Abstract
Myosmine, 3-(1-pyrroline-2-yl) pyridine is a minor tobacco alkaloid that has also been found in various widely used foods. Recently, this phytochemical has been gaining an increasing interest as a potential risk factor for the development of oesophageal adenocarcinoma. This study aimed to examine the effects of myosmine on the cell viability and proliferative activity of erythroleukemia and hepatocellular carcinoma cells and to obtain additional information about the mechanisms underlying its cytotoxic activity. The in vitro cytotoxic effect of myosmine on the HepG2 and MEL tumour cell lines was assessed by MTT dye reduction and trypan blue dye exclusion assays. The alterations in the tumour cell morphology induced by myosmine were analysed by fluorescent microscopy after staining with acridine orange (AO)/ethidium bromide (EtBr) and 4′,6-diamidine-2′-phenylindole dihydrochloride (DAPI). Annexin V-FITC/propidium iodide (PI) staining was used to assess the apoptosis-inducing ability of myosmine. The modulating action of antioxidant treatment on myosmine-induced cytotoxicity against the HepG2 tumour cell line was also examined. The cell viability tests indicated that myosmine induced a significant dose-dependent reduction of the viability and proliferative activity of both tumour cell lines. Fluorescent microscopy studies revealed marked alterations in the morphology of myosmine-treated tumour cells with signs of cell cycle arrest and apoptosis. The results of the simultaneous treatment with myosmine and vitamin C showed modulating activity of vitamin C on the cytotoxic effect of myosmine with concentration- and time-dependent variations. The presented results could contribute to the assessment of the potential health risks associated with the dietary myosmine exposure. Abbreviations AO: acridine orange; DAPI: 4‘,6-diamidine-2‘-phenylindole dihydrochloride; DMEM: Dulbecco's modified Eagle medium; DMSO: dimethyl sulfoxide; EtBr: ethidium bromide; FITC: fluorescein isothiocyanate; GERD: gastroesophageal reflux disease; MEL: murine erythroleukemia; MTT: 3-(4,5-dimethylthiazol-2-yl)-2,5-diphenyltetrazolium bromide; NNN: N'-nitrosonornicotine; PBS: phosphate buffered saline; PI: propidium iodide
Introduction
Myosmine, 3-(1-pyrrolin-2-yl)pyridine, is an alkaloid found in tobacco [Citation1]. Unlike nicotine, the occurrence of myosmine is not limited to plants of the family Solanaceae. After the detection of myosmine in Duboisia hopwoodii, it was also found in nuts and nut products [Citation2]. In subsequent studies, myosmine has been identified in various staple foods like wheat, maize, rice and milk as well as in different fruits and vegetables at concentrations between 0.02 and 6.1 ng/g [Citation3]. It is not surprising, therefore, that myosmine has also been detected in human toenail, plasma and saliva [Citation4]. Recent data indicate that myosmine may be a tobacco-independent carcinogenic source associated with the increased incidence of oesophageal adenocarcinoma [Citation5, Citation6]. It has been shown that the reactions of nitrosation and peroxidation of myosmine take part in its metabolic activation and lead to formation of the oesophageal carcinogen N′-nitrosonornicotine (NNN) and other reactive intermediates capable of forming DNA and protein adducts [Citation6–8]. Thus, endogenous nitrosation of myosmine present in the diet could be a significant risk factor for the occurrence of tumours in the upper digestive tract, such as oesophageal adenocarcinoma, which are not associated with tobacco smoking [Citation6]. Due to its imine structure, myosmine exhibits higher reactivity than that of nicotine and other tobacco alkaloids [Citation8]. It can be assumed that superoxide or hydroxyl radicals are the reactive species involved in myosmine peroxidation. Increased carcinogenic potential of myosmine may be expected when the oxidative stress in the cell is enhanced. Literature data support the hypothesis that myosmine peroxidation might be facilitated by enhanced oxidative stress in the cell thus increasing the effect of its genotoxic activity [Citation8]. The genotoxicity of myosmine has been studied in a number of in vitro models. Comet assay studies have shown that myosmine induces DNA damage in nasal mucosa cells, human lymphocytes and in cell lines derived from human oesophageal adenocarcinoma [Citation9, Citation10].
