods, but currently some emerging methods of synthesis include the use of microorganisms and plant extracts which exclude the use of harmful chemicals and toxic byproducts [Citation138]. In this respect, K. flaccidum was useful in the biosynthesis of iron oxide since it promoted the intracellular production of β-akageneite (β-FeOOH) nanorods [Citation72]. These pioneer works on the nanotech application of Klebsormidiium are of great importance since this modern type of biosynthesis is considered as being of significant help to minimize the use of harmful chemicals and solvents and is simple, cost effective and time saving [Citation138]. Based on a large number of studies on the biosynthesis of NPs by different algae published in recent years, Sharma et al. [Citation5] concluded that the algae-mediated biosynthesis of NPs is an ecofriendly, economical, high-yielding, expeditious and energy-efficient method of green nanotechnology.
In the last two decades, NPs have been used in remediation as an alternative to existing treatment materials due to their efficiency, cost effectiveness and eco-friendly nature [Citation138]. Iron NPs are considered the first ones to be used in environmental clean-up with various technologies currently under development. The potential of gold NPs in the degradation of organic dyes has been analyzed. Some recent hybrid technologies like nanobioremediation, or the use of NPs to remove pollutants by enhancing microbial activity, are significant in transforming and detoxifying pollutants which harm the ecosystem [Citation138]. In this aspect the role of NPs biosynthesized using plant extracts, bacteria, fungi and algae is increasing and it is believed that ecofriendly, green synthesis of NPs coupled with remediation can go a long way in promoting sustainability [Citation138].
Polyphasic identification of three Klebsormidium strains, isolated from soils in Bulgaria
Morphological description and identification by light microscopy (LM)
Strains ACUS 00001 and ACUS 00014
Filaments are short, straight or slightly bent, usually dissociating into single or few-celled fragments which mostly achieve moniliform view by ageing due to constricted transversal cell walls () or have been seen as hooked due to fast defragmentation. Cells are 5–6 × 10–12 µm, cylindrical to barrel-shaped (doliform) with larger dimensions reached just before division (). The cell wall looks rigid and thick (). The plastid is parietal, trough-like, encircling half to two-thirds of the cell circumference (). There is one pyrenoid, globose to elongated in shape, with multiple starch grains, relatively large but better visible in older cultures or after staining. Reproduction runs by division of cells into two daughter cells or by disintegration of the filaments, often to larger broadly elliptical cells (). Zoospores have not been seen. Formation of numerous pre-akinetes with lipid droplets concentrated below the cell walls was typical of old (12-year) cultures on BBM agar (). Quite rarely, in the slides with old ACUS 00014, there were large elongated doliform cells (12–15 × 18–20(25–30) µm), which tentatively resembled the akinetes formed on dry BBM agar by the strain of K. dissectum collected from Pirin Mountain [Citation59]. There was one obvious difference between the ageing and the old cultures of both strains, namely the easier defragmentation of ACUS 00001 () in the ageing culture compared to the main development of ACUS 00014 in a filamentous stage ().
Figure 1. LM microphotographs of old culture material of strain ACUS 00001 (a, b) and of strain ACUS 00014 (c, d). Note: Scale bar is indicated on each microphotograph.
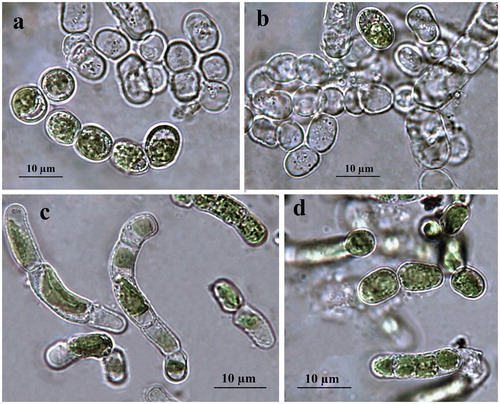
The initial refreshed cultures observed in January 2019 after one week of cultivation showed almost no difference between the two strains collected from two different depths (). There, algae were seen mainly in single (initial) cells (8–10 × 16–21 µm) with thick cell walls, or in stages of two cells (9–10 × 16–17 µm) (), while filaments were extremely rare (). These young filaments (cells 5 × 7 µm) were with non-constricted cells (). The apical-basal polarity of growing filaments (), and H-shaped cell-wall parts were well-visible ().
Figure 2. LM microphotographs of fresh culture material of strain ACUS 00001 (a–c). Note: Scale bar is indicated on each microphotograph.
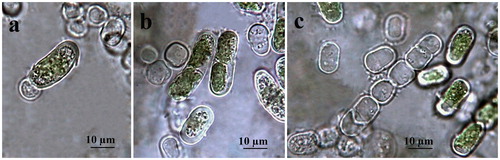
Figure 3. LM microphotographs of old culture material of strain ACUS 00014 (a–d). Note: Scale bar is indicated on each microphotograph.
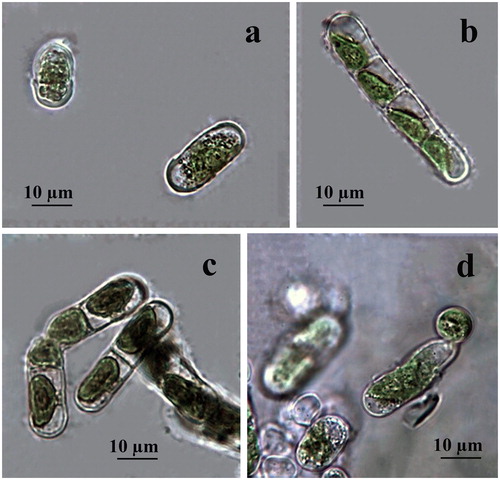
Figure 4. LM microphotographs of old culture material of strain ACUS 00207 (a–d). Note: Scale bar is indicated on each microphotograph.
