Abstract
The pathogenesis of mycoplasmas requires their attachment to the epithelial mucosa membrane of the host cells, followed by colonisation and necrotic destruction of the submucosal tissue. The extent of this pathogenesis depends on the ability of species of Mycoplasma to effectively attach and invade the host’s tissue. In this regard, the cytadherence tip organelle has evolved within the mycoplasmas to accomplish this feat. However, species of Mycoplasma that do not possess the specialized structure remain virulent with the use of surface-membrane lipoproteins. The lipoprotein ligands bind to sulfatides and sialoglycoconjugates on the host’s mucosa membranes. This host-mycoplasma interaction, though poorly studied, appears to have a wide underlying array of complex molecular mechanisms, which after activation trigger cytadherence, immunomodulation and virulence. Mycoplasmas with their highly redundant minimal genomes display dynamic genotypic and phenotypic plasticity; a trait that has allowed them to adapt, persist and survive successfully in adverse niches through circumvention and tempering of the host’s humoral immune response. Additionally, the linkages between the mycoplasmas persistence and chronic inflammatory diseases in humans necessitate examining the host-mycoplasma interaction at the proteogenomic level. This paper provides an overview on the molecular mechanisms involved in cytadherence, surface-membrane antigenic variation and survival strategies of human urogenital mycoplasmas.
Introduction
The human urogenital Mycoplasma species are a group of strains that share R-segments (a 50-kb genomic fragment consists of the entire rRNA operon and the adjacent flanking region) with average nucleotide identities ≥97% [Citation1]. This group comprising of Mycoplasma fermentans, Mycoplasma genitalium, Mycoplasma hominis and Mycoplasma penetrans appears to be evolving towards adapting to an intracellular habitat (). A trait that might be directly related to their small size, minimal genomes, phenotypic plasticity, abilities to alter their shape, develop specialized cytadherence organelles and invade host cells. M. penetrans HF-2 has the distinct ability to actively penetrate the host cell and survive in its cytoplasm [Citation2, Citation3]. Recently, Hopfe et al. [Citation4] have demonstrated the invasive ability of M. hominis into HeLa cells. M. fermentans and M. hominis species exist extracellularly, adhering to the surface of the host cells [Citation5]. On the other hand, M. penetrans and M. genitalium strains (under certain conditions) can exist intracellularly in non-phagocytic cells [Citation6]. An intracellular existence can be seen as the ultimate strategy to survive the hostile conditions of parasitism. The phylogenetic relationship amongst the human urogenital mycoplasmas is illustrated in .
Figure 1. Phylogenetic tree of human urogenital Mycoplasma species based on the conserved gyrA gene sequence divergence. Note: The gyrA gene sequences were obtained from GenBank (NCBI) and aligned using the CLUSTAL_X. The neighbour-joining dendrogram was created using MEGA (Windows version 7.0) [Citation86]. The topology of the dendrogram was assessed by bootstrap analysis of 500 replicates. Scale indicates nucleotide divergence of 0.05.
![Figure 1. Phylogenetic tree of human urogenital Mycoplasma species based on the conserved gyrA gene sequence divergence. Note: The gyrA gene sequences were obtained from GenBank (NCBI) and aligned using the CLUSTAL_X. The neighbour-joining dendrogram was created using MEGA (Windows version 7.0) [Citation86]. The topology of the dendrogram was assessed by bootstrap analysis of 500 replicates. Scale indicates nucleotide divergence of 0.05.](/cms/asset/453bbc33-5cc5-461e-8255-dabd048016bf/tbeq_a_1607556_f0001_b.jpg)
Table 1. General features of the Mycoplasma species and strains of the human urogenital tract.
Lacking a protective peptidoglycan cell wall, the plasma membranes of Mycoplasma are exposed to their immediate environment making them vulnerable to the host’s defence system [Citation7]. However, by losing their cell walls, they have developed phenotypic plasticity through the ability to constantly change the antigenic lipoproteins in their plasma membranes. This lipoprotein variation is due to the mycoplasma’s genotypic plasticity. Their dynamic genomes, having a low G + C content, can undergo frequent DNA rearrangement [Citation7–9] (reviewed in [Citation10]). Consequently, this ability amongst other traits, has allowed the species of Mycoplasma to survive and persist within their habitat by evasion and modulation of the host’s immune system.
The host’s immune system can be modulated via activation of Toll-like receptors (TLRs) in the host’s cell membranes by mycoplasma lipoproteins. This lipoprotein (or lipopeptide) signalling leads to the activation of macrophages, monocytes and lymphocytes, which can initiate a pro-inflammatory response. M. fermentans has been shown to have an immunomodulatory effect via macrophage-activating lipopeptide 2 (MALP-2), M161Ag and P48 [Citation11]. MALP-2, a 2 kDa M161Ag lipoprotein derivative serves as the ligand, which binds to TLR2. M161Ag is reported to be a cytokine inducer for monocytes, macrophages, maturing dendritic cells (antigen-presenting cells), and host complement activation [Citation12]. Apoptosis of host cells can be induced by lipoproteins which cause the release of ATP that binds to P2X7 purinergic receptors [Citation13–15].
The sequences of the lipoproteins of mycoplasmas have been reported to be significantly homologous to the host structural proteins resulting in molecular mimicry [Citation5, Citation16]. As a result, the species of Mycoplasma not only lead to acute diseases such as acute urethritis and prostatitis [Citation17, Citation18], but also to chronic autoinflammatory diseases such as systemic lupus erythematosus, rheumatoid arthritis and psoriasis [Citation19–21]. They have also been implicated in non-autoimmune diseases such as infertility and cancer (reviewed in [Citation22]). To the contrary, some species of Mycoplasma such as M. hominis exists within the host in a non-pathogenic state [Citation15]. Pathogenicity can partially be mediated through bacteriophages [Citation21]. For example, phage ɸMFV1 encodes a unique coiled-coil membrane surface protein (Mem) that enables the pathogenicity of M. fermentans [Citation22]. Thus, the mechanisms of mycoplasma pathogenesis and virulence require further investigation.