Gastroesophageal reflux disease (GERD) is a digestive disorder known as a major risk factor for development of oesophageal adenocarcinoma [Citation11]. The chronic inflammation caused by GERD is related to an increase in oxidative stress and acidity in the oesophagus thus creating conditions for endogenous peroxidation and nitrosation of myosmine and formation of reactive intermediates with carcinogenic potential [Citation10]. It has been found that the high dietary intake of the antioxidants vitamin C, beta-carotene and alpha-tocopherol significantly decrease the risk of oesophageal adenocarcinoma, and this effect has been greater in patients with GERD [Citation12]. Vitamin C has been reported to reduce considerably endogenous nitrosations in humans [Citation13].
This study aims to examine the antiproliferative and proapoptotic effect of myosmine on murine erythroleukemia (MEL) and human hepatocellular carcinoma HepG2 cell lines. Previously, we have studied the modulating activity of vitamin C on the inhibitory action of myosmine on the cell growth and on the cell cycle of MEL cells [Citation14]. For the purpose of studying the interrelation between the oxidative status of the cells and the cytotoxic activity of myosmine, we explored the combined effect of the antioxidant vitamin C applied along with myosmine on HepG2 cells. The HepG2 cell line has been widely used as a model system for studies of liver metabolism and toxicity of xenobiotics as well as for the identification of environmental and dietary cytotoxic and genotoxic agents [Citation15]. Vitamin C was selected as an antioxidant because of its wide use as a dietary supplement and its presence in the daily diet. To clarify the mode of cell death induced by myosmine, the morphological alterations in MEL and HepG2 cells were examined by fluorescent microscopy.
Materials and methods
Myosmine
3-(1-Pyrrolin-2-yl)pyridine, CAS Number 532-12-7 (Sigma-Aldrich, St. Louis, MO), was used in the experiments. Myosmine solutions were prepared in cell culture media immediately prior to the cell treatment.
Cell cultures
MEL, clone F4N –MEL cells (virus-transformed erythroid precursor cells), were obtained from the European Collection of Authenticated Cell Cultures (ECACC). Cells were cultured in Minimum Essential Media (Gibco, Carlsbad, CA) containing 10% calf serum, 100 U mL−1 penicillin and 100 µg mL−1 streptomycin and were cultivated in an incubator at 37 °C in a humidified atmosphere with 5% CO2.
HepG2 cells – human hepatocellular carcinoma were obtained from American Type Culture Collection (ATCC) and were grown in Dulbecco’s modified Eagle medium (Gibco, Carlsbad, CA) supplemented with 10% foetal calf serum, 2 mmol L−1 glutamine and antibiotics penicillin 100 U mL−1 and streptomycin100 µg mL−1 at 37 °C and 5% CO2.
Cell viability assays
Trypan blue dye exclusion test
The effect of myosmine on the cell viability and proliferative activity of MEL cells was studied by trypan blue dye exclusion test. An aliquot of 1 × 105 MEL cells mL−1 in the exponential phase of growth was incubated with five different concentrations of myosmine (1.25, 2.5, 5, 10 and 20 mmol L−1) in 24-well microtiter plates (Orange Scientific, Braine-l’Alleud, Belgium). After 24 h of treatment with myosmine, the cells were stained with trypan blue and counted with an automatic cell counter (CountessTM, InvitrogenTM, ThermoFisher Scientific).
MTT dye reduction test
The cytotoxic and antiproliferative potential of myosmine was evaluated in HepG2 cells by MTT-test according to the standard procedure [16]. Briefly, cultures of HEP-G2 cells in the exponential phase of growth were trypsinized and seeded in 96-well microtiter plates (100 μL well−1) at a density of 1 × 105 cells mL−1. The cells were allowed to adhere for 24 h and then were treated with eight concentrations of myosmine ranging from 0.2 to 20 mmol L−1 (six replicates/concentration). The modulating effect of the vitamin C (200 µmol L−1) on the cytotoxicity of myosmine was examined in parallel experiments. The control and treated cells were incubated for 24 and 48 h in an incubator at 37 °C and 5% CO2. The treatment medium was replaced with DMEM containing 0.5 mg mL−1 MTT and the cells were incubated for three hours. The MTT solution was removed from the plates and the formed formazan crystals were dissolved in DMSO: 96% ethanol (1:1 v/v) solution. The optical density of the samples was measured by a microplate reader (TECAN, Sunrise TM, Groedig/Salzburg, Austria) at 540 nm.