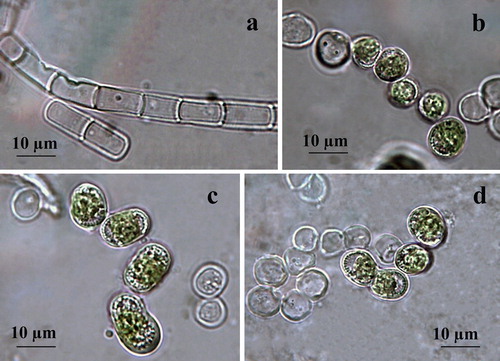
Both strains were identified as belonging to K. dissectum (F. Gay) Ettl et Gärtner in 2007–2009 [Citation60], which was confirmed after observations on old and young cultures made in January 2019 for this study. The main reason were the cell shape and dimensions (short cells in filaments, no more than 1.5 times longer than wide), thick cell walls with H-parts and constrictions in ageing filaments, as well as the easy defragmentation with formation of rounded apical cells and the apical-basal polarity of growing filaments. Staining with Indian ink did not reveal any mucilage envelope around the filaments.
Strain ACUS 00207
Filaments are short, mainly straight, not constricted at cell walls and usually dissociating into single, or few-celled fragments (). Cells are (5–8)-9-10 × 10–14(15) µm long, cylindrical with broadly rounded ends with larger dimensions reached just before division. The cell wall looks thin when cells are joined in the filaments (), and gets thicker when disintegrating occurs or resting cells are formed (). The plastid is parietal, trough-like covering two-thirds or less of the cell circumference (). There is one pyrenoid, globose to elongated with multiple starch grains, better visible after staining (). Reproduction runs by division of cells into two daughter cells, or, more often, by disintegration of the filaments, often to larger broadly elliptical or more spherical cells (). Zoospores were not seen, but some typical openings in empty filamentous cells, despite being rare, indicate their possible formation in vegetative cells in the old cultures observed in January 2019 (). Formation of numerous pre-akinetes with lipid droplets concentrated below the cell walls was observed in the same old cultures on BBM agar (). The presence of similar broadly elliptical large cells with numerous shining droplets of reserve products in old cultures were outlined by Moshkova and Gollerbakh [Citation65]. Staining with Indian ink did not reveal any mucilage envelope around the filaments.
Figure 5. LM microphotographs of fresh culture material of strain ACUS 00207 (a–d). Note: Material on (c) is stained by iodine solution and an arrow points at the pyrenoid. Scale bar is indicated on each microphotograph.
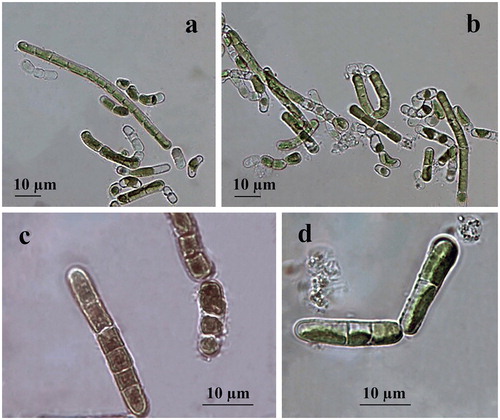
Immediately after collection, the strain was tentatively identified as K. dissectum, but noting many similarities to K. klebsii (G. M. Smith) P. C. Silva, K. R. Mattox et W. H. Blackwell, especially based on overlapping of cell dimensions and shapes. Since Hormidium nitens was taken as a synonym of K. klebsii by Silva et al. [Citation143], its closest species – Klebsormidium nitens (Kützing) Lokhorst, was also checked. However, Lokhorst [Citation12] left out of consideration K. klebsii. The comparison of diagnoses and descriptions of both species (K. klebsii and K. nitens) in the taxonomic literature showed almost total overlapping of their morphological features. Therefore, we decided to postpone the identification of ACUS 00207, with hesitation between K. dissectum and K. klebsii, due to the lack of long filaments pointed out as typical of the species synonyms Hormidium nitens Meneghini emend. Klebs in Printz [Citation63] and Chlorhormidium flaccidum (Kützing) Fott var. nitens Meneghini emend. Klebs in Starmach [Citation64] and Moshkova & Gollerbakh [Citation65]. After observations of the old cultures in January 2019 prepared for this study, we identified the strain as K. klebsii mainly due to its cell shape and dimensions with longer cells in the filaments (1.5–2 times longer than wide), lack of constrictions, lack of H-parts, thin cell walls, easy defragmentation and trend to form resting stages. The hesitation between K. nitens and K. klebsii was expressed in the morphological descriptions by Rindi et al. [Citation16], who pointed out the presence of a small pyrenoid, constrictions and H-parts in K. nitens. This contradicts with the species description given by Lokhorst [Citation12]. The presence of constrictions, but ‘usual’ absence of H-parts was indicated also by Mikhailyuk et al. [Citation39] in their description of K. nitens and proposal of strain SAG 19.31 as its epitype. This proposal was rejected by Rindi et al. [Citation144], who proposed their sample F1B (=culture CAUP J 306) as an appropriate candidate of this species. Unfortunately, the last study did not take into account K. klebsii. It has to be underlined, that in the description of the new proposed epitype for K. nitens, Rindi et al. [Citation144] noted the lack of constrictions and H-parts.
In the young cultures made in January 2019, we saw mainly filaments which disintegrate easily (). In these young filaments, some cells were short, but this could be due to fast division (). Cells were 5-6-8 × 10-13-14 µm. Although some cells were with well-developed and relatively thick cell wall, H-parts were not observed. According to the last observations, we decided to identify strain ACUS 00207 as K. klebsii.
Phylogenetic position of the studied Klebsormidium strains
The results from the phylogenetic analysis performed using ITS rDNA and rbcL sequences ( ), showed that all the three studied strains belong to the main superclade E of the genus Klebsormidium [Citation16, Citation21, Citation39, Citation144]. This superclade initially consisted of the strains of its most widespread species K. flaccidum, K. fluitans, K. dissectum and K. nitens [Citation16], but later, K. flaccidum with its strains was separated in clade B [Citation39], or B and C [Citation144]. This separation was in conformity with earlier ITS results by Sluiman et al. [Citation23], who pointed out the early phylogenetical divergence of K. flaccidum by contrast with K. fluitans, K. dissectum and K. nitens, which formed an ‘unresolved clade’. The polyphyletic character of K. nitens and K. dissectum with occurrence in different lineages (based on ITS+rbcL), was noted also by Škaloud and Rindi [Citation21]. However, despite that ‘in the last 10 years, molecular data on Klebsormidium have been produced in plentiful amounts’ [Citation144, p.79], K. klebsii remained out of the studies.
