Abstract
p53 is usually regarded as a tumour suppressor protein but its constant activation may result in pro-tumorigenic inflammation. The activation of p53 can be provoked by an increase in its concentration as a result of high level transcription, by transformation of the p53 protein to an active conformation or by its translocation from the cytoplasm to the nucleus. p53 can be activated by a wide variety of stress signals that a cell might encounter during malignant progression, such as genotoxic damage, oncogene activation and hypoxia. We found that the HMGB1 protein can play the role of a signal molecule that provokes the accumulation of p53 in the nucleus. Only the full-length protein stimulated the translocation of p53 from the cytosol to the nucleus and the effect was considerably strong and almost equal to that generated by the positive control actinomycin D. The truncated tail less form of HMGB1 was not functional. This supported the hypothesis that the C terminus plays an important role in regulating the properties of HMGB1.
Keywords:
Introduction
Tumour development and response to therapy both depend on the balance between apoptosis (‘programmed cell death’) and autophagy (‘programmed cell survival’). The High mobility group box 1 protein HMGB1 and the tumour suppressor protein p53 are essential partners in cellular fate determination in terms of apoptosis and autophagy regulation. It is shown that they form a complex regulating the balance between tumour cell death and survival [Citation1]. Any change in the delicate p53–HMGB1 relationship would lead to misbalance in cell death pathways and might result in increased levels of autophagy and cell survival, respectively, to drug resistance [Citation2]. The tumour suppressor factor p53 is involved in various cellular processes. Normally p53 exists as an inactive form in the cytoplasm, and several cellular stimuli, such as genotoxic DNA damage, hypoxia, deprivation of growth factors etc., can induce the formation of active p53. A series of studies by Liang and Clarke [Citation3] have shown that the activation of p53 can be registered at three levels: (a) an increase of the p53 protein concentration as a result of increased translation or elongated half‐life; (b) the transformation of the p53 protein to an active conformation; and (c) the translocation of the p53 protein from the cytoplasm to the nucleus [Citation3]. In most unstressed cells p53 undergoes nuclear-cytoplasmic shuttling. In response to DNA damage and other stress factors, the protein is subjected to various post-translational modifications that result in its stabilization, accumulation in the nucleus and activation as a transcription factor [Citation4]. The nuclear import is essential for p53 for its normal functions as a transcription factor. There is piling evidence that several proteins such as hsp70 family member Mot2, Bcl2, calcium‐dependent protein kinase C MDM2, etc. [Citation3–6] are involved in p53 regulation.
High-mobility group box 1 (HMGB1) is a nuclear nonhistone protein composed of three domains, two basic HMG-box domains (HMG boxes A and B) and a highly acidic C-terminal domain. Through its ability to bend DNA, HMGB1 plays an ‘architectural’ role in many DNA-dependent nuclear processes, such as transcription, replication, recombination and repair [Citation7–9]. HMGB1 facilitates DNA binding of a number of transcription factors including the tumour suppressor p53 [Citation3]. HMGB1 might serve also as a sensor of DNA damage that provokes p53-mediated DNA damage response [Citation10, Citation11] and may act at different steps—from damage recognition to adduct removal, possibly affecting each step differently. The new role of HMGB1 outside the cell is now a subject of intensive studies. When released into the extracellular medium, this protein serves as a tissue damage signal and acts as a damage-associated molecular pattern (DAMP) [Citation12]. Extracellular HMGB1 is also responsible for the inflammatory response, tumourigenesis and increased metastatic potential [Citation7, Citation13]. Growing evidence clearly demonstrates the relationship between the functions of HMGB1and p53 in some cellular processes. Keeping in mind that the tumour suppressor p53 is activated by a wide variety of stress signals that a cell might encounter during malignant progression, genotoxic damage, oncogene activation, hypoxia etc., we decided to address the effect of HMGB1 on p53’s transition from the cytosol to the nucleus in lung cancer cells A549. Two protein constructs were used: full-length HMGB1 and a truncated form lacking the C terminus (HMGB1ΔC). We were motivated to apply the truncated form in our research, as the C terminus serves as a modulator of HMGB1 properties in many cellular processes like replication, repair and remodelling [Citation8, Citation9, Citation14, Citation15].