Fluorescent microscopy
The morphological alterations induced by myosmine in MEL and HepG2 cell were analysed by fluorescent microscopy. HepG2 cells were cultured on 13-mm-diameter cover glasses in 24-well plates and were treated for 24 h with myosmine in a concentration equal to the IC50 value established by the MTT test. MEL cells were treated with myosmine as described above for the cell viability test. After 24 h, the cell suspension was centrifuged for 5 min at 125×g, washed with PBS and dropped on microscope slides. MEL and HepG2 cells were stained by three different methods:
Acridine orange/ethidium bromide-double staining. Acridine orange (AO) and ethidium bromide (EtBr) (live/dead) staining were performed as previously described [Citation17]. Briefly, cell preparations of HepG2 and MEL cells were stained with the fluorescent dyes AO (5 µg mL−1) and EtBr (5 μg mL−1) in PBS.
DAPI staining. The alterations in the nuclear morphology of the HepG2 and MEL cells induced by myosmine were studied after staining with the DNA-binding dye 4′,6-diamidine-2′-phenylindole dihydrochloride (DAPI). The cells were fixed with methanol, incubated for 15 min in 1 µg mL−1 DAPI in methanol in the dark.
Annexin V and propidium iodide (PI) double staining. The apoptosis-inducing ability of myosmine was assessed using Annexin V Apoptosis Detection Kit: sc-4252 AK (Santa Cruz Biotechnology, Inc., Dallas, TX) according to the manufacturer’s instructions. The cells were fixed with 3% paraformaldehyde, washed with assay buffer and incubated in a solution containing 5 µg mL−1 Annexin V-FITC and 5 µg mL−1 PI for 10 min, in the dark, at room temperature.
Stained cell cultures were visualized and examined under a fluorescence microscope (Leica DM 5000B, Wetzlar, Germany).
Statistical analysis
Statistical analysis was performed by One-way analysis of variance (ANOVA) followed by Bonferroni’s post-hoc test (GraphPad Prism software package, GraphPad, La Jolla, CA). Values of p < .05 were accepted as the lowest level of statistical significance. Nonlinear regression (curve fit) analysis (GraphPad Prism) was applied to determine the concentrations inducing 50% inhibition of the cell growth (IC50 values).
Results and discussion
Antiproliferative and cytotoxic effect of myosmine on MEL cells
The growth inhibitory and cytotoxic effect of the treatment of MEL cells with five different concentrations of myosmine, ranging from 1.25 to 20 mmol L−1, for 24 h was examined by trypan blue dye exclusion test (). The presented results indicate that myosmine induced statistically significant reduction of the cell viability compared to the control at concentrations of 5, 10 and 20 mmol L−1. The observed effect showed a clear concentration dependence. The IC50 value of myosmine was 6.940 mmol L−1.
Antiproliferative and cytotoxic effect of myosmine applied alone or in combination with vitamin C on HepG2 cells
The effect of the myosmine treatment on the viability of HepG2 cells and the modulating action of 200 µmol L−1 vitamin C was studied by MTT test after 24 and 48 h of exposure (). The results indicated that myosmine reduced the cell viability and proliferative activity in a concentration- and time-dependent manner. The cell viability of the myosmine-treated cells was significantly lower compared to the controls at concentrations ranging from 2.5 to 20 mmol L−1 after 24 h of exposure (). After 48 h of exposure, significant reduction of the viability was also established in the cell cultures treated with 1.25 mmol L−1 myosmine (). Statistically significant dose-dependence of the cytotoxic effect of myosmine was found at the concentration range 2.5–20 mmol L−1 and 0.31–5 mmol L−1 for 24 or 48 h, respectively. The extension of the exposure time from 24 to 48 h resulted in a statistically significant increase of the cytotoxic effect of myosmine at the concentration range 2.5–10 mmol L−1. Myosmine-treatment with 0.16 mmol L−1 for 48 h induced a slight increase in the cell viability compared to the control. The simultaneous treatment with myosmine and vitamin C for 24 h resulted in a reduction of the cell viability compared to the treatment with myosmine only; however, the observed difference was not statistically significant (). At 48 h, there was protective effect of vitamin C against the myosmine-induced cytotoxicity at the concentration range 0.63–5 mmol L−1 (). This effect was statistically significant at 2.5 and 5 mmol L−1 myosmine. As a result of the addition of vitamin C, the IC50 value of myosmine was reduced from 5.219 to 4.478 mmol L−1 at 24 h and increased from 1.958 to 2.483 mmol L−1 at 48 h.