Figure 6. Neighbour joining phylogenetic tree of ITS sequences constructed from studied ACUS strain sequences and their closest homologous sequences retrieved after BLAST search. Bootstrap values greater than 50% confidence are shown at branching points (percentage of 1000 resamplings). Sequence accession numbers are shown in brackets.
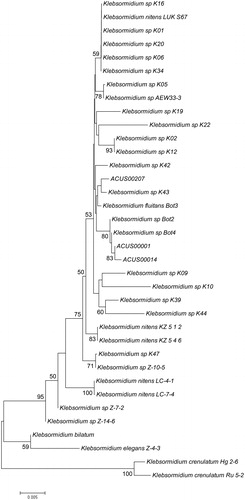
Figure 7. Neighbour joining phylogenetic tree of rbcL sequences constructed from studied ACUS strain sequences and their closest homologous sequences retrieved after BLAST search. Bootstrap values greater than 50% confidence are shown at branching points (percentage of 1000 resamplings). Sequence accession numbers are shown in brackets.
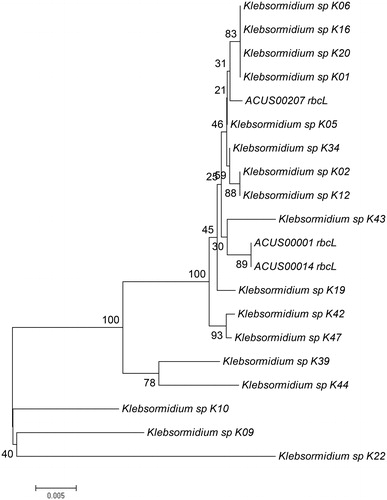
Figure 8. Neighbour joining phylogenetic tree of concatenated ITS and rbcL sequences constructed from studied ACUS strain sequences and their closest homologous sequences retrieved after BLAST search. Bootstrap values greater than 50% confidence are shown at branching points (percentage of 1000 resamplings). Sequence accession numbers are shown in brackets.
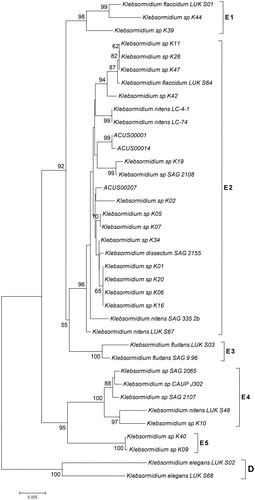
The topology of our phylogenetic trees based on ITS and rbcL sequences analyzed as single markers or in combination () is generally in accordance with the earlier works cited above [Citation16, Citation21, Citation39, Citation144]. Despite some variations in the detailed topology, in all trees the two strains from Kresnensko Defile (ACUS 00001 and ACUS 00014) grouped close to each other, while the Rila strain (ACUS 00207) were more distant. In the ITS rRNA-tree (), ACUS 00001 and ACUS 00014 grouped with K. dissectum strains Bot2 and Bot4 [Citation39], while ACUS 00207 was near to Klebsormidium sp. K43 (which, according to ITS+rbcL, is close to K. nitens SAG13.91 in [Citation21] and to K. flacccidum SCCA011, K. flaccidum SCCA009 and K. nitens SAG13.91 in [Citation48]). In the phylogenetic tree based on rbcL (), ACUS 00207 is clearly grouped with the Klebsormidium strains K01, K06, K16 and K20 (all relevant to K. nitens SAG 52.91 [Citation21] and K01 and K06 close to the same strain and K. dissectum SAG 2155 in [Citation144]), but not with the above-mentioned Klebsormidium sp K43. In the rbcL-tree, Klebsormidium sp. K43 strain was grouped with ACUS 00001 and ACUS 00014, thus contrasting with the results obtained from the ITS-based tree. However, in the topology based on combined ITS and rbcL, all three strains grouped nearer to the strains identified as K. nitens, except strain K. dissectum SAG 2155 (). Albeit, seemingly controversial at first glimpse, these results are in conformity with the generally close positions of these two species in the E superclade, and in the clade E1 in particular [Citation144], with intra-clade variations when the trees use single-marker datasets of ITS rDNA and of rbcL, or are concatenated with both genes [Citation16, Citation21, Citation39, Citation48, Citation144]. This shows their close molecular-genetic characteristics. These data also reflect the quite similar morphology of both species K. dissectum and K. nitens revealed after careful reading of taxonomic descriptions [Citation10–12, Citation16, Citation39, Citation63–68, Citation144].
Since not all the strains from the literature data have both ITS and rbcL sequences, considering the results from LM morphological observations, at the present state-of-art we refer ACUS 00001 and ACUS 00014 as belonging to K. dissectum, and ACUS 00207 (identified morphologically as K. klebsii) as belonging to K. nitens. What is more, the relevance of the Rila strain to K. nitens was also confirmed by the rbcL-tree (). The final result for different identification of Rila and Kresnensko Defile strains, is in conformity with the ecological evaluations and conclusions of Mikhailyuk et al. [Citation39], who showed some clustering in the distribution of Klebsormidium morphotypes and lineages along the elevation gradient. Accordingly, K. dissectum was collected only at lower altitudes, and K. nitens and K. cf. nitens with its morphotypes occurred at middle and high elevations [Citation39].
Based on the morphological and molecular data, we could tentatively suggest that there is similarity between K. klebsii and K. nitens. At the same time, the overlapping morphological data reported by different authors [Citation10–12, Citation16, Citation39, Citation63–68, Citation144] and earlier grouping of K. nitens and K. dissectum with K. flaccidum as its varieties or forms [Citation64, Citation65] combined with the strong intra-clade variation of various strains identified as K. nitens and K. dissectum, indicate the need for further deeper elucidation of the taxonomy of these yet problematic species. Therefore, we agree with the opinion of Rindi et al. [Citation16, Citation144] and propose further more detailed polyphasic investigations of a broader palette of cultures collected at a global scale with more detailed and targeted morpho-ecological observations in order to resolve the taxonomy of Klebsormidium.
