Abstract
Acetylshikonin has an inhibitory effect on various tumour cells, but its inhibitory effect and mechanism of action in melanoma have not been reported. In this study, effects of acetylshikonin on the migration and invasion of human melanoma A375 cells and their underlying mechanism were investigated. A375 cells were treated with 0, 2, 4 and 8 μmol/L acetylshikonin and the proliferation ability, apoptosis rate, apoptosis-related protein expressions, migration and invasion, epithelial-mesenchymal transition (EMT) process and the protein expressions of phosphatidylinositol 3-kinase/protein kinase B/mammalian target of rapamycin (PI3K/Akt/mTOR) signalling pathway were detected. The results showed that compared with 0 μmol/L acetylshikonin, the proliferation of A375 cells decreased and the apoptotic rate increased significantly in 2, 4 and 8 μmol/L acetylshikonin groups; meanwhile, the scratch healing rate of A375 cells increased and the number of invasive cells in the acetylshikonin-treated groups decreased significantly. Furthermore, acetylshikonin could also elevate the protein expression of Bax and the mRNA levels of E-cadherin, but lower the protein expressions of Bcl-2, matrix metalloproteinase-2 (MMP-2), MMP-9, fibronectin (FN), p-PI3K, p-Akt and p-mTOR and the mRNA levels of vimentin and N-cadherin. These results suggest that acetylshikonin can inhibit the migration and invasion of A375 cells, which may be related to its inhibiting the activation of PI3K/Akt/mTOR pathway and reversing the process of EMT.
Introduction
Melanoma is a highly malignant tumour originating from neuromelanocytes, with high mortality, easy metastasis and poor prognosis [Citation1]. In recent years, the incidence of melanoma has increased year by year, with an average annual growth rate of 3% [Citation2]. Surgical resection is the most important therapy for in situ melanoma and the 5-year survival rate of the melanoma with thickness less than 1 mm is more than 95% [Citation3]. However, when a melanoma progresses to a metastatic melanoma, it is insensitive to radiotherapy and chemotherapy, and its prognosis is extremely poor, with a median survival time about 8 ∼ 18 months [Citation4]. Thus, it is urgent to look for safe and effective drugs for its treatment. At present, natural drugs have become a new breakthrough in the field of cancer research, and traditional Chinese medicines also have a long history and rich clinical experience in antitumour treatment [Citation5]. Traditional Chinese medicines and their components have shown some antitumour potential, such as multi-target, and low drug resistance and less side effects [Citation6–8].
Lithospermum is a traditional Chinese medicine with a long medicinal history in China. It cools blood, activates blood circulation, clears heat and detoxifies and promotes rashes based on the theory of traditional Chinese medicine. Lithospermum is primarily used for the treatment of wet macula, purpura, haematuria, stranguria with turbid discharge and bloody dysentery, accumulated heat-induced constipation and large carbuncle in clinic [Citation9]. Modern pharmaceutical studies have demonstrated that Lithospermum has a therapeutic effect on burns, and cardiotonic, anti-inflammatory, antimicrobial, antiviral and antitumour effects [Citation10–12]. The main active compounds of Lithospermum are naphthoquinone pigment compounds, including shikonin, acetylshikonin, β-hydroxyisovalerylalkannin and β-dimethylacrylshikonin, among which acetylshikonin is the main antitumour active component [Citation13]. The inhibitory effect of acetylshikonin on cells of pancreatic cancer, liver cancer, stomach cancer and lung cancer has been reported [Citation14–17], but its mechanism of action is still not clarified. To date, there are no reports on the effect of acetylshikonin on human melanoma A375 cells. The aim of this study was to investigate the effects of acetylshikonin on the migration and invasion of human melanoma A375 cells and their underlying mechanism, in order to lay the foundation for the development of a new natural antitumour drug in which acetylshikonin is the main active ingredient.