Material and methods
Cell culture
Human adenocarcinoma lung cancer cell line A549 (ATCC) was used in this study. The cells were cultured in Dulbecco’s modified Eagle’s medium (DMEM, Thermo Fisher) supplemented with 10% foetal bovine serum (Thermo Fisher), 5% L-glutamine (PAA) and 1% penicillin/streptomycin (PAA). The cells were maintained by sub-culturing in 25 cm2 tissue culture flasks and were incubated at 37 °C in a CO2 incubator in an atmosphere of humidified 5% CO2 and 95% air. Cells growing in the exponential phase were used for all the experiments.
Preparation of plasmid constructs and purification of recombinant HMGB1 proteins
Recombinant full-length, and truncated (ΔC) forms of HMGB1 were cloned in pET28a+ (Novagen) plasmid vector [Citation16] and expressed in modified E. coli BL21 Poly Lys S. The His-tagged HMGB1 and HMGB1ΔC proteins were purified on a HIS Select HF Nickel Affinity gel (Sigma) as previously described [Citation17]. After affinity purification, the His-tags were removed by using 0.5 units of thrombin (human plasma, Calbiochem) per milligram recombinant protein. The purity of the proteins was assessed by SDS-18% polyacrylamide gel electrophoresis (PAGE).
Immunofluorescence
Cells were grown on glass coverslips, fixed in 3.7% paraformaldehyde in phosphate-buffered saline (PBS) for 5 min at room temperature and permeabilized with 0.1% Triton X-100 in PBS for 2 × 5 min. After blocking in 10% foetal calf serum, 1% bovine serum albumine and 0.1% TX-100 in PBS, the coverslips were incubated in anti-p53 mouse monoclonal antibody clone DO7 (Biolegend) at 1:500 dilutions at 37 °C for 30 min, visualized with secondary donkey anti-mouse Alexa488 antibody and mounted with ProLong Gold containing DAPI mounting medium (Thermo Fisher). Images were obtained with a Zeiss AxioVert 200 M microscope using a 63× objective lens (NA = 1.4) equipped with a CCD camera AxioCam MRm. All images in an experiment were taken under identical settings. The images were processed using Image J. The intensity levels were adjusted equally on all samples within the experiment.
Western blot analysis
Total protein lysates were obtained by lysing the cells with RIPA buffer supplemented with proteinase inhibitor cocktail (Roche) and quantified by Bradford assay (Bio-Rad). Cytoplasm and nuclear fractions were isolated using a Nuclear/Cytosol Fractionation Kit (BioVision) following the manufacturer's instructions. The total protein concentration in each fraction was quantified by Bradford assay (Bio-Rad). One hundred micrograms of each sample were denatured in sample buffer (50 mmol/L Tris HCl pH 6.8; 2% SDS; 10% glycerol; 1% β-mercaptoethanol; 12.5 mmol/L EDTA, 0.02% bromphenol blue), subjected to 12% SDS-PAGE, transferred to nitrocellulose membranes and incubated overnight at 4 °C with the appropriate antibodies: anti-β-actin (Thermo Fisher; 1:2000), anti-p53 (Abcam; 1:2000), anti-PCNA (Biolegend 1:500). Proteins were visualized using a Li-cor Odyssey IR imaging system with appropriate IRDye-labeled secondary antibodies (Li-cor Biosciences).
Statistical analysis
For statistical analysis, GraphPad Prism 7 software was used. The western blot data are presented as mean values with standard deviation (± SD) of three independent experiments. Student’s t-test was used to compare the means between groups. Statistical differences in microscopic analysis were assessed by one-way analysis of variance (ANOVA). P-values ≤ 0.05 were considered to indicate statistically significant results.