Conclusions
The results obtained from intensive literature search and our professional experiences indicated the valuable biotechnological and nanotechnological potential of the green filamentous algal genus Klebsormidium with possible utilizations in different human affairs (food, medicine, pharmaceutics, cosmetics, water treatment and remediation, bioindication, etc.). In particular, the strong stress-resistance and easy defragmentation combined with possibility to produce resting cells (pre-akinetes and akinetes) are cultivation advantages in the future use of this streptophyte genus. Together with its biochemical composition, these advantages provide a reason for possible implementation of Klebsormidium as a soil biofertilizer. The broad palette of applications supports the need for phycoprospecting of Klebsormidium species based on reliable polyphasic identification. In this respect, the morphological identification of three Klebsormidium strains capable of pre-akinete formation, isolated from soils in protected areas in Bulgaria and kept in the Algal Collection of Sofia University (ACUS), was supported by molecular methods, which showed their phylogenetic position. In this way, using the polyphasic approach, two strains were identified as belonging to the species K. dissectum (ACUS 00001 and ACUS 00014), while the strain ACUS 00207 was identified as K. klebsii and its similarity with K. nitens was supposed.
Disclosure statement
No potential conflict of interest was reported by the authors. Ralitsa Atanasova is working voluntarily in her free-time in preparing a thesis аs a PhD student on a self-study basis with the Group of Systematics of Algae and Fungi at the Department of Botany of Sofia University.
Additional information
Funding
References
- Stoykova P, Stoyneva-Gärtner M, Draganova P. Morphological characterization and phylogenetic analysis of strains from Vischeria/Eustigmatos group with potential industrial utilizations. Biotechnol Biotechnol Equip. 2019.
- Borowitzka MA. Algal biotechnology. In: Sahoo D, Seckbach J, editors. The Algae World. Dordrecht: Springer; 2015. p. 319–338.
- Stoyneva-Gärtner M, Uzunov B, Gärtner G, Borisova C, Draganova, Radkova M, Stoykova P & Atanassov, I. Carotenoids in five aeroterrestrial strains from Vischeria/Eustigmatos group: Updating the pigment pattern of Eustigmatophyceae. Biotechnol Biotechnol Equip. 2019. DOI:10.1080/13102818.2019.1573154
- Stoyneva-Gärtner MP, Stoykova P, Uzunov B. Current bioeconomical interest in stramenopilic eustigmatophytes: a review. Biotechnol Biotechnol Equip. 2019;1–13. Forthcoming.
- Sharma A, Sharma S, Sharma K, et al. Algae as crucial organisms in advancing nanotechnology: a systematic review. J Appl Phycol. 2016;28:1759–1774.
- Kim S-K, editor. Handbook of marine macroalgae: biotechnology and applied phycology. Chichester (UK): Wiley; 2011.
- Mazarassa I, Olsen YS, Mayol E, et al. Rapid growth of seaweed biotechnology provides opportunities for developing nations. Nat Biotechnol. 2013;31:591–592.
- Sharma N, Sharma P. Industrial and biotechnological applications of algae: A review. JAPB. 2017;1:1–4.
- Rasul I, Azeem F, Siddique MH, et al. Chapter 8 - Algae biotechnology: a green light for engineered algae. In: Zia KM, Zuber M, Ali M, editors. Algae based polymers, blends, and composites. Chemistry, Biotechnology and Material Sciences. Amsterdam: Elsevier; 2017. p. 301–334.
- Ettl H, Gärtner G. Syllabus der Boden-, Luft- und Flechtenalgen. Stuttgart: Gustav Fischer; 1995.
- Ettl H, Gärtner G. Syllabus der Boden-, Luft- und Flechtenalgen. 2. Auflage. Berlin: Springer Spektrum; 2014.
- Lokhorst GM. Comparative taxonomic studies on the genus Klebsormidium (Charophyceae) in Europe. Cryptogam Stud. 1996;5:1–132.
- Ryšánek D. Terrestrial algae of the genus Klebsormidium (Streptophyta) in the light of the hypothesis “Everything is everywhere, but the environment selects” [dissertation]. Prague: Charles University; 2016.
- Karsten U, Herburger K, Holzinger A. Photosynthetic plasticity in the green algal species Klebsormidium flaccidum (Streptophyta) from a terrestrial and a freshwater habitat. Phycologia. 2017;56:213–220.
- Büdel B, Darienko T, Deutschewitz K, et al. Southern African biological soil crusts are ubiquitous and highly diverse in drylands, being restricted by rainfall frequency. Microb Ecol. 2009;57:229–247.
- Rindi F, Mikhailyuk T, Sluiman H, et al. Phylogenetic relationships in Interfilum and Klebsormidium (Klebsormidiophyceae, Streptophyta). Mol Phylogenet Evol. 2011;58:218–231.
- Karsten U, Herburger K, Holzinger A. Living in biological soil crust communities of African deserts—physiological traits of green algal Klebsormidium species (Streptophyta) to cope with desiccation, light and temperature gradients. J Plant Physiol. 2016;194:2–12.
- Zhu H, Li S, Hu Z, et al. Molecular characterization of eukaryotic algal communities in the tropical phyllosphere based on real-time sequencing of the 18S rDNA gene. BMC Plant Biol. 2018;18:365–374.
- Ryšánek D, Elster J, Kováčik L, et al. Diversity and dispersal capacities of a terrestrial algal genus Klebsormidium (Streptophyta) in polar regions. FEMS Microbiol Ecol. 2016; 92:fiw039.
- Škaloud P. Variation and taxonomic significance of some morphological features in European strains of Klebsormidium (Klebsormidiophyceae, Streptophyta). Nova Hedw. 2006;83:533–550.
- Škaloud P, Rindi F. Ecological differentiation of cryptic species within an asexual protist morphospecies: a case study of filamentous green alga Klebsormidium (Streptophyta). J Eukaryot Microbiol. 2013;60:350–362.
- Civáň P, Foster PG, Embley MT, et al. Analyses of charophyte chloroplast genomes help characterize the ancestral chloroplast genome of land plants. Genome Biol Evol. 2014;6:897–911.
- Sluiman H, Guihal C, Mudimu O. Assessing phylogenetic affinities and species delimitations in Klebsormidiales (Streptophyta): Nuclear-encoded rDNA phylogenies and its secondary structure models in Klebsormidium, Hormidiella, and Entransia(1). J Phycol. 2008;44:183–195.