Materials and methods
Materials
Acetylshikonin (purity > 97%) was purchased from Bio Purify Phytochemicals Ltd (Chengdu, Sichuan, China). Human melanoma A375 cells were purchased from ATCC cell bank. Dulbecco's Modified Eagle Medium (DMEM) and foetal bovine serum were purchased from Hyclone Company, USA. Methyl-thiazolyl-tetrazolium (MTT) was purchased from AMRESCO Company, USA. Annexin-fluorescein isothiocyante (FITC)/propidium iodide (PI) cell apoptosis detection kit was provided by Nanjing KGI Company, China. Transwell® was purchased from Costar Company, USA. Fibronectin detection kit was the product of Nanjing Jiancheng Biological Engineering Institute, China. Bax, Bcl-2, MMP-2, MMP-9, p-PI3K, p-Akt, p-mTOR and glyceraldehyde-3-phosphate dehydrogenase (GAPDH) polyclonal antibodies were purchased from Santa Cruz Company, USA. Horseradish peroxidase-labelled secondary antibody was purchased from Beijing Zhongshan Golden Bridge Biotechnology Co. Ltd., China.
Methyl-thiazolyl-tetrazolium (MTT) assay
A375 cells were routinely cultured and the density of cell solution was adjusted to 1 × 106/mL by the logarithmic growth stage. The cells were cultured at a density of 200 μL per well in 96-well cell culture plates for 24 h, and then the culture medium was discarded; 180 μL new culture medium was added into each well and then 20 μL acetylshikonin at the different concentrations was added into each well, respectively, in which the final concentration was respectively 0, 2, 4 and 8 μmol/L, and the total volume was 200 μL. The cells were continuously cultured for 24, 48 and 72 h, respectively. Then, 20 μL MTT was added into each well and the cells were continuously cultured for 4 h. The culture medium was discarded, then 150 μL dimethyl sulphoxide were added into each well, and after the dimethyl sulphoxide was dissolved by fully shaking the plate, the absorbance values were measured at 490 nm and the cell proliferation was calculated.
Flow cytometry
A375 cells were seeded at a density of 5 × 105 cells per well in 6-well cell culture plates, and 24 h later, the cells completely adhered to the wall of wells. After the culture medium was carefully discarded, 1.8 mL new culture medium was added into each well and then 200 μL acetylshikonin at the different concentrations was added into each well, in which the final concentration was 0, 2, 4 and 8 μmol/L, respectively. The cells were continuously cultured in a CO2 incubator for 48 h. The cells were collected after being digested with trypsin and the cell concentration was adjusted to 1 × 105/mL. The cells in 1 mL of the cell suspension were washed with PBS twice and then were re-suspended with 200 μL binding buffer; 10 μL Annexin-V was added to them in a dark place and the samples were left standing for 10 min. Then 5 μL PI was added to the cells, continuously left standing for 5 min, and then the apoptosis of cells was measured and analysed by flow cytometry (FACSC anto II, BD Biosciences, USA).
Scratch test
A375 cells were seeded at a density of 5 × 105 per well in 6-well cell culture plates. After the cells were cultured for 24 h and adhered to the wall of cells, a transverse scratch was made on the back of the culture plates with the head of a pipette. The exfoliated cells were carefully washed off with phosphate buffer saline (PBS), and then acetylshikonin at different concentrations (0, 2, 4, 8 μmol/L) were added into the wells and the cells were cultured for 48 h. The cell culture plate was taken out, the culture medium was discarded, and the cells were photographed under an inverted microscope to observe the scratch healing and calculate the wound healing rate.
Cell invasion assay
The cell invasion experiment was performed in transwell chamber. Twenty-five microlitres of Matrigel™ were spread on the microporous polycarbonate filter membrane between the upper and lower chambers, and after the matrigel was dry, 50 μL A375 cell suspension was added into the upper chamber (5 × 105/chamber) and then acetylshikonin (0, 2, 4, 8 μmol/L) was added into it, respectively. The cells were cultured for 48 h; then the filter membrane was taken out, and after the fixation, staining and other steps, five visual fields were randomly selected for the observation on the number of invasive cells under a microscope (200×).