Results and discussion
Normally the induction of apoptosis or senescence is the key mechanism by which p53 eliminates cancer cells. p53 can also prevent cancer development through a number of other mechanisms. For example, p53 has been shown to promote autophagy through negative regulation of mTOR signalling and to act as an antioxidant to prevent DNA damage and genome instability [Citation18, Citation19]. p53 is usually regarded as a tumour suppressor, but its constant activation may result in pro-tumorigenic inflammation via HMGB1 release [Citation20]. It was shown that decreased levels of HMGB1 increased p53 cytoplasmic translocation. The same pattern was reported when low expression level of p53 in colon cancer cells resulted in HMGB1 enhanced cytoplasmic localization [Citation1, Citation2, Citation21]. Such a correlation suggested that the HMGB1/p53 interdependence affected their cellular redistribution. The localization of both proteins turned out to be closely related to autophagy, which suggested that the relationship between HMGB1 and p53 may regulate the balance between tumour cell death and survival. A possible mediator may be RAGE (Receptor for Advanced Glycation End products), which is a specific cellular receptor for HMGB1 [Citation22, Citation23].
In order to test whether the nonhistone protein HMGB1, when secreted in the extracellular medium, may activate the tumour suppressor factor p53 and stimulate its transition from the cytoplasm to the nucleus, we incubated human adenocarcinoma cells A549 with full-length HMGB1 and its truncated form. We expressed and purified recombinant HMGB1 and its truncated form lacking the C terminus (HMGB1ΔC). The non-small cell lung cancer (NSCLC) line A549 with a functional p53 gene was used as an experimental model. The cells were treated with increased protein concentrations of purified recombinant HMGB1 and HMGB1ΔC. The optimal results were obtained at 10 nmol/L final concentration of both proteins and an incubation time of 16 h. The localisation of p53 was registered with anti-p53 antibody (). As a positive control we used actinomycin D (ActD) at a final concentration of 10 nmol/L (). ActD is an antineoplastic antibiotic isolated from Streptomyces parvullus, reported to induce cytotoxicity and apoptosis. The application of high doses of ActD (> 800 nmol/L) is limited because of its high toxicity due to its action as a transcription blocker. However, at low doses (10–100 nmol/L), it induces p53 expression without being highly toxic [Citation18]. The serum used for cell cultivation contains numerous kinds of growth factors and cytokines which contribute to the activation of the signal pathways related to survival and proliferation of the cells of interest. To avoid such effects and to bring the cells in the same phase of the cell cycle, the cells were kept for 8 h in 1% FBS prior to all incubations ().
Figure 1. Immunofluorescent analysis of p53 localization in control and HMGB1-treated human adenocarcinoma lung cancer cells A549. (A–E) P53 signal alone is presented in the black-and-white images on the left parts of panels (A–E). The right parts of the panels present two-colour merged images [where p53 signal (green) is superposed with DAPI staining (red)]. (F) Statistical analysis of the fluorescence intensity of the nuclear p53; an outline of the nuclei counterstained with DAPI was used in the green channel and the integrated density in these masks was calculated. Minimum 150 nuclei were scored for each experimental condition in three independent experiments (n = 3). (G) Differences among the groups were determined by ANOVA with Tukey's multiple comparisons test using GraphPad Prism version 7.0. A value of P < 0.05 was considered statistically significant (**P < 0.01; ***P < 0.001; ****P < 0.0001).
![Figure 1. Immunofluorescent analysis of p53 localization in control and HMGB1-treated human adenocarcinoma lung cancer cells A549. (A–E) P53 signal alone is presented in the black-and-white images on the left parts of panels (A–E). The right parts of the panels present two-colour merged images [where p53 signal (green) is superposed with DAPI staining (red)]. (F) Statistical analysis of the fluorescence intensity of the nuclear p53; an outline of the nuclei counterstained with DAPI was used in the green channel and the integrated density in these masks was calculated. Minimum 150 nuclei were scored for each experimental condition in three independent experiments (n = 3). (G) Differences among the groups were determined by ANOVA with Tukey's multiple comparisons test using GraphPad Prism version 7.0. A value of P < 0.05 was considered statistically significant (**P < 0.01; ***P < 0.001; ****P < 0.0001).](/cms/asset/0c675257-d9ca-4a20-accc-07b1a374112e/tbeq_a_1604159_f0001_c.jpg)
The immunofluorescent images of A549 cells incubated with HMGB1 and HMGB1ΔC (, respectively) clearly show that only the full- length HMGB1 protein induced p53 translocation from the cytoplasm to the nucleus. In this experiment, we used non-treated and starved A549 cells (, respectively) as negative controls, and cells treated with ActD as a positive control ().