Mycoplasmas have developed a number of strategies that allow them to persist in their habitats while maintaining minimal genomes. One such strategy is the evolution of proteins with dual functionality (adherence and catalytic properties). For example, M. genitalium G37 can attach to the mucin component of the epithelial mucus membrane via the glycolytic enzyme glyceraldehyde-3-phosphate dehydrogenase. This glycolytic enzyme thus acts as an adhesin in some species of mycoplasmas [Citation23]. Similarly, the multifunctional lipoprotein, oligopeptide permease substrate-binding protein (OppA) of M. hominis PG21 [Citation24, Citation25] and OppF of M. penetrans [Citation26] are involved in cytadherence to host cells. The reliance on its host for essential nutrients, along with the utilisation of tandem repeats and other redundant sequences for phenotypic plasticity allows mycoplasmas to maintain a minimal genome.
The minimal genome concept of mycoplasma
Mycoplasmas (class Mollicutes) are a group of Gram-negative bacteria which have the smallest self-replicating genomes and lack a protective peptidoglycan-containing cell wall (reviewed in [Citation6]). Phylogenetic classification of the prokaryotic domain indicates that the genus Mycoplasma has evolved from the low G + C-containing genomes of Gram-positive bacteria belonging to the genera: Lactobacillus, Bacillus, Streptococcus and two Clostridium species, Clostridium innocuum and Clostridium ramosum [Citation27, Citation28].
The descent of mycoplasmas is thought to be a consequence of degenerative (reductive) evolution due to their parasitic nature. They rely on their host for essential nutrients as they lack the capability to synthesize several vital carbohydrates and lipids due to a reduced and redundant genome.
Of the mycoplasmas, M. genitalium G37 was the first to be fully sequenced and is noted to have the smallest genome size (0.58Mb). The fact that it was also the smallest known self-replicating bacterium [Citation7] with little genomic redundancy (470 predicted coding regions) led to the concept of a ‘minimal gene complement’ for cellular life [Citation29].
In 1996, a comparative proteogenomic analysis between M. genitalium and Haemophilus influenzae reported that the ‘minimal gene set’ necessary to sustain cellular life consisted of at least 256 genes [Citation30], whereas Hutchison et al. [Citation31] using mutagenesis techniques identified a gene set (265–350) of the 480 protein-coding genes for M. genitalium. By 2005, the number of protein coding genes identified in M. genitalium had increased to 482 and by the use of global transposon mutagenesis experimentation, the number of essential protein coding genes was 387 [Citation32], that is 131 more genes than determined theoretically by Mushegian and Kooninin 1996 [Citation30], and 181 more than the 206-gene core of a minimal bacterial gene set previously proposed by Gil et al. [Citation33].
Later, Pereyre et al. [Citation34] working with three minimal mollicute genomes, documented that their genomes shared 247 coding sequences (CDSs) plus a set of nine energy-producing genes. The authors deduced that a minimal mycoplasma cell, without virulence factors, could theoretically contain a minimal genome of 256 genes [Citation34]. A recent comparative genomic study of four human urogenital Mycoplasma species predicted a minimal genome of 248 genes [Citation35].
For Mycoplasma pneumoniae, which infects the respiratory tract of humans and has a close evolutionary relationship with M. genitalium, its minimal genome constitutes 33% (269 kb) of the whole genome. Its minimal genome includes non-coding regions and small ORFs (<100 residues) that are essential genomic components owing to their involvement in protein and possibly DNA/RNA complexes [Citation36]. Similarly, from an evolutionary and survival perspective, within the context of their natural environment, any genes encoding for cytadherence and adhesin-related accessory proteins must be considered essential and included in the minimal gene set estimation. To date, the number of protein coding genes in M. genitalium G37 is 518 [Citation37], 52% of which are of unknown molecular function and 109 encode hypothetical proteins. Therefore, it can be opined that the determination of the smallest ‘minimal gene set’ is not feasible at this time due to the unavailability of a fully annotated genome of M. genitalium.
Virulence factors in host-mycoplasma attachment
The main virulence factor in the pathogenesis of the species of Mycoplasma is the ability of these organisms to adhere to the host’s epithelial mucus membrane and colonize the affected tissue [Citation6]. Many mycoplasmas rely on the concentration of adhesins and adhesin-related accessory proteins at the cytadherence tip organelle () for attachment and motility [Citation38–40]. None of the genes encoding the adhesins and adhesin-related accessory proteins have homologs across the various species and are not part of the core genome of these organisms. The virulence genes are in fact specific for each species of Mycoplasma, forming their own category within the Mycoplasma genome [Citation35]. It has, therefore, been speculated that the cytadherence tip organelle might indeed be a homoplasy resulting from evolutionary convergence [Citation26]. This would explain the diversity in protein composition and structural arrangement (in the tip organelle) in different Mycoplasma species.
Figure 2. Scheme of a transverse section of M. pneumoniae cytadherence tip organelle depicting location of adhesins and adhesin-related accessory proteins. Note: P1 and P30 are the primary adhesins, P40 and P90 are adhesin-related accessory proteins; high molecular weight (HMW) proteins are integrated within the plasma membrane as surface-membrane lipoproteins or associated with a central cytoskeletal like structure, HMW1 and HMW3, respectively.
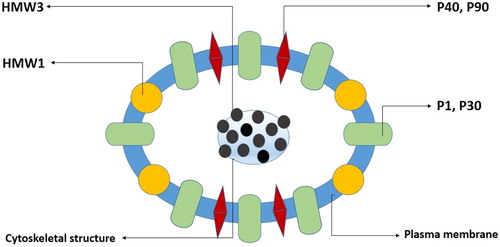
Unlike M. pneumoniae whose primary virulent adhesin is the protein P1 [Citation5, Citation41], that becomes concentrated in the tip organelle, those for the human urogenital mycoplasmas are generally unknown. Though a number of adhesins and adhesin-related accessory proteins have been identified in the human urogenital Mycoplasma species, it remains unclear as to how they are arranged and function in the tip organelle.