Cytomorphological alterations induced by myosmine in MEL and HepG2 cells
The morphological alterations induced by myosmine in MEL and HepG2 cells were examined by fluorescent microscopy of control and treated cells stained with AO/ EtBr, DAPI and annexin V-FITC/PI.
Acridine orange/ethidium bromide staining of control and myosmine-treated MEL cells
Control MEL cells stained with AO/ EtBr were round-shaped with homogenous green staining (). MEL cells treated with 2.5 mmol L−1 myosmine showed early apoptotic changes, such as membrane blebbing and chromatin condensation evident as bright yellow–green areas in the nucleus, karyopyknosis and nuclear fragmentation (). In the cell cultures exposed to myosmine in concentrations higher than 5 mmol L−1, there were late apoptotic cells with orange fluorescence, indicating the loss of membrane integrity and passage of EtBr into the cell (). There was a concentration-dependent increase in the number of the late apoptotic cells.
Figure 3. Fluorescent microscopy images of the morphological alterations induced by myosmine in murine erythroid leukaemia cell line MEL, clone F4N.Control cell cultures (a, d, h); cell cultures exposed to 2.5 mmol L−1 (b, e, i) or 10 mmol L−1 (c, f, j) myosmine for 24 h. Acridine orange/ethidium bromide staining (a, b); DAPI staining (c, d); Annexin V-FITC and propidium iodide staining (e, f).
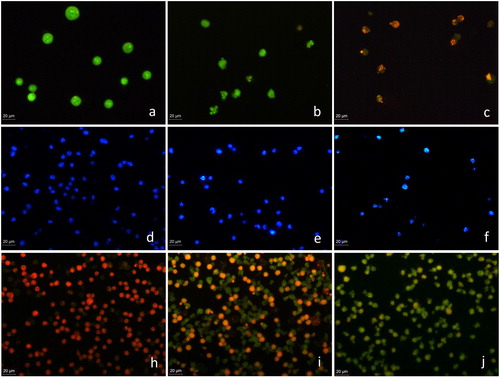
DAPI staining of control and myosmine-treated MEL cells. The nuclei of the untreated MEL cells were round to oval in shape with diffuse blue staining (), whereas the myosmine-treated cells were with irregular shape and showed chromatin condensation (bright blue staining) and nuclear fragmentation (). The observed apoptotic nuclear abnormalities aggravated with the increase of myosmine concentration ().
Annexin V-FITC/propidium iodide staining of control and myosmine-treated MEL cells. The apoptogenic potential of myosmine was studied by fluorescent microscopy after Annexin V-FITC/PI staining of the cells. PI was used to visualize all cells after fixation and FITC labelled Annexin V, to mark the apoptotic cells. The control MEL cells were stained only with PI and appeared red (), whereas the myosmine-treated cells had green fluorescence due to binding of Annexin V to phosphatidylserine exposed on the surface of the apoptotic MEL cells ().
Acridine orange/ethidium bromide staining of control and myosmine-treated HepG2 cells. Microscopic observation showed that the control viable HepG2 cells showed green fluorescence due to the diffusion of AO through the cellular membrane (). Myosmine treatment induced significant alterations in the shape of the cells and nuclei. Most of the cells were early apoptotic with intense yellow–green fluorescence, chromatin condensation and nuclear fragmentation. Late apoptotic and necrotic cells showed orange and red fluorescence due to the loss of membrane integrity and the passage of EtBr into the cells ().
Figure 4. Fluorescent microscopy images of the morphological alterations induced by myosmine in human hepatocellular carcinoma cell line HepG2. Control cell cultures (a, c, d); cell cultures exposed to 5 mmol L−1 myosmine for 24 h (b, d, f). Acridine orange/ethidium bromide staining (a, b); DAPI staining (c, d); Annexin V-FITC and propidium iodide staining (e, f).