The removal of the acidic C-tail abolished the property of HMGB1 to induce p53 translocation from the cytoplasm to the nucleus. The quantification of the fluorescence intensity for p53 in the outline of the nuclei, counterstained with DAPI (shown in red in the merged images) of at least 150 scored nuclei per each experimental condition, is shown in . It can be seen that HMGB1 had a well-defined stimulatory effect on the p53 protein transition from the cytoplasm to the nucleus and that the C-terminus was indispensable in this process. HMGB1-treated cells demonstrated an almost twofold increase in the fluorescence intensity for the p53 signal in the nuclei than the untreated or starved cells. The calculated ANOVA ) clearly demonstrates that only full-length HMGB1 had a statistically significant stimulatory effect.
To further investigate the precise intracellular translocation of p53 in HMGB1-treated A549 cells, we performed cell fractionation followed by western blot analysis. The results are presented in . β actin was used as a loading control for the cytoplasmic signal, and Proliferating Cell Nuclear Antigen (PCNA) for the nuclear. The quantification of the signals is presented as a mean value ± SD of three independent experiments (). Indeed, the cytoplasmic fraction of p53 slightly decreased; however, the increase of the nuclear fraction was nearly threefold. This result indicates that the observed accumulation of p53 in the nuclei of HMGB1-treated cells might be a consequence of a more complex synergistic effect of translocation, stabilization and an increase in the transcription level of the protein. Future experiments should further explore the role of full-length HMGB1 in p53 regulation.
Figure 2. Western blot analysis of p53 level in the cytoplasm, in the nucleus or in a total cell lysate of A549 cells in response to 10 nmol/L HMGB1 for 16 h. (A) In these experiments both the control and the HMGB1 cells incubated with 10 nmol/L were starved for 8 h in low serum (1% FBS) medium prior to the incubation. β-actin was used as a loading control for the cytoplasmic fraction and PCNA for nuclear fraction. (B) Densitometric analysis is summarised in the bar graph as mean values ± SD (n = 3). *P < 0.05 (Student’s t-test). (C) Western blot analysis of total lysates of control and 10 nmol/L HMGB1-treated cells. ****P < 0.0001 (Student’s t-test).
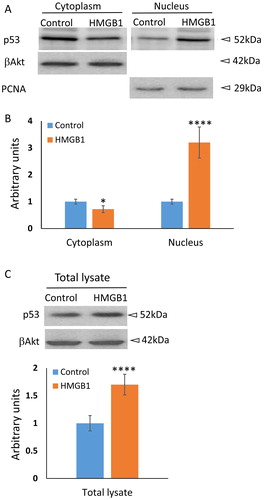
The C-terminus of the protein was found to be an important regulator of fundamental biological functions of HMGB1 either by participating in protein–protein interactions or by affecting the conformation of the full-length HMGB1 [Citation21]. Our results clearly show that only the full-length protein stimulated the translocation of p53 from the cytosol to the nucleus and the effect was almost as strong as that generated by a positive control ActD. The truncated form was not functional, which once again supported the presumption that, although the acidic C-tail of HMGB1 is outside the p53-binding site, the C-terminus is crucial for the properties of HMGB1 and influences the interaction between the proteins [Citation21, Citation24]. Future experiments should further explore the role of full-length HMGB1 in p53 regulation. Of course, the main question that must be answered in the future is: what signalling pathway/s is/are involved in this process?
Conclusions
Based on our experimental results, we can draw the conclusion that the stimulation of A549 cells with recombinant HMGB1 results in an increase in the p53 level in the nucleus. Our results support the conception that the C-terminus of HMGB1 is essential for the activity of HMGB1. This might be used for development of new therapeutics based on the inactive tail-less form of HMGB1 as a competitor in cancer treatment.
Disclosure statement
No potential conflict of interest was reported by the authors.
Additional information
Funding
References
- Livesey KM, Kang R, Vernon P, et al. p53/HMGB1 complexes regulate autophagy and apoptosis. Cancer Res. 2012;72:1996–2005.
- Livesey KM, Kang R, Zeh HJ, 3rd, et al. Direct molecular interactions between HMGB1 and TP53 in colorectal cancer. Autophagy. 2012;8:846–848.