The immunodominant MgPa adhesin, the proline-rich proteinP65 and the P32 protein of M. genitalium are encoded by genes mgpB, MG_217 and MG_318, respectively. These correspond to P1, HMW2 and HMW3 found in the tip organelle of M. pneumoniae. By extension, it is likely that M. genitalium has a tip organelle with similarly arranged adhesins and adhesin-related accessory proteins. A high proline content in adhesin-related accessory proteins makes them more susceptible to folding and binding which are necessary properties for attachment [Citation15]. Mutants of M. genitalium, which have transposon insertions in these genes, show a reduction in motility and cytadherence [Citation40]. MG_218 of M. genitalium, which encodes a 190 kDa protein, is reported to be involved in the structural and functional stability of adhesin P140/mgPa (gene mgpB) and its cytadherence-related protein P110/mgpC (gene mgpC) since disruption of this gene by homologous recombination results in non-cytadhering phenotypes [Citation42]. Adhesins P140 and P110 stimulate humoral and cervicovaginal antibody production during M. genitalium infection. Both mgpB and mgpC produce sequence variation through recA mediated homologous recombination with MgPar donor sequences leading to antigenic diversity in these adhesins [Citation43–46]. M. genitalium attaches to mucin, a component of the host’s epithelial mucus membrane via ligands of the bacterial surface-exposed enzyme glyceraldehyde-3-phosphate dehydrogenase (GAPDH). Hence, this enzyme assumes dual roles acting both as catalysts and adhesins when required. When GAPDH is blocked by specific monoclonal antibodies, M. genitalium cytadherence ability is reduced by 70% [Citation23]. The attachment and virulence of M. genitalium are also reported to be influenced by the peptide, methionine-sulfoxide reductase A (MsrA), an antioxidant enzyme which converts oxidized methionine [Met(O)] into methionine (Met) [Citation45].
M. hominis is the second smallest (665 kb genome) self-replicating bacterium. The strain M. hominis PG21 has a genome which is only 85.3 kb larger than that of M. genitalium G37 [Citation37]. Unlike M. genitalium, M. hominis does not possess a cytadherence tip organelle. Instead, adherence is associated with an exuberant surface membrane lipoprotein, Vaa (variable adherence-associated) adhesin, that exhibits antigenic size variation (28–72 kDa) [Citation46, Citation47] and phase variation with ON/OFF expression switching at a rate of 10−3–10−4 [Citation48–51]. The N-terminal region of Vaa folds into an elongated triple helix structure containing coiled-coil domains with heptad repeats and has two beta-sheets at the C-terminus. The β-sheet region, which is located outside of the mycoplasma membrane, contains the adherence-mediating structure which likely binds to sulfoglycolipids and sialoglycoconjugates on the host cell membrane [Citation16, Citation51, Citation52]. Many physiological processes (e.g. cell–cell adhesion, signalling and differentiation) and pathological processes (e.g. metastasis) are reported to be associated with the presence of O-acetylated sialoglycoconjugates (O-AcSGs) in cell membranes [Citation51, Citation53, Citation54].
Little is also known about the cytadherence tip organelle in M. fermentans but some strains do possess such a structure as evidenced by electron microscopy [Citation54]. P29, a surface membrane lipoprotein of M. fermentans, has been identified as the molecule involved in host cell attachment. Using flow cytometric analysis, it has been demonstrated that M. fermentans is unable to bind to HeLa cells in the presence of a recombinant fusion protein, FP29, which mimics P29 [Citation55].
The middle region of the P29 sequence, which extends from amino acid 88 to 165, is reported as the region that is required for ligand binding [Citation55]. In vitro studies using HeLa cells seem to suggest that M. fermentans requires two surface membrane proteins for adhesion to cells, the protease-sensitive lipoprotein P29 and a phosphocholine-containing glycolipid [Citation56]. Plasminogen (Plg) binds to α-enolase, a glycolytic enzyme that acts as a Plg-receptor and is located on the surface membrane of M. fermentans [Citation57]. Once plasminogen is bound it is converted to plasmin by urokinase which allows M. fermentans to penetrate HeLa cells [Citation56]. This is probably due to degradation of extracellular membrane (ECM) proteins of the HeLa cells by plasmin (a serine protease). Pathogens are known to regulate the mammalian plasminogen-plasmin system [Citation58]. Interestingly, FP29 also has the capacity to block binding of M. hominis to HeLa cells which would suggest that these two organisms share a similar mechanism with regards to cell interaction [Citation55]. Studies by Rosales-Pérez et al. [Citation54] have also revealed the involvement of a putative 40 kDa protein (named as SP40 by the authors) in the adherence of M. fermentans P140 to cultured human epithelial cells (HEp-2). Anti-SP40 antibodies has been reported to prevent the clustering of M. fermentans P140 and its attachment to the HEp-2 cells as evidenced by enzyme immunoassay (EIA) technology.
M. penetrans HF-2, which has a cytadherence tip organelle [Citation2], is perhaps the only true intracellular Mycoplasma with the natural ability to actively penetrate epithelial cells within the respiratory and urogenital tracts, where it can replicate and persist for months as documented in patients with AIDS [Citation58, Citation59]. The cytadherence tip organelle of M. penetrans appears to form when plasma membrane adhesins bind to fibronectin located in the host cell membrane. This binding also results in the rearrangement of cytoskeletal proteins involved in gliding motility [Citation3]. Subunit E2 of pyruvate dehydrogenase and OppF, a surface membrane component of oligopeptide ABC transporter have been identified in the internal structure of M. penetrans tip organelle. The binding component of this ABC transporter is OppA which is known to act as an adhesin in M. hominis. OppA has not been identified in M. penetrans and as such OppF might be serving as the adhesin involved in attachment [Citation26]. Also present in the plasma membrane of M. penetrans is the highly abundant and immunogenic lipoprotein P35 [Citation60, Citation61]. Two paralogs of P35 and MYPE6960 (which has an ortholog MG_309 in M. genitalium that is known to bind and activate Toll-like receptors) have also been identified in the tip organelle. Five proteins of unknown molecular function that possess predicted alpha-helical coiled-coil regions with molecular weights greater than 90 kDa have also been reported to exist within the tip organelle [Citation26]. These properties are typical of cytoskeletal proteins, hence, they may be playing a structural role in the formation of the cytadherence tip organelle of M. penetrans.