Real-time quantitative polymerase chain reaction (real-time qPCR)
The total RNA of A375 cells treated with acetylshikonin (0, 2, 4, 8 μmol/L) for 48 h was extracted by the TRIzol method and reverse transcripted to cDNA by reverse transcriptase. The real-time qPCR reaction system included 2 μL cDNA, upstream and downstream primers, 1 μL each, 10 μL 2 × SYBR Green Mix and 6 μL distilled water. The primers () were synthesized by Dalian Shenggong Bioengineering Co., Ltd and real-time qPCR was performed on the Applied Biosystems 2720 Thermal Cycler (Thermo Scientific, USA). The relative expression of vimentin, N-cadherin and E-cadherin mRNA was detected by using 2−ΔΔCt method, in which GAPDH was taken as the internal reference gene.
Table 1. Oligonucleotide sequences of primers used in real-time qPCR.
Enzyme-linked immunosorbent assay (ELISA)
The A375 cell cultures were centrifuged at 1000 rpm (Centrifuge 5424R, Eppendorf, Germany) for 10 min to collect the supernatants of cells treated with the different concentrations of acetylshikonin (0, 2, 4, 8 μmol/L) for 24, 48 and 72 h. The expression level of fibronectin in the supernatants of cells treated at the different times was detected according to the operation steps in the instructions of the ELISA detection kit (Jiancheng Biological Engineering Institute, China).
Western blot
The culture liquids of A375 cells treated with acetylshikonin (0, 2, 4, 8 μmol/L) for 48 h were centrifuged to extract the total protein, and the protein concentrations were determined by the BCA method. After the thermal denaturation of proteins, 20 μg of the protein samples were loaded on a 12% sodium dodecyl sulphate-polyacrylamide gel electrophoresis (SDS-PAGE) electrophoresis instrument, then the proteins were transferred onto polyvinylidene fluoride (PVDF) membranes after the electrophoresis, followed by adding the primary antibody (1: 500) and the secondary antibody (1: 1000), and enhanced chemiluminescent (ECL) was used for the colour development and the exposure; GAPDH was taken as the internal reference and the ratio of the grey values was used to represent the expression of GAPDH protein.
Statistical analysis
All the data were expressed as mean values with standard deviation (± SD) and SPSS17.0 software was used for the statistical analysis. The means of two samples were compared by t test. Analysis of variance was used to compare the means of multiple samples, and α = 0.05 or α = 0.01 was the significant test level.
Results and discussion
Inhibiting the proliferation and inducing the apoptosis of acetylshikonin in A375 cells
Melanoma mainly occurs in the skin, as well as in the mouth, respiratory tract, digestive tract, reproductive system and other mucous membranes, accounting for about 1% of malignant skin tumours [Citation18]. Although it can be cured by surgical excision, it is easy for the melanoma at its early stage to be ignored by patients, and it is often diagnosed at an advanced stage. An advanced melanoma is prone to metastasis, poor prognosis and high mortality. Besides the insensitivity to chemotherapy, radiotherapy and immunotherapy, the poor prognosis of melanoma is also related to its strong migration and invasion [Citation19,Citation20]. In recent years, the research on chemotherapeutic drugs for melanoma has never been interrupted, but no significant progress has been made, so it is very important to find safe and effective anti-melanoma drugs. The results of the MTT experiment showed that, compared with the proliferation in the 0 μmol/L acetylshikonin group, the proliferation of A375 cells in 2, 4 and 8 μmol/L acetylshikonin groups decreased significantly after the treatment for 24, 48 and 72 h (p < 0.05 or p < 0.01) in a concentration- and time-dependent manner. This indicated that acetylshikonin could inhibit the proliferation of A375 cells (). Flow cytometry was used to detect the A375 cells treated with the different concentrations of acetylshikonin for 48 h. The results showed that, the apoptotic rate of A375 cells in 2, 4 and 8 μM acetylshikonin groups increased significantly compared with that in the 0 μmol/L acetylshikonin group, after the treatment for 48 h (p < 0.01, ). Western blot was used for the further analysis on the expression of apoptosis-associated proteins. The results showed that the expression of Bax increased and that of Bcl-2 decreased significantly in A375 cells treated with the different concentrations of acetylshikonin for 48 h (p < 0.01), indicating that acetylshikonin could induce the apoptosis of A375 cells ().