- Turmel M, Otis C, Lemieux C. Tracing the evolution of streptophyte algae and their mitochondrial genome. Genome Biol Evol. 2013;5:1817–1835.
- Frahm J-P. Epiphytische Massenvorkommen der fädigen Grünalge Klebsormidium crenulatum (Kützing) Lokhorst im Rheinland. Decheniana (Bonn). 1999;152:117–119.
- Türk R, Gärtner G. Biological soil crusts in the subalpine, alpine, and nival areas in the Alps. In: Belnap J, Lange OL, editors. Biological soil crusts: structure, function and management. Berlin: Springer; 2001. p. 67–73.
- Lim AS, Lee OM. The distribution of aerial algae and evaluation of algal inhabitation on five stone cultural properties in Gyeonggi-do. Algae. 2008;23:269–276.
- Rindi F, Guiry MD, López‐Bautista JM. Distribution, morphology and phylogeny of Klebsormidium (Klebsormidiales, Charophyceae) in urban environments in Europe. J Phycol. 2008;44:1529–1540.
- Uzunov B, Stoyneva MP, Gärtner G. Review of the studies on aero-terrestrial cyanoprokaryotes and algae in Bulgaria with a Checklist of the recorded species. I. Phytol Balc. 2007;13:65–73.
- Uzunov B, Stoyneva MP, Gärtner G. Review of the studies on aero-terrestrial cyanoprokaryotes and algae in Bulgaria with a Checklist of the recorded species. II. Phytol Balc. 2008;14:11–18.
- Karsten U, Rindi F. Ecophysiological performance of an urban strain of the aeroterrestrial alga Klebsormidium sp. (Klebsormidiales, Klebsormidiophyceae). Eur J Phycol. 2010;45:426–435.
- Karsten U, Lütz C, Holzinger A. Ecophysiological performance of the aeroterrestrial green alga Klebsormidium crenulatum (Charophyceae, Streptophyta) isolated from an alpine soil crust with an emphasis on desiccation stress. J Phycol. 2010;46:1187–1197.
- Holzinger A, Lütz C, Karsten U. Desiccation stress causes structural and ultrastructural alterations in the aeroterrestrial green alga Klebsormidium crenulatum (Klebsormidiophyceae, Streptophyta) isolated from alpine soil crust. J Phycol. 2011; 47:591–602.
- Karsten U, Holzinger A. Light, temperature and desiccation effects on photosynthetic activity, and drought-induced ultrastructural changes in the green alga Klebsormidium dissectum (Streptophyta) from a high alpine soil crust. Microb Ecol. 2012;63:51–63.
- Tran TH, Govin A, Guyonnet R, et al. Influence of the intrinsic characteristics of mortars on bifouling by Klebsormidium flaccidum. Int Biodeterior Biodegrad. 2012;70:31–39.
- Karsten U, Pröschold T, Mikhailyuk T, et al. Photosynthetic performance of different genotypes the green alga Klebsormidium sp. (Streptophyta) isolated from biological soil crusts in the Alps. Algo Stud. 2013;142:45–62.
- Karsten U, Holzinger A. Green algae in alpine biological soil crust communities: acclimation strategies against ultraviolet radiation and dehydration. Biodivers Conserv. 2014;23:1845–1858.
- Hallmann C. Biodiversity of terrestrial algal communities from soil and air-exposed substrates using a molecular approach [dissertation]. Göttingen: Georg-August-University School of Science (GAUSS). 2015.
- Mikhailyuk T, Glaser K, Holzinger A, et al. Biodiversity of Klebsormidium (Streptophyta) from alpine biological soil crusts (Alps, Tyrol, Austria, and Italy). J Phycol. 2015;51:750–767.
- Ragon M, Fontaine MLC, Moreira D, et al. Different biogeographic patterns of prokaryotes and microbial eukaryotes in epilithic biofilms. Mol Ecol. 2012;21:3852–3868.
- Drongová Z, Kováčik L. Some soil cyanobacteria and algae of the Záhorská Nížina lowland (Southwest Slovakia). Acta Bot Univ Comenianae. 2011;46:41–48.
- Tschaikner A. Soil algae and soil algal crusts in the alpine regions of Tyrol (Ötztal, Austria). [dissertation]. Innsbruck: University of Innsbruck; 2008.
- Stoyneva M, Mancheva A, Gärtner, et al. Are the algae from the uncommon Belogradchik rocks common ones? In: Petrova A, editor. Proceedings of the VII National Conference in Botany; 2011 29-30 September; Sofia. Sofia, Bulgarian Botanical Society; 2012. p. 265–269. Bulgarian.
- Mancheva AD. [Investigation of aerophytic algae from the natural landmark Belogradchik rocks] [dissertation]. Sofia (Bulgaria): Sofia University “St Kliment Ohridski”; 2013. Bulgarian.
- Stoyneva MP. Contribution to the knowledge on the biodiversity of hydro- and aerobiontic prokaryotic and eukaryotic algae in Bulgaria [D.Sc.thesis]. Sofia: Sofia University “St Kliment Ohridski”; 2014. Bulgarian.
- Stevens AE, McCarthy BC, Morgan LV. Metal content of Klebsormidium-dominated (Chlorophyta) algal mats from acid mine drainage waters in Southeastern Ohio. J Torrey Bot Soc. 2001;128:226–233.
- Novis PM. Taxonomy of Klebsormidium (Klebsormidiales, Charophyceae) in New Zealand streams and the significance of low-pH habitats. Phycologia. 2006;45:293–301.
- Škaloud P, Lukešová A, Malavasi V, et al. Molecular evidence for the polyphyletic origin of low pH adaptation in the genus Klebsormidium (Klebsormidiophyceae, Streptophyta). Plecevo. 2014;147:333–345.
- Pierangelini M, Ryšánek D, Lang I, et al. Terrestrial adaptation of green algae Klebsormidium and Zygnema (Charophyta) involves diversity in photosynthetic traits but not in CO2 acquisition. Planta. 2017;246:971–986.
- Herburger K, Karsten U, Holzinger A. Entransia and Hormidiella, sister lineages of Klebsormidium (Streptophyta), respond differently to light, temperature, and desiccation stress. Protoplasma. 2016;253:1309–1323.