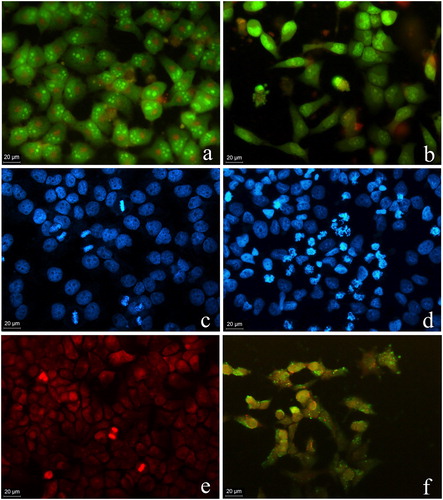
DAPI staining of control and myosmine-treated HepG2 cells. The nuclei of the control cells showed normal morphology and homogenous blue staining (). Myosmine-treated cells were smaller with more brightly stained nuclei. Chromatin condensation that intensifies the DAPI staining as well as nuclear fragmentation and formation of apoptotic bodies were observed ().
Annexin V-FITC/propidium iodide staining of control and myosmine-treated HepG2 cells. The control HepG2 cells showed homogenous red fluorescence as a result of PI staining because both control and treated cells were fixed with 3% paraformaldehyde. Green fluorescence, which indicates apoptotic changes in the cellular membrane, was not found in the control cells (). Myosmine-treated HepG2 cells showed apoptotic membrane changes visible as green fluorescence staining of the cells ().
Myosmine is an alkaloid chemically closely related to nicotine. Myosmine has been found in tobacco as well as in various widely used foods such as corn, potatoes, rice, kiwi, apples, tomatoes, cocoa, milk and cream [Citation3, Citation18]. Recently, myosmine has received considerable attention as a potential carcinogenic risk factor associated with the development of oesophageal adenocarcinoma [Citation6]. The presence of myosmine in the daily diet determines the need of more detailed studies of its toxicity, genotoxicity and carcinogenicity that will enable the assessment of the potential human health risks.
Previously published data have shown that high concentrations of myosmine (10–50 mmol L−1) induce a weak but significant concentration and time-dependent genotoxic effect in human lymphocytes and upper aerodigestive tract epithelial cells [Citation9] as well as in the human oesophageal adenocarcinoma cell line [Citation10]. In this study, the cytotoxic potential of myosmine was assessed by MTT and trypan blue tests in HepG2 and MEL cell cultures. Significant reduction of the cell viability and proliferation with clearly expressed concentration-dependency was found in both cell lines.
Our previous studies have shown that myosmine affects the cell cycle progression of murine bone marrow cells in vivo and MEL cells in vitro. Significant increase of the percentage of cells in S and G2/M phases was found in both cell types. The flow cytometry analysis also indicated that myosmine induced a fraction of hypoployd cells [Citation19]. The influence of the oxidative status of the cells on the inhibitory effect that myosmine has on the cell proliferation was studied in MEL cells. A protective effect of the antioxidant vitamin C against myosmine-induced cytotoxicity has been found [Citation13]. This study examined the effect of this antioxidant on the antiproliferative and cytotoxic potential of myosmine in HepG2 cells. The results indicated that vitamin C significantly reduced the cytotoxic effect of myosmine applied in concentrations 2.5 and 5 mmol L−1 after 48 h of treatment.
To analyse the nature of cell death caused by myosmine, morphological studies of untreated and myosmine-treated MEL and HepG2 cells were performed. The induced alterations in the cellular and nuclear morphology indicated that myosmine showed apoptosis-inducing ability. These results were confirmed by cytochemical investigation with Annexin V- FITC staining, which showed apoptotic membrane alterations in the cells exposed to myosmine.
In addition to the known genotoxic activity, myosmine has also been found to inhibit DNA-repair [Citation10, Citation20]. The results of the morphological studies indicate that myosmine induced apoptosis, which could probably be due to the inability of the cells to repair the DNA damage.
Conclusions
Myosmine induced a significant and dose- and time-dependent reduction of the cell viability of erythroleukemia and hepatocellular carcinoma cells. The antioxidant vitamin C exerted a moderate modulating effect on the cytotoxic action of myosmine in HepG2 cells. Fluorescent microscopy of myosmine-treated cells showed morphological alterations typical of apoptosis, such as reduction of the cellular and nuclear volume, condensation of chromatin, fragmentation of the nucleus, membrane blabbing and translocation of phosphatidylserine to the outer membrane surface. These results suggest that apoptotic cell death could be one of the main mechanisms of the antiproliferative and cytotoxic action of myosmine.