Figure 1. Effects of acetylshikonin on the proliferation and apoptosis of A375 cells. Cell proliferative ability (A); apoptosis rate (B); flow cytometry analysis (C); western blot analysis (D); relative expression of Bax (E) and Bcl-2 (F). Each value is presented as mean ± SD (n = 5); *p < 0.05, vs. 0 μmol/L acetylshikonin group; **p < 0.01, vs. 0 μmol/L acetylshikonin group.
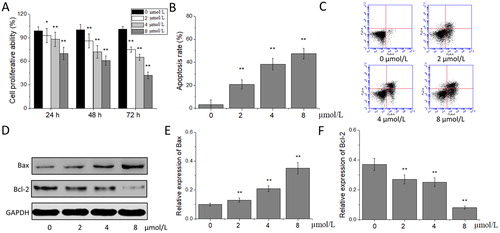
Effects of acetylshikonin on the migration and invasion of A375 cells
The postoperative recurrence and visceral metastasis are the primary causes for melanoma deaths, so finding ways to inhibit the metastasis and invasion of melanoma cells and prevent the recurrence and metastasis has become one of the focus areas in the treatment of melanoma currently [Citation21]. Due to these reasons, we investigated the effect of acetylshikonin on the migration and invasion of A375 cells by cell scratch test and Transwell® invasion test. In this study, the rate of cell scratch healing and the number of invasive cells was significantly decreased in the acetylshikonin-treated groups, suggesting that acetylshikonin can inhibit the migration and invasion of A375 cells.
Extracellular matrix (ECM) is closely related to the migration and invasion of tumour cells, and matrix metalloproteinases (MMPs) play an important role in regulating the metabolic balance of ECM [Citation22]. MMP-2 and MMP-9 can accelerate the growth and diffusion of tumour cells by promoting the angiogenesis and endogenesis of capillaries, and on the other hand, specifically degrade collagen IV, the main component of the basement membrane, resulting in the loss of integrity of the basement membrane [Citation23]. Studies have shown that exogenous MMP-2 and MMP-9 inhibitors can significantly inhibit the migration and invasion of tumour cells [Citation24]. Lu et al. [Citation25] confirmed that silibinin could inhibit the migration and invasion of human gastric cancer SGC7901 cells, and the mechanism was related to the down-regulation of the expression of MMP-2 and MMP-9. In our study, the results of the cell scratch test showed that, compared with that in the 0 μmol/L acetylshikonin group, the scratch healing rate of A375 cells in 2, 4 and 8 μmol/L acetylshikonin groups increased significantly and concentration-dependently after the treatment for 48 h (p < 0.05 or p < 0.01), suggesting that acetylshikonin might inhibit the migration of A375 cells (). The results of the cell invasion test showed that after the treatment with the different concentrations of acetylshikonin for 48 h, compared with that in the 0 μmol/L acetylshikonin group, the number of invasive cells in the acetylshikonin-treated groups decreased significantly in a concentration-dependent manner (p < 0.05 or p < 0.01), indicating that acetylshikonin could inhibit the invasion of A375 cells (). The results of the western blot showed that the expression of MMP-2 and MMP-9 in the acetylshikonin-treated groups decreased significantly compared with that in the 0 μmol/L acetylshikonin group (p < 0.01) after the treatment with the different concentrations of acetylshikonin for 48 h (). This demonstrated that acetylshikonin could inhibit the expression of MMP-2 and MMP-9 in A375 cells to some extent.