Mycoplasma surface lipoproteins: diversity, size and phase variation
Diversity of lipoproteins
In spite of having minimal genomes, the mycoplasmas have developed genetic systems that are able to produce a wide diversity of surface membrane lipoproteins. Generally, lipoproteins consist of a surface exposed peptide moiety and a lipid moiety. The lipid moiety is integrated into the plasma membrane. It is the peptide moiety which gives rise to antigenic variation. The genetic mechanisms that lead to variation are DNA slippage utilising repeat sequences, gene duplication with antigenic drift leading to gene conversion (unidirectional recombination) and homologous recombination at specific sites resulting in shifting of motifs (reviewed in [Citation10]).
M. genitalium undergoes phase and antigenic variation in two of its surface proteins (MgpB and MgpC) based on segmental reciprocal recombination using repeat sequences [Citation8, Citation43]. RecA, which plays only a small role in DNA repair in M. genitalium, is required for the sequence variation at the mgpB/C locus [Citation62, Citation63]. Given this method of lipoprotein variation in M. genitalium, it is not surprising that its small genome contains 4% repeat sequences (termed as MgPar), with a number of simple tandem repeats and minisatellites being present in its virulence genes [Citation35, Citation64]. The frequency of reciprocal recombination using repeat sequences increases exponentially when the period of the tandem repeat increases from 8 to 20 [Citation65]. M. genitalium appears to have made good with this property by having many minisatellite insertions as oppose to simple repeat sequences in its genome [Citation35].
Lmp1 is a 135 kDa surface membrane lipoprotein of M. hominis which has multiple homologs forming a multigene family [Citation5]. Seven 471 kb tandem repeats are present in the 4032 bp ORF of the lmp gene. A single nucleotide substitution (C→A) located in the seventh repeat produces a stop codon, which leads to the gene being transcribed and translated into the Lmp1 lipoprotein. Other genetic events within the 471 kb repeats lead to Lmp2, Lmp3 and Lmp4 [Citation66]. The overall result is diversification of the lmp gene.
Gene duplication with antigenic drift leading to gene conversion is seen with surface membrane lipoprotein P120′. The P120′ gene is a duplicate of gene P120 in M. hominis but unlike the latter its gene product is weakly immunogenic and its function is unknown. The P120′ lipoprotein shows variability among isolates of M. hominis in the N-terminal exposed region of its sequence due to numerous single nucleotide polymorphisms (SNPs) within the gene [Citation67].
Size and phase variation of lipoproteins
Mycoplasmas can vary the lipoproteins size (size variation) and expression in each generation of the progeny via ON/OFF phase (phase variation). The M. hominis Vaa lipoprotein undergoes size variation to produce variants such as protein P50 which is basically a truncated variant of Vaa [Citation46]. The variants are made up of a conserved N-terminal region plus 1 to 5 interchangeable protein modules. The Vaa gene itself being composed of five gene categories separated by intergenic tandem repeats with a polyA sequence upstream (5′ end of gene). Size variation of Vaa results from the number of intragenic tandem repeats within the gene, whereas phase variation results from the number of insertions or deletions occurring within the poly A sequence that cause DNA slippage. Transcription of the vaa gene only occurs when the polyA tract is of a particular length [Citation10, Citation50].
As previously described, the rearrangement of genes and mutations within genes or repeat sequences associated with genes can produce phase variation of surface membrane lipoproteins. However, for the abundant immunogenic lipoprotein P35 of M. penetrans, a different mechanism is used to accomplish phase variation even though there are 44 paralogs of the P35 gene which form the P35 gene family [Citation60]. In studies carried out on colonies of M. penetrans, none of the above stated genetic events were identified including masking and unmasking of P35 epitopes. This has led to the conclusion that P35 may be under regulatory control at the transcription level by an unknown regulatory protein(s) [Citation61].
Survival strategies of mycoplasma
It would appear that the species of Mycoplasma with their minimal genomes have undergone evolutionary changes through natural selection to maximize survival. Both physical and chemical factors are involved in their survival strategies.
Physical factors: size, growth rate, phenotypic plasticity and intracellularity
Size and growth rate
Perhaps the first basic survival strategy relates to the size of mycoplasmas. In general, mycoplasmas are about 20% smaller than other typical bacteria. Mycoplasma being the smallest organisms are about 200 nm in width and 500 nm in length [Citation6, Citation68] compared to 1000 nm × 3000 nm for Escherichia coli [Citation69]. Based on the physical size alone, they are theoretically less likely to be attacked by the host defense system while in the presence of other larger bacteria. Also, an important property of bacteria regarding size is that smaller organisms have a slower growth rate [Citation70]. Experiments with mice infected by slowly replicating strains of lymphocytic choriomeningitis virus (LCMV) and the more rapidly replicating strains have shown that the mice infected with the slower replicating strain produced weaker CD8+-T-lymphocyte responses [Citation71] due to slow virus-antigen amplification; indicating that there is a direct correlation between virus growth kinetics and host immune response. Given that concept, Mycoplasma such as M. genitalium which have extremely slow growth rates [Citation72] may similarly allow the host immune system to adapt to the mycoplasma surface antigens hence increasing the persistence and survival of Mycoplasma.