- Mattox KR. Zoosporogenesis and resistant-cell formation in Hormidium flaccidum. In: Parker BC, Brown Jr RM, editors. Contributions in Phycology. Lawrence Kanes: Allen Press; 1971. p. 137–144.
- Morison MO, Sheath RG. Response to desiccation stress by Klebsormidium rivulare (Ulotrichales, Chlorophyta) from a Rhode Island stream. Phycologia. 1985;24:129–145.
- Segečová J, Elster J, Kováčik L. Filamentous green alga Klebsormidium flaccidum as a laboratory model for the study of dormancy. Acta Bot Univ Comen. 2011; 46:49–103.
- Lee JW, Kim GH. Isolation and characterization of two phototropins in the freshwater green alga, Spirogyra varians (Streptophyta, Zygnematales). Algae. 2017;32:235–244.
- Hori K, Maruyama F, Fujisawa T, et al. Factors for plant terrestrial adaptation. Nat. Commun. 2014;5:1–9.
- Becker B, Marin B. Streptophyte algae and the origin of embryophytes. Ann Bot. 2009;103:999–1004.
- Holzinger A, Pichrtová M. Abiotic stress tolerance of charophyte green algae: new challenges for OMICS techniques. Front Plant Sci. 2016;7:art.678.
- Colleman A. The role of resting spores and akinetes in chlorophyte survival. In: Fryxell GA, editor, Survival strategies of the algae. Cambridge: Cambridge University Press; 1983. p. 1–22.
- Uzunov BA, Gärtner G, Stoyneva MP. Notes on the akinete-forming strain of the green alga Klebsormidium dissectum (Streptophyta) from Pirin Mts., Bulgaria. Phyton - Ann Rei Bot. 2012b;52:139–144.
- Uzunov BA. Aeroterrestrial algae from Pirin Mountain (Bulgaria) [dissertation]. Innsbruck: University of Innsbruck; 2009.
- Gärtner G, Stoyneva MP, Mancheva AD, et al. A new method in collection and cultivation of aerophytic and endolithic algae. Ber Nat Med Ver Innsbruck. 2010;96:27–34.
- Uzunov B, Stoyneva M, Mancheva A, et al. [ACUS – the new collection of living aeroterrestrial algae of Sofia University “St. Kliment Ohridski”]. In: Petrova A, editor. Proceedings of the VII National Conference in Botany; 2011 29–30 September; Sofia. Sofia, Bulgarian Botanical Society; 2012. p. 271–274. Bulgarian.
- Printz H. Die Chaetophoralen der Binnengewässer. Hydrobiologia. 1964;24:1–376.
- Starmach K. [Chlorophyta III. Zielenice Nitkowate: Ulothrichales, Ulvales, Prasiolales, Sphaeropleales, Cladophorales, Chaetophorales, Trentepohliales, Siphonales, Dichotomosiphonales (with keys for the identification of filamentous green algae mentioned in this volume)]. In: Starmach K, Siemińska J, editors. Flora Słodkowodna Polski. Warszawa-Kraków: PWN; 1972. Polish.
- Moshkova NA, Gollerbakh MM. Chlоrophyta: Ulotrichophyceae, 1. Ulotrichales. Vol. 10(1). In: Vinogradova KL, editor. [Classification key of the freshwater algae of USSR] Leningrad: Nauka; 1986. Russian.
- Hindák F. Key to the unbranched filamentous green algae (Ulotrichiniae, Ulotrichales, Chlorophyceae). Bull Slov Bot Spoločnosti SAV. Suppl. 1996;1:1–77.
- John DM, Whitton BA, Brook AJ, editors. The freshwater algal flora of British Isles. An identification guide to freshwater and terrestrial algae. Cambridge: Cambridge Univeristy Press; 2002.
- John DM, Whitton BA, Brook AJ, editors. The freshwater algal flora of British Isles. An identification guide to freshwater and terrestrial algae. Second edition. Cambridge: Cambridge Univeristy Press; 2011.
- et al. White TJ, Bruns TD, Lee SB. Amplification and direct sequencing of fungal ribosomal RNA Genes for phylogenetics, In: Innis MA, Gelfand DH, Sninsky JJ, et al. editors. PCR protocols: a guide to methods and applications. London: Academic Press; 1990. p. 315–322.
- Tamura K, Dudley J, Nei M, et al. MEGA4: Molecular Evolutionary Genetics Analysis (MEGA) software version 4.0. Mol Biol Evol. 2007;24:1596–1599.
- Medina JR, Garrote RL. The effect of two cryoprotectant mixtures on frozen surubí surimi. Braz J Chem Eng. 2002;19:419–424.
- Brayner R, Yéprémian C, Djediat C, et al. Photosynthetic microorganism-mediated synthesis of akaganeite (beta-FeOOH) nanorods. Langmuir. 2009;25:10062–10067.
- Sicard C, Brayner R, Margueritat J, et al. Nanogold biosynthesis by silica-encapsulated micro-algae: a living biohybrid material. J Mater Chem. 2010;20:9342–9347.
- Dahoumane AS, Djédiat C, Yéprémian C, et al. Recycling and adaptation of Klebsormidium flaccidum microalgae for the sustained production of gold nanoparticles. Biotechnol Bioeng. 2012;109:284–288.
- Dahoumane AS, Djédiat C, Yéprémian C, et al. Species selection for the design of gold nanobioreactor by photosynthetic organisms. J Nanopart Res. 2012b;14:883–900.
- Oberholster P, Cheng P-H, Botha AM, et al. The potential of selected microalgal species for treatment of AMD at different pH values in temperate regions. Water Res. 2014;60:89–92.
- Liu J, Vanormelingen P, Vyverman W. Fatty acid profiles of four filamentous green algae under varying culture conditions. Biores Technol. 2016;200:1080–1084.
- Liu J, Danneels B, Vanormelingen P, et al. Nutrient removal from horticultural wastewater by benthic filamentous algae Klebsormidium sp., Stigeoclonium spp. and their communities: From laboratory flask to outdoor Algal Turf Scrubber (ATS). Water Res. 2016;92:61–68.
- Manoj BS, Chavan M, Ma S, et al. Phycoremediate terrestrial microalgae serve source for bioethanol. Int J Curr Microbiol Appl Sci. 2018;7:3174–3185.