Figure 2. Effects of acetylshikonin on the migration and invasion of A375 cells. Cell scratch wound healing assay (A); cell invasion assay (B); healing rate (C); invasive cells (D); western blot analysis (E); relative expression of MMP-2 (F) and MMP-9 (G). Each value is presented as mean ± SD (n = 5); *p < 0.05, vs. 0 μmol/L acetylshikonin group; **p < 0.01, vs. 0 μmol/L acetylshikonin group.
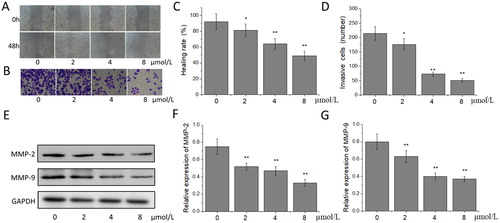
Effects of acetylshikonin on the expression of epithelial-mesenchymal transition (EMT)-related molecular markers in A375 cells
Tumour migration and invasion are multi-step processes, including detachment from the primary tumour site, invasion of the extracellular matrix, lymphatic vessels and blood vessels, adherence to the vasculature with platelets and endothelial cells and formation of new migration foci [Citation26,Citation27]. In recent years, a large number of basic studies and clinical trials have shown that the EMT process is always involved in the metastasis and invasion of melanoma, lung cancer, breast cancer, liver cancer and other gastrointestinal cancers, and plays an important role in these processes [Citation28–30]. In the EMT process, epithelial cells are transformed into interstitial cells in a specific microenvironment, and their epithelial polarity is lost, the adhesion among the cells is weakened and the epithelial characteristics are lost, particularly primarily characterized by the up-regulation of vimentin expression and the down-regulation of N-cadherin expression [Citation31,Citation32]. We used real-time qPCR to detect the expression of EMT-related molecular markers vimentin, N-cadherin and E-cadherin mRNA, and found that, compared with those in the 0 μmol/L acetylshikonin group, the expression levels of vimentin and N-cadherin mRNA decreased significantly, and those of E-cadherin mRNA increased significantly in A375 cells treated with the different concentrations of acetylshikonin for 48 h (p < 0.01, ). In addition, fibronectin is an extracellular matrix glycoprotein involved in the adhesion and migration between the cells and the matrix, as well as among the cells, and decreased fibronectin levels can inhibit the process of EMT [Citation33]. The dynamic changes of fibronectin levels in A375 cells treated with acetylshikonin were investigated by ELISA, and the results showed that compared with that in the 0 μmol/L acetylshikonin group, the expression of fibronectin decreased significantly at all the time points in A375 cells treated with the different concentrations of acetylshikonin for 24, 48 and 72 h (p < 0.01, ).
Figure 3. Effects of acetylshikonin on the expression of EMT-related molecular markers in A375 cells. Relative mRNA expression of vimentin (A), N-cadherin (B) and E-cadherin (C); expression of fibronectin (D). Each value is presented as mean ± SD (n = 5); *p < 0.05, vs. 0 μmol/L acetylshikonin group; **p < 0.01, vs. 0 μmol/L acetylshikonin group.