Phenotypic plasticity and intracellularity
The constant variation in surface lipoproteins results in a membrane that is rather plastic, a feature that is key to the survival of the species of Mycoplasma. As discussed previously, Mycoplasma can vary their surface antigens by changing the size of the immunogenic lipoproteins, physically masking the lipoproteins or switching on and off the expression of the antigen [Citation44, Citation60]. Whichever mechanism is used, the result is that the host humoral immune system is evaded allowing the Mycoplasma species to persist.
The ultimate survival strategy of the mycoplasmas would be to exist and reproduce intracellularly. By doing so the organism can completely avoid the host’s defense system and save energy in the process that is required for maintaining a plastic plasma membrane. The species of human urogenital Mycoplasma appear to be transitioning towards intracellularity. However, as they can only replicate extracellularly other mechanisms have evolved for their survival. They defend themselves by intervening and manipulating the host immune response using chemical factors.
Chemical factors: immune system modulation, apoptosis, toxin release, antibiotic resistance and bacteriophage defense
Immune system modulation
Mycoplasma lipoproteins play a critical role in attachment and are engaged in triggering signalling pathways via Toll-like receptors (TLRs) on eukaryotic cells. Binding of mycoplasma diacylated and triacylated lipoproteins to TLRs induces apoptosis, activates monocytes and macrophages as well as initiate the secretion of proinflammatory substances such as tumour necrosis factor-alpha (TNF-α), nuclear factor-kappa B (NF-ƙB) interleukin-1 & 6 (IL-1 and IL-6), chemokines and macrophage inflammatory protein-1 [Citation5, Citation73, Citation74]. The MIB-MIP [Mycoplasma immunoglobulins (Ig) binding protein-Mycoplasma Ig protease] system which is encoded by two genes specific to mycoplasma has the capability to target and deactivate host immunoglobulins (IgG). MIB forms a complex with the Fv region of the host IgG which activates the serine protease, MIP, resulting in the dissociation of the VH domain from the IgG antibody [Citation75, Citation76]. Thus, Mycoplasma species evade the host defense system not only by expressing size and phase variation in immunogenic lipoproteins but also by modulating the host’s immune system.
Apoptosis and toxin release
Some Mycoplasma have employed the secretion of toxins as a survival mechanism. Mycoplasma lipoproteins can induce increase permeability in lymphocytes and monocytes leading to apoptosis, by causing the release of ATP [Citation13]. ATP regulates the cytotoxicities of the lipoproteins to the cells, possibly by the binding of ATP to receptors such as the P2X7 purinergic receptor [Citation77]. The lipoprotein OppA of M. hominis, which is an ecto-ATPase, is also responsible for the release of ATP and cell death as evidenced by studies carried out using HeLa cells [Citation78]. In the same organism, lipoproteins P60 and P80 exist as a P60/P80 complex in the membrane, which dissociates to release P80 that likely acts as a signal peptide. The latter is believed to be transported across the membrane where it interacts with the Histidine triad motif-T (HinT) which hydrolyses adenosine monophosphate (AMP) derivatives that can induce apoptosis of macrophages [Citation79].
The genomes of M. hyosynoviae strains encode virulence-associated protein D (VapD) [Citation80]. The latter has been assigned a number of different functions in virulence mechanisms including acting as a toxin [Citation81]. The gene MHO_4710 of M. hominis has been predicted to code for VapD as it contains a motif that shares 60% similarity to that found in Helicobacter pylori [Citation34]. Hence, VapD in the urogenital species, M. hominis, may also be acting as a toxin.
Antibiotic resistance and bacteriophage defense
Other factors affecting the survival of the urogenital mycoplasmas are the presence of antibiotics and bacteriophages within their environment. Having evolved without a cell wall, the species of Mycoplasma are naturally resistant to β-lactams and all other antibiotics, which act by degrading bacterial cell walls. For antibiotics that don’t target cell walls, Mycoplasma have shown great resilience as they are able to adapt and exhibit decrease in susceptibility to lincosamides and/or macrolides through genetic mutations [Citation82]. It has been documented in studies that specific mutations in parC and parE (topoisomerase IV genes) as well as in the DNA gyrase (topoisomerase II) genes gyrA and gyrB are able to induce resistance toward quinolone antibiotics (such as nalidixic acid, ciprofloxacin, and norfloxacin) in M. hominis [Citation83]. Some Mycoplasma species possess the CRISPR-Cas (clustered regularly interspaced short palindromic repeats-CRISPR associated) type II-A system [Citation84], which provides an adaptive and hereditary immunity against mobile genetic elements (bacteriophages and plasmids). In this system, small CRISPR RNAs (crRNAs) guide nucleases to complementary nucleic acids of phages which results in the degradation of the phage genomes (reviewed in [Citation85]). Though the CRISPR-Cas system may be present in the human urogenital mycoplasmas, none have so far been reported.
Conclusions
Comparative studies on mycoplasmas have confirmed that each species has a unique or specific set of virulence genes, which transcribe proteins involved in cytadherence. The interplay between these surface membrane lipoproteins in the host-mycoplasma pathogenic interaction remains unclear. Individual species have their own adherence mechanism at the molecular level. Adding further complexity to this interaction is that many of the lipoproteins have multiple functionality acting as enzymes, adhesins/adhesin-related accessory proteins and/or signalling molecules. Also, the fact that some of the major immunogenic lipoproteins (like the P35 lipoprotein used in serological assay for M. penetrans) can be totally switched off at the transcription level makes it difficult to detect these difficult-to-culture fastidious organisms in disease states. This means that infections which are caused by these organisms can go undetected. Management of patients infected by Mycoplasma can be compromised by the inability to detect and accurately genotype these organisms in a quick simple reliable manner due to antigenic phase variation. Phenotypic plasticity and formation of antibiotic resistance genes also makes it particularly challenging to design effective vaccines and antibiotics against Mycoplasma. All of these issues necessitate the application of modern genomic, transcriptomic and proteomic tools to identify robust biomarkers for real-time detection of pathogenic mycoplasmas and to elucidate the molecular function of these lipoproteins involved in pathogenesis and survival of urogenital species of Mycoplasma.