- Liang SH, Clarke MF. Regulation of p53 localization. Eur J Biochem. 2001;268:2779–2783.
- Inoue K, Fry EA, Frazier DP. Transcription factors that interact with p53 and Mdm2. Int J Cancer. 2016;138:1577–1585.
- Zheng HC. The molecular mechanisms of chemoresistance in cancers. Oncotarget. 2017;8:59950–59964.
- Matsuura K, Canfield K, Feng W, et al. Metabolic regulation of apoptosis in cancer. Int Rev Cell Mol Biol. 2016;327:43–87.
- Ugrinova I, Pasheva E. HMGB1 protein: a therapeutic target inside and outside the cell. Adv Protein Chem Struct Biol. 2017;107:37–76.
- Ugrinova I, Zlateva S, Pashev IG, et al. Native HMGB1 protein inhibits repair of cisplatin-damaged nucleosomes in vitro. Int J Biochem Cell Biol. 2009;41:1556–1562.
- Topalova D, Ugrinova I, Pashev IG, et al. HMGB1 protein inhibits DNA replication in vitro: a role of the acetylation and the acidic tail. Int J Biochem Cell Biol. 2008;40:1536–1542.
- Stros M, Muselikova-Polanska E, Pospisilova S, et al. High-affinity binding of tumor-suppressor protein p53 and HMGB1 to hemicatenated DNA loops. Biochemistry. 2004;43:7215–7225.
- McKinney K, Prives C. Efficient specific DNA binding by p53 requires both its central and C-terminal domains as revealed by studies with high-mobility group 1 protein. Mol Cell Biol. 2002;22:6797–6808.
- Scaffidi P, Misteli T, Bianchi ME. Release of chromatin protein HMGB1 by necrotic cells triggers inflammation. Nature. 2002;418:191–195.
- Kostova N, Zlateva S, Ugrinova I, et al. The expression of HMGB1 protein and its receptor RAGE in human malignant tumors. Mol Cell Biochem. 2010;337:251–258.
- Mitkova E, Ugrinova I, Pashev IG, et al. The inhibitory effect of HMGB-1 protein on the repair of cisplatin-damaged DNA is accomplished through the acidic domain. Biochemistry. 2005;44:5893–5898.
- Pasheva EA, Pashev IG, Favre A. Preferential binding of high mobility group 1 protein to UV-damaged DNA. Role of the COOH-terminal domain. J Biol Chem. 1998;273:24730–24736.
- Elenkov I, Pelovsky P, Ugrinova I, et al. The DNA binding and bending activities of truncated tail-less HMGB1 protein are differentially affected by Lys-2 and Lys-81 residues and their acetylation. Int J Biol Sci. 2011;7:691–699.
- Osmanov T, Ugrinova I, Pasheva E. The chaperone like function of the nonhistone protein HMGB1. Biochem Biophys Res Commun. 2013;432:231–235.
- Choong ML, Yang H, Lee MA, et al. Specific activation of the p53 pathway by low dose actinomycin D: a new route to p53 based cyclotherapy. Cell Cycle. 2009;8:2810–2818.
- Vousden KH, Prives C. Blinded by the light: the growing complexity of p53. Cell. 2009;137:413–431.
- Yan HX, Wu HP, Zhang HL, et al. p53 promotes inflammation-associated hepatocarcinogenesis by inducing HMGB1 release. J Hepatol. 2013;59:762–768.
- Banerjee S, Kundu TK. The acidic C-terminal domain and A-box of HMGB-1 regulates p53-mediated transcription. Nucleic Acids Res. 2003;31:3236–3247.
- Logsdon CD, Fuentes MK, Huang EH, et al. RAGE and RAGE ligands in cancer. Curr Mol Med. 2007;7:777–789.
- Tesarova P, Kalousova M, Zima T, et al. HMGB1, S100 proteins and other RAGE ligands in cancer - markers, mediators and putative therapeutic targets. Biomed Pap Med Fac Univ Palacky Olomouc Czech Repub. 2016;160:1–10.
- Imamura T, Izumi H, Nagatani G, et al. Interaction with p53 enhances binding of cisplatin-modified DNA by high mobility group 1 protein. J Biol Chem. 2001;276:7534–7540.