- Manoj BS, Sushma Chavan M, et al. Western Ghats terrestrial microalgae serve as a source of amylase and antioxidant enzymes. J Pharmacogn Phytochem. 2018;7:1555–1560.
- Say PJ, Diaz BM, Whitton BA. Influence of zinc on lotic plants. I. Tolerance of Hormidium species to zinc. Freshwater Biol. 1977;7:357–376.
- Brown LM, Hellebust JA. The contribution of organic solutes to osmotic balance in some green and eustigmatophyte algae. J Phycol. 1980;16:265–270.
- Say PJ, Whitton BM. Changes in flora down a stream showing a zinc gradient. Hydrobiologia. 1981;76:255–262.
- Takamura N, Kasai F, Watanabe MM. Effects of Cu, Cd and Zn on photosynthesis of freshwater benthic algae. J Appl Phycol. 1989;1:39–52.
- Skowrońsky T, Klainowska R, Pawlik-Skowrońska B. [Algae in heavy metal polluted environments]. Kosmos. 2002;51:165–173. Polish
- Teoh ML, Chu WL, Harvey M, et al. Influence of culture temperature on the growth, biochemical composition and fatty acid profiles of six Antarctic micro algae. J Appl Phycol. 2004;16:421–430.
- Elster J, Degma P, Kováčik L, et al. Freezing and desiccation injury resistance in the filamentous green alga Klebsormidium from the Antarctic, Arctic and Slovakia. Biologia. 2008;63:839–847.
- Nagao M, Matsui K, Uemura M. Klebsormidium flaccidum, a charophycean green alga, exhibits cold acclimation that is closely associated with compatible solute accumulation and ultrastructural changes. Plant Cell Environ. 2008;31:872–885.
- Popper ZA, Michel G, Hervé C, et al. Evolution and diversity of plant cell walls: from Algae to Flowering plants. Annu Rev Plant Biol. 2011;62:567–590.
- Kaplan F, Lewis LA, Wastian J, et al. Plasmolysis effects and osmotic potential of two phylogenetically distinct alpine strains of Klebsormidium (Streptophyta). Protoplasma. 2012;249:789–804.
- Gerotto C, Morosinotto T. Evolution of photoprotection mechanisms upon land colonization: evidence of PSBS-dependent NPQ in late Streptophyte algae. Physiol Plant. 2013;149:583–598.
- Holzinger A, Karsten U. Desiccation stress and tolerance in green algae: consequences for ultrastructure, physiological and molecular mechanisms. Front Plant Sci. 2013;4:art.327.
- Holzinger A, Kaplan F, Blaas K, et al. Transcriptomics of desiccation tolerance in the streptophyte green alga Klebsormidium reveal a land-plant defense reaction. PLoS One. 2014;9:e110630–e110645.
- Kitzing C, Pröschold T, Karsten U. UV-induced effects on growth, photosynthetic performance and sunscreen contents in different populations of the green alga Klebsormidium fluitans (Streptophyta) from alpine soil crusts. Microb Ecol. 2014;67:327–340.
- Mikhailyuk T, Holzinger A, Massalski A, et al. Morphological and ultrastructural aspects of Interfilum and Klebsormidium (Klebsormidiales, Streptophyta) with special reference to cell division and thallus formation. Eur J Phycol. 2014;49:395–412.
- Herburger K, Holzinger A. Localization and quantification of callose in streptophyte green algae Zygnema and Klebsormidium: correlation with desiccation tolerance. Plant Cell Physiol. 2015;56:2259–2270.
- Holzinger A, Becker B. Desiccation tolerance in the streptophyte green alga Klebsormidium: the role of phytohormones. Commun Integr Biol. 2015;8:e1059978.
- Kitzing C, Karsten U. Effects of UV radiation on optimum quantum yield and sunscreen contents in members of the genera Interfilum, Klebsormidium, Hormidiella and Entransia (Klebsormidiophyceae, Streptophyta). Eur J Phycol. 2015;50:279–287.
- Munk M, Brandão HM, Nowak S, et al. Direct and indirect toxic effects of cotton-derived cellulose nanofibres on filamentous green algae. Ecotoxicol Env Safety. 2015;122:399–405.
- Ryšánek D, Hrčková K, Škaloud P. Global ubiquity and local endemism of free-living terrestrial protists: phylogeographic assessment of the streptophyte alga Klebsormidium. Mol Phylogenet Evol. 2015;58:218–231.
- Kondo S, Hori K, Sasaki-Sekimoto Y, et al. Primitive extracellular lipid components on the surface of charophytic alga Klebsormidium flaccidum and their possible pathways as deduced from genomic sequences. Front Plant Sci. 2016;7:art.952.
- Ohtaka K, Nori K, Kanno Y, et al. Primitive auxin response without TIR1 and Aux/IAA in the charophyte alga Klebsormidium nitens. Plant Physiol. 2017;174(3):1621–1632.
- De Vries J, Curtis BA, Gould SB, et al. Embryophyte stress signaling evolved in the algal progenitors of land plants. Proc Natl Acad Sci USA. 2018;115:E3471–E3480.
- Herburger K, Ryan LM, Popper ZA, et al. Localisation and substrate specificities of transglycanases in charophyte algae relate to development and morphology. J Cell Sci. 2018;131:jcs203208. [cited 19 Jan 2019][13p.].
- Ananieva K, Ananiev ED, Doncheva S, et al. Senescence progression in a single darkened cotyledon depends on the light status of the other cotyledon in Cucurbita pepo (zucchini) seedlings: potential involvement of cytokinins and cytokinin oxidase/dehydrogenase activity. Physiol Plant. 2008;134:609–623.
- Hodkinson TR, Jones MB, Waldren S, et al. editors. Climate change, ecology and systematics. Cambridge: Cambridge University Press. 2011.
- Welsh D. Ecological significance of compatible solute accumulation by micro organisms: from single cells to global climate. FEMS Microbiol Rev. 2000; 24:263–290.
- Yansey PH. Organic osmolytes as compatible, metabolic and counteracting cytoprotectants in high osmolarity and other stresses. J Experim Biol. 2005;208:2819–2830.