Acknowledgements
The authors thank Dr. Alvin Holder of Old Dominion University for his assistance in providing copies of some difficult-to-source research articles.
Disclosure statement
No potential conflict of interest was reported by the authors.
Funding
A part of this research was supported by UWI Campus Research Awards Fund (to BRM). OR gratefully acknowledges the support from the UWI Graduate Student Research Award.
References
- Roachford OSE, Nelson KE, Mohapatra BR. A novel approach for the identification and phylogenetic delineation of human Mycoplasma species and strains using genomic segment sequence analysis. Infect Genet Evol. 2019;68:68–76.
- Andreev J, Borovsky Z, Rosenshine I. Invasion of HeLa cells by Mycoplasma penetrans and the induction of tyrosine phosphorylation of a 145-kDa host cell protein. FEMS Microbiol Lett. 1995;132:189–194.
- Girón JA, Lange M, Baseman JB. Adherence, fibronectin binding, and induction of cytoskeleton reorganization in cultured human cells by Mycoplasma penetrans. Infect Immun. 1996;64:197–208.
- Hopfe M, Deenen R, Degrandi D, et al. Host cell responses to persistent mycoplasmas—different stages in infection of HeLa cells with Mycoplasma hominis. PLoS One. 2013;8:e54219.
- Ladefoged SA, Birkelund S, Hauge S, et al. A 135-kilodalton surface antigen of Mycoplasma hominis PG21 contains multiple directly repeated sequences. Infect Immun. 1995;63:212–223.
- Rottem S. Interaction of mycoplasmas with host cells. Physiol Rev. 2003;83:417–432.
- Razin S, Yogev D, Naot Y. Molecular biology and pathogenicity of mycoplasmas. Microbiol Mol Biol Rev. 1998;62:1094–1156.
- Razin S. The prokaryotes: a handbook on the biology of bacteria. 3rd ed. Vol. 4, Bacteria: Firmicutes, Cyanobacteria. New York (NY): Springer; 2006.
- Ma L, Jensen JS, Myers L, et al. Mycoplasma genitalium: an efficient strategy to generate genetic variation from a minimal genome. Mol Microbiol. 2007;66:220–236.
- Citti C, Nouvel LX, Baranowski E. Phase and antigenic variation in mycoplasmas. Future Microbiol. 2010;5:1073–1085.
- Seya T, Matsumoto M. A lipoprotein family from Mycoplasma fermentans confers host immune activation through Toll-like receptor 2. Int J Biochem Cell Biol. 2002;34:901–906. Aug 1
- Nishiguchi M, Matsumoto M, Takao T, et al. Mycoplasma fermentans lipoprotein M161Ag-induced cell activation is mediated by Toll-like receptor 2: role of N-terminal hydrophobic portion in its multiple functions. J Immunol. 2001;166:2610–2616.
- Into T, Okada K, Inoue N, et al. Extracellular ATP regulates cell death of lymphocytes and monocytes induced by membrane-bound lipoproteins of Mycoplasma fermentans and Mycoplasma salivarium. Microbiol Immunol. 2002;46:667–675.
- Di Virgilio F, Schmalzing G, Markwardt F. The elusive P2X7 macropore. Trends Cell Biol. 2018;28:392–404.
- Baseman JB, Tully JG. Mycoplasmas: sophisticated, re-emerging, and burdened by their notoriety. Emerg Infect Dis. 1997;3:21–32.
- Falk L, Fredlund H, Jensen JS. Signs and symptoms of urethritis and cervicitis among women with or without Mycoplasma genitalium or Chlamydia trachomatis infection. Sex Transm Infect. 2005;81:73–78.
- Dehon P, McGowin CL. The immunopathogenesis of Mycoplasma genitalium infections in women: a narrative review. Sex Transm Dis. 2017;44:428–432.
- Nicolson GL, Nasralla MY, Franco AR, et al. Diagnosis and integrative treatment of intracellular bacterial infections in chronic fatigue and fibromyalgia syndromes, Gulf war illness, rheumatoid arthritis and other chronic illnesses. Clin Pract Altern Med. 2000;1:92–102.
- Gdoura R, Kchaou W, Chaari C, et al. Ureaplasma urealyticum, Ureaplasma parvum, Mycoplasma hominis and Mycoplasma genitalium infections and semen quality of infertile men. BMC Infect Dis. 2007;7:129.
- Zarei O, Rezania S, Mousavi A. Mycoplasma genitalium and cancer: a brief review. Asian Pac J Cancer Prev. 2013;14:3425–3428.
- Wagner PL, Waldor MK. Bacteriophage control of bacterial virulence. Infect Immun. 2002;70:3985–3993.
- Röske K, Calcutt MJ, Wise KS. The Mycoplasma fermentans prophage phiMFV1: genome organization, mobility and variable expression of an encoded surface protein. Mol Microbiol. 2004;52:1703–1720.
- Alvarez RA, Blaylock MW, Baseman JB. Surface localized glyceraldehyde-3-phosphate dehydrogenase of Mycoplasma genitalium binds mucin. Mol Microbiol. 2003;48:1417–1425.
- Henrich B, Hopfe M, Kitzerow A, et al. The adherence-associated lipoprotein P100, encoded by an opp operon structure, functions as the oligopeptide-binding domain OppA of a putative oligopeptide transport system in Mycoplasma hominis. J Bacteriol. 1999;181:4873–4878.
- Hopfe M, Dahlmanns T, Henrich B. In Mycoplasma hominis the OppA-mediated cytoadhesion depends on its ATPase activity. BMC Microbiol. 2011;11:185.
- Distelhorst SL, Jurkovic DA, Shi J, et al. The variable internal structure of the Mycoplasma penetrans attachment organelle revealed by biochemical and microscopic analyses: implications for attachment organelle mechanism and evolution. J Bacteriol. 2017;199:e00069–17.