Disclosure statement
No potential conflict of interest was reported by the authors.
Additional information
Funding
References
- Zwickenpflug W, Tyroller S, Richter E. Metabolism of myosmine in Wistar rats. Drug Metab Dispos. 2005;33:1648–1656.
- Zwickenpflug W, Meger M, Richter E. Occurrence of the tobacco alkaloid myosmine in nuts and nut products of Arachis hypogaea and Corylus avellana. J Agric Food Chem. 1998;46:2703–2706.
- Tyroller S, Zwickenpflug W, Richter E. New sources of dietary myosmine uptake from cereals, fruits, vegetables, and milk. J Agric Food Chem. 2002;50:4909–4915.
- Schütte-Borkovec K, Heppel K, Heling CW, et al. Analysis of myosmine, cotinine and nicotine in human toenail, plasma and saliva. Biomarkers. 2009;14:278–284.
- Bollschweiler E, Wolfgarten E, Gutschow C, et al. Demographic variations in the rising incidence of esophageal adenocarcinoma in white males. Cancer Am Cancer Soc. 2001;92:549–555.
- Wilp J, Zwickenpflug W, Richter E. Nitrosation of dietary myosmine as risk factor of human cancer. Food Chem Toxicol. 2002;40:1223–1228.
- Zwickenpflug W. N-nitrosation of myosmine yields HPB (4-hydroxy-1-(3-pyridyl)-1-butanone) and NNN (N-nitrosonornicotine). J Agric Food Chem. 2000; 48:392–394.
- Zwickenpflug W, Tyroller S. Reaction of the tobacco alkaloid myosmine with hydrogen peroxide. Chem Res Toxicol. 2006;19:150–155.
- Kleinsasser NH, Wallner BC, Harréus UA, et al. Genotoxic effects of myosmine in human lymphocytes and upper aerodigestive tract epithelial cells. Toxicology. 2003;192:171–177.
- Vogt S, Fuchs K, Richter E. Genotoxic effects of myosmine in a human esophageal adenocarcinoma cell line. Toxicology. 2006;222:71–79.
- Shaheen N, Ransohoff DF. Gastroesophageal reflux, Barrett esophagus, and esophageal cancer: scientific review. JAMA. 2002;287:1972–1981.
- Terry P, Lagergren J, Ye W, et al. Antioxidants and cancers of the esophagus and gastric cardia. Int J Cancer. 2000;87:750–754.
- Vermeer ITM, Moonen EJC, Dallinga JW, et al. Effect of ascorbic acid and green tea on endogenous formation of N-nitrosodimethylamine and N-nitrosopiperidine in humans. Mutat Res. 1999;428:353–361.
- Mateva R, Detcheva R, Pajpanova T, et al. Modulating action of vitamin C on the cytotoxic effect of myosmine. C R Acad Bulg Sci. 2017;70:237–242.
- Shah UK, Mallia J, Singh N, et al. A three-dimensional in vitro HepG2 cells liver spheroid model for genotoxicity studies. Mut Res/Gen Toxicol Environ Mut. 2018;825:51–58.
- Mossman T. Rapid colorimetric assay for cellular growth and survival: application to proliferation and cytotoxicity assays. J Immunol Meths. 1983;65:55–63.
- Toshkova R, Manolova N, Gardeva E, et al. Antitumor activity of quaternized chitosan-based electrospun implants against Graffi myeloid tumor. Int J Pharm. 2010;400:221–233.
- Leslie LJ, Vasanthi Bathrinarayanan P, Jackson P, et al. A comparative study of electronic cigarette vapor extracts on airway-related cell lines in vitro. Inhalat Toxicol. 2017;29:126–136.
- Boteva BI, Mateva RM, Iliev IA, et al. Flow cytometric analysis of the influence of myosmine on the cell cycle. Croat Chem Acta. 2011;84:355–359.
- Dator R, von Weymarn LB, Villalta PW, et al. In vivo stable-isotope labeling and mass-spectrometry-based metabolic profiling of a potent tobacco-specific carcinogen in rats. Anal Chem. 2018;90:11863–11872.