- Burg MB, Ferraris JD. Intracellular organic osmolytes: function and regulation. J Biol Chem. 2008;283:7309–7313.
- World Health Organization. WHO Model List of Essential Medicines (19th List). 2015 [cited 2019 Jan 19]. Available from: https://www.who.int/medicines/publications/essentialmedicines/EML2015_8-May-15.pdf
- Khan S, Ahmad N, Ahmad F, et al. Naturally occurring organic osmolytes: from cell physiology to disease prevention. IUBMB Life.. 2010;62:891–895.
- Juneja A, Ceballos RMI, Murthy GS. Effects of environmental factors and nutrient availability on the biochemical composition of algae for biofuels production: A review. Energies. 2013;6:4607–4638.
- Oren A, Gunde-Cimerman N. Mycosporines and mycosporine-like amino acids: UV protectants or multipurpose secondary metabolites?. FEMS Microbiol Lett. 2007;269:1–10.
- Wada N, Sakamoto T, Matsugo S. Mycosporine-like amino acids and their derivatives as natural antioxidants. Antioxidants. 2015;4:603–646.
- Bhatia S, Garg A, Sharma K, et al. Mycosporine and mycosporine-like amino acids: a paramount tool against ultra violet irradiation. Phcog Rev. 2011;5:138–146.
- Choi YH, Yang DJ, Kulkarni A, et al. Mycosporine-like amino acids promote wound healing through focal adhesion kinase (FAK) and mitogen-activated protein kinases (MAP Kinases) signaling pathway in keratinocytes. Mar Drugs. 2015; 3:7055–7066.
- Chrapusta E, Kaminski A, Duchnik K, et al. Mycosporine-like amino acids: potential health and beauty ingredients. Mar Drugs. 2017;15:326–356.
- Sinha RP, Singh SP, Häder DP. Database on mycosporines and mycosporine-like amino acids (MAAs) in fungi, cyanobacteria, macroalgae, phytoplankton and animals. J Photochem Photobiol. 2007;89:29–35.
- Breton G, Danyluk J, Ouellet F, et al. Biotechnological applications of plant freezing associated proteins. Biotechnol Ann Rev. 2000;6:59–101.
- Pulicherla KK, Ghosh M, Kumar PS, et al. Psychrozymes - the next generation industrial enzymes. J Marine Sci Res Dev. 2011;1:102.
- Ahanger MA, Akram NA, Ashraf M, et al. Plant responses to environmental stresses-from gene to biotechnology. AoB Plants. 2017;9:plx025.
- Sørensen I, Pettolino FA, Bacic A, et al. The charophycean green algae provide insights into the early origins of plant cell walls. Plant J. 2011;68:201–211.
- Andersen R, editor. Algal culturing techniques. Amsterdam: Elsevier Academic Press; 2005.
- Coombs J, Alston YR, editors. The International Biotechnology Directory. Basingstokes (UK): Palgrave Macmillan; 1993.
- Holzinger A, Lütz C. Algae and UV irradiation: effects on ultrastructure and related metabolic functions. Micron. 2006;37:190–207.
- Stoyneva M. Soil algae in museum samples from some Southwest Asia sites. I. Hist Nat Bulg. 2000;12:129–146.
- Painter T. Carbohydrate polymers in desert reclamation: the potential of microalgal biofertilizers. Carbohydrate Polym. 1993;20:77–86.
- Win TT, Barone JD, Secundo F, et al. Algal biofertilizers and plant growth stimulants for sustainable agriculture. Ind Biotechnol. 2018;14:203–211.
- Venkataraman GS. A method of preserving blue-green algae for seeding purposes. J Gen Appl Microbiol. 1961;7:96–98.
- Venkataraman GS. 1961. The role of blue-green algae in agriculture. Sci Cult. 1961;27:9–13.
- Venkataraman GS. Algalization. Phykos. 1966;5:164–174.
- Venkataraman GS. Algal biofertilizers and rice cultivation. Faridabad (India): Today and Tomorrow Printers and Publishers; 1972.
- Venkataraman GS. Economics and energetics of blue green algal contribution to rice. Curr. Sci. 1981;50:94–96.
- Venkataraman GS. Blue-green algae for rice production: A manual for its promotion. FAO Soils Bulletin. 1981;46:1–103.
- Innok S, Chunleuchanon S, Boonkerd N, et al. Cyanobacterial akinete induction and its application as biofertilizer for rice cultivation. J Appl Phycol. 2009;21:737–744.
- Mata TM, Martins AA, Caetano NS. Microalgae for biodiesel production and other applications: a review. Renew Sustain Energy Rev. 2010;14:217–232.
- Phang S-M, Chu W-L, Rabiei R. Phycoremediation. In: Sahoo D, Seckbach J, editors. The algae world. Dordrecht: Springer; 2015. p. 357–390.
- Davis AS, Prakash P, Thamaraiselvi K. Nanobioremediation technologies for sustainable environment. In: Prashanthi M, Sundaram R, Jeyaseelan A, editors. Bioremediation and sustainable technologies for cleaner environment, environmental science and engineering. Amsterdam: Springer International Publishing AG; 2017. p. 13–33.
- Dahoumane S, Jeffryes C, Mechouet M, et al. Biosynthesis of inorganic nanoparticles: A fresh look at the control of shape, size and composition. Bioengineering. 2017; 4:14. 4010014
- Dastjerdi R, Montazer M. A review on the application of inorganic nano-structured materials in the modification of textiles: focus on anti-microbial properties. Colloids Surf B Biointerfaces. 2010;79:5–18.
- Masaroviová E, Král’ová K. Metal nanoparticles in plants. Ecol Chem Eng S. 2013;20:9–22.
- Dahoumane AS, Yéprémian C, Djédiat C, et al. A global approach of the mechanism involved in the biosynthesis of gold colloids using micro-algae. J Nanopart Res. 2014;16:2607–2624.
- Silva PC, Mattox KR, Blackwell WH. Jr. The generic name Hormidium as applied to green algae. Taxon. 1972;21:639–645.
- Rindi F, Ryšánek D, Škaloud P. Problems of epitypification in morphologically simple green microalgae: a case study of two widespread species of Klebsormidium Klebsormidiophyceae, Streptophyta). Fottea. 2017;17:78–88.