- Woese CR, Fox GE. Phylogenetic structure of the prokaryotic domain: the primary kingdoms. Proc Natl Acad Sci USA. 1977;74:5088–5090.
- Weisburg WG, Tully JG, Rose DL, et al. A phylogenetic analysis of the mycoplasmas: basis for their classification. J Bacteriol. 1989;171:6455–6467.
- Fraser CM, Gocayne JD, White O, et al. The minimal gene complement of Mycoplasma genitalium. Science. 1995;270:397–403.
- Mushegian AR, Koonin EV. A minimal gene set for cellular life derived by comparison of complete bacterial genomes. Proc Natl Acad Sci USA. 1996;93:10268–10273.
- Hutchison Iii CA, Petterson SN, Gill SR, et al. Global transposon mutagenesis and a minimal Mycoplasma genome. Science. 1999;286:2165–2169.
- Glass JI, Assad-Garcia N, Alperovich N, et al. Essential genes of a minimal bacterium. Proc Natl Acad Sci USA. 2006;103:425–430.
- Gil R, Silva FJ, Peretó J, et al. Determination of the core of a minimal bacterial gene set. Microbiol Mol Biol Rev. 2004;68:518–537.
- Pereyre S, Sirand-Pugnet P, Beven L, et al. Life on arginine for Mycoplasma hominis: clues from its minimal genome and comparison with other human urogenital mycoplasmas. PLoS Genet. 2009;5:e1000677–e1000613.
- Roachford OSE, Nelson KE, Mohapatra BR. Comparative genomics of four Mycoplasma species of the human urogenital tract: analysis of their core genomes and virulence genes. Int J Med Microbiol. 2017;307:508–520.
- Lluch-Senar M, Delgado J, Chen WH, et al. Defining a minimal cell: essentiality of small ORFs and ncRNAs in a genome-reduced bacterium. Mol Syst Biol. 2015;11:780.
- NCBI Resource Coordinators. Database resources of the National Center for Biotechnology Information. Nucleic Acids Res. 2016;44:D7–D19.
- García-Morales L, González-González L, Querol E, et al. A minimized motile machinery for Mycoplasma genitalium. Mol Microbiol. 2016;100:125–138.
- Seybert A, Gonzalez-Gonzalez L, Scheffer MP, et al. Cryo-electron tomography analyses of terminal organelle mutants suggest the motility mechanism of Mycoplasma genitalium. Mol Microbiol. 2018;108:319–329.
- Pich OQ, Burgos R, Ferrer-Navarro M, et al. Role of Mycoplasma genitalium MG218 and MG317 cytoskeletal proteins in terminal organelle organization, gliding motility and cytadherence. Microbiology. 2008;154:3188–3198.
- Ruland K, Wenzel R, Herrmann R. Analysis of three different repeated DNA elements present in the P1 operon of Mycoplasma pneumoniae: size, number and distribution on the genome. Nucleic Acids Res. 1990;18:6311–6317.
- Dhandayuthapani S, Rasmussen WG, Baseman JB. Disruption of gene mg218 of Mycoplasma genitalium through homologous recombination leads to an adherence-deficient phenotype. Proc Natl Acad Sci USA. 1999;96:5227–5232.
- Peterson SN, Bailey CC, Jensen JS, et al. Characterization of repetitive DNA in the Mycoplasma genitalium genome: possible role in the generation of antigenic variation. Proc Natl Acad Sci USA. 1995;92:11829–11833.
- Iverson-Cabral SL, Wood GE, Totten PA. Analysis of the Mycoplasma genitalium MgpB adhesin to predict membrane topology, investigate antibody accessibility, characterize amino acid diversity, and identify functional and immunogenic epitopes. PLoS One. 2015;10:e0138244.
- Dhandayuthapani S, Blaylock MW, Bebear CM, et al. Peptide methionine sulfoxide reductase (MsrA) is a virulence determinant in Mycoplasma genitalium. J Bacteriol. 2001;183:5645–5650.
- Kitzerow A, Hadding U, Henrich B. Cyto-adherence studies of the adhesin P50 of Mycoplasma hominis. J Med Microbiol. 1999;48:485–493.
- Hasebe A, Mu H-H, Cole BC. factor from Mycoplasma hominis is a TLR2-dependent, macrophage-activating, P50-related adhesin. Am J Reprod Immunol. 2014;72:285–295.
- Boesen T, Emmersen J, Jensen LT, et al. The Mycoplasma hominis vaa gene displays a mosaic gene structure. Mol Microbiol. 1998;29:97–110.
- Boesen T, Fedosova NU, Kjeldgaard M, et al. Molecular design of Mycoplasma hominis Vaa adhesin. Protein Sci. 2001;10:2577–2586.
- Mandal C, Chatterjee M, Sinha D. Investigation of 9-O-acetylated sialoglycoconjugates in childhood acute lymphoblastic leukaemia. Br J Haematol. 2000;110:801–812.
- Henrich B, Feldmann RC, Hadding U. Cytoadhesins of Mycoplasma hominis. Infect Immun. 1993;61:2945–2951.
- Varki A. Sialic acids as ligands in recognition phenomena. FASEB J. 1997;11:248–255.
- Hamaguchi T, Kawakami M, Furukawa H, et al. Identification of novel protein domain for sialyloligosaccharide binding essential to Mycoplasma mobile gliding. FEMS Microbiol Lett. 2019;366:fnz016.
- Rosales-Pérez M, Giono-Cerezo S, Girón J, et al. Adherence of a clinical strain of Mycoplasma fermentans to human cultured epithelial cells. Adv Microbiol. 2014;4:726–735.
- Leigh SA, Wise KS. Identification and functional mapping of the Mycoplasma fermentans P29 adhesin. Infect Immun. 2002;70:4925–4935.
- Yavlovich A, Katzenell A, Tarshis M, et al. Mycoplasma fermentans binds to and invades HeLa cells: involvement of plasminogen and urokinase. Infect Immun. 2004;72:5004–5011.