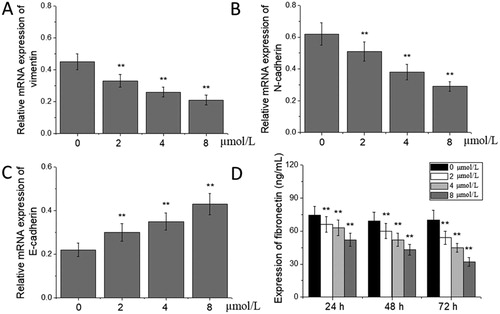
Effects of acetylshikonin on the PI3K/Akt/mTOR signalling pathway in A375 cells
Various stimuli in tumour microenvironment can induce the EMT process of tumour cells through different intracellular signalling pathways, in which the PI3K/Akt/mTOR pathway plays a key role in regulating the EMT process of tumour cells [Citation34]. PI3K/Akt/mTOR pathway is a central pathway that promotes the growth, movement, protein synthesis, survival and metabolism of cells, and can be affected by hormones, growth factor and nutrition [Citation35]. PI3K can activate Akt, and this kinase, in turn, causes the phosphorylation and activation of mTOR by cascading regulators [Citation36]. A lot of evidence indicates that a sustained activation of the PI3K/Akt/mTOR pathway is one of the main characteristics of the EMT process in tumour cells [Citation37]. Feng et al. [Citation38] found that thymoquinone could induce cytotoxic effects on gastric cancer cells and inhibit the EMT process of gastric cancer cells by targeting the PI3K/Akt/mTOR pathway. Baek et al. [Citation39] also found that ginkgolic acid could inhibit the EMT process of lung cancer cells by inactivating the PI3K/Akt/mTOR pathway, finally playing an inhibitory role in the migration and invasion of lung cancer cells. This study also found that compared with those in the 0 μmol/L acetylshikonin group, the expression levels of p-PI3K, p-Akt and p-mTOR in the acetylshikonin-treated groups were lowered, of which the levels of p-PI3K and p-mTOR decreased significantly in the 2, 4 and 8 μmol/L acetylshikonin groups, and those of p-Akt in the 4 and 8 μmol/L acetylshikonin groups decreased significantly (p < 0.05 or p < 0.01, ). The results indicated that acetylshikonin could block the activation of PI3K/Akt/mTOR pathway and then inhibit the EMT process in A375 cells.
Figure 4. Effects of acetylshikonin on the PI3K/Akt/mTOR signalling pathway in A375 cells. Western blot analysis (A); relative expression of p-PI3K (B), p-Akt (C) and p-mTOR (D). Each value is presented as mean ± SD (n = 5); *p < 0.05, vs. 0 μmol/L acetylshikonin group; **p < 0.01, vs. 0 μmol/L acetylshikonin group.
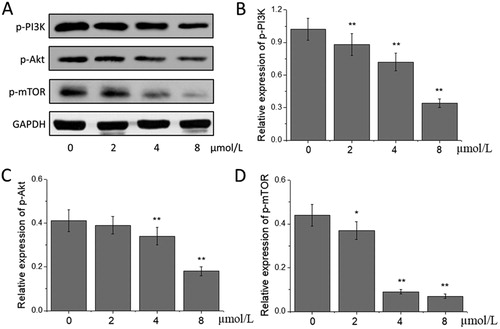
Conclusions
This study provided evidence that acetylshikonin can inhibit the proliferation and induce the apoptosis of human melanoma A375 cells. Acetylshikonin can also inhibit the migration and invasion of A375 cells. These effects may be related to its inhibiting the activation of the PI3K/Akt/mTOR pathway and reversing the process of EMT.
Disclosure statement
No potential conflict of interest was reported by the authors.
Additional information
Funding
References
- Diernaes J, Heje M, Pfeiffer-Jensen M, et al. Non-melanoma skin cancer as a possible adverse event due to anti-interleukin-6 treatment. Scand J Rheumatol. 2018;29:1–3.
- Qi RQ, He L, Zheng S, et al. BRAF exon 15 T1799A mutation is common in melanocytic nevi, but less prevalent in cutaneous malignant melanoma, in Chinese Han. J Invest Dermatol. 2011;131:1129–1138.
- Amaral T, Tampouri I, Garbe C. How to use neoadjuvant medical treatment to maximize surgery in melanoma. Expert Rev Anticancer Ther. 2018;18:121–130.
- Postlewait LM, Farley CR, Seamens AM, et al. Morbidity and outcomes following axillary lymphadenectomy for melanoma: weighing the risk of surgery in the era of MSLT-II. Ann Surg Oncol. 2018;25:465–470.
- Yang G