- Yavlovich A, Rechnitzer H, Rottem S. Alpha-enolase resides on the cell surface of Mycoplasma fermentans and binds plasminogen. Infect Immun. 2007;75:5716–5719.
- Lähteenmäki K, Edelman S, Korhonen TK. Bacterial metastasis: the host plasminogen system in bacterial invasion. Trends Microbiol. 2005;13:79–85.
- Sasaki Y, Ishikawa J, Yamashita A, et al. The complete genomic sequence of Mycoplasma penetrans, an intracellular bacterial pathogen in humans. Nucleic Acids Res. 2002;30:5293–5300.
- Ferris SL, Watson H, Neyrolles O, et al. Characterization of a major Mycoplasma penetrans lipoprotein and of its gene. FEMS Microbiol Lett. 1995;130:313–320.
- Neyrolles O, Chambaud I, Ferris S, et al. Phase variations of the Mycoplasma penetrans main surface lipoprotein increase antigenic diversity. Infect Immun. 1999;67:1569–1578.
- Iverson-Cabral SL, Astete SG, Cohen CR, et al. mgpB and mgpC sequence diversity in Mycoplasma genitalium is generated by segmental reciprocal recombination with repetitive chromosomal sequences. Mol Microbiol. 2007;66:55–73.
- Burgos R, Wood GE, Young L, et al. RecA mediates MgpB and MgpC phase and antigenic variation in Mycoplasma genitalium, but plays a minor role in DNA repair. Mol Microbiol. 2012;85:669–683.
- Ma L, Taylor S, Jensen JS, et al. Short tandem repeat sequences in the Mycoplasma genitalium genome and their use in a multilocus genotyping system. BMC Microbiol. 2008;8:130–142.
- Rocha EPC, Blanchard A. Genomic repeats, genome plasticity and the dynamics of Mycoplasma evolution. Nucleic Acids Res. 2002;30:2031–2042.
- Ladefoged SA, Jensen LT, Brock B, et al. Analysis of 0.5-kilobase-pair repeats in the Mycoplasma hominis lmp gene system and identification of gene products. J Bacteriol. 1996;178:2775–2784.
- Mardassi BBA, Ayari H, Béjaoui-Khiari A, et al. Genetic variability of the P120′ surface protein gene of Mycoplasma hominis isolates recovered from Tunisian patients with uro-genital and infertility disorders. BMC Infect Dis. 2007;7:142.
- Arraes FBM, de Carvalho MJA, Maranhão AQ, et al. Differential metabolism of Mycoplasma species as revealed by their genomes. Genet Mol Biol. 2007;30:182–189.
- Reshes G, Vanounou S, Fishov I, et al. Cell shape dynamics in Escherichia coli. Biophys J. 2008;94:251–264.
- Chien A, Hill NS, Levin PA. Cell size control in bacteria. Curr Biol. 2013;22:1–23.
- Bocharov G, Ludewig B, Bertoletti A, et al. Underwhelming the immune response: effect of slow virus growth on CD8+-T-lymphocyte responses. J Virol. 2004;78:2247–2254.
- Gibson DG, Glass JI, Lartigue C, et al. Creation of a bacterial cell controlled by a chemically synthesized genome. Science. 2010;329:52–56.
- Jan G, Fontenelle C, Le Hénaff M, et al. Acylation and immunological properties of Mycoplasma gallisepticum membrane proteins. Res Microbiol. 1995;146:739–750.
- Zuo L, Wu Y, You X. Mycoplasma lipoproteins and Toll-like receptors. J Zhejiang Univ Sci B. 2009;10:67–76.
- Grover RK, Zhu X, Nieusma T, et al. A structurally distinct human Mycoplasma protein that generically blocks antigen-antibody union. Science. 2014;343:656–661.
- Arfi Y, Minder L, Di Primo C, et al. MIB-MIP is a mycoplasma system that captures and cleaves immunoglobulin G. Proc Natl Acad Sci USA. 2016;113:5406–5411.
- Lister MF, Sharkey J, Sawatzky D, et al. The role of the purinergic P2X7 receptor in inflammation. J Inflamm. 2007;4:5.
- Hopfe M, Henrich B. OppA, the ecto-ATPase of Mycoplasma hominis induces ATP release and cell death in HeLa cells. BMC Microbiol. 2008;8:55.
- Hopfe M, Hoffmann R, Henrich BP, et al. P80, the HinT interacting membrane protein, is a secreted antigen of Mycoplasma hominis. BMC Microbiol. 2004;4:46.
- Bumgardner EA, Kittichotirat W, Bumgarner RE, et al. Comparative genomic analysis of seven Mycoplasma hyosynoviae strains. Microbiologyopen. 2015;4:343–359.
- Kwon AR, Kim JH, Park SJ, et al. Structural and biochemical characterization of HP0315 from Helicobacter pylori as a VapD protein with an endoribonuclease activity. Nucleic Acids Res. 2012;40:4216–4228.
- Hata E, Nagai K, Murakami K. Mutations associated with change of susceptibility to lincosamides and/or macrolides in field and laboratory-derived Mycoplasma californicum strains in Japan, and development of a rapid detection method for these mutations. Vet Microbiol. 2019;229:81–89.
- Bébéar CM, Renaudin H, Charron A, et al. Alterations in topoisomerase IV and DNA gyrase in quinolone-resistant mutants of Mycoplasma hominis obtained in vitro. Antimicrob Agents Chemother. 1998;42:2304–2311.
- Chylinski K, Le Rhun A, Charpentier E. The tracrRNA and Cas9 families of type II CRISPR-Cas immunity systems. RNA Biol. 2013;10:726–737.
- Burmistrz M, Pyrć K. CRISPR-Cas systems in prokaryotes. Pol J Microbiol. 2015;64:193–202.
- Kumar S, Stecher G, Peterson D, et al. MEGA-CC: Computing core of molecular evolutionary genetics analysis program for automated and iterative data analysis. Bioinformatics. 2012;28:2685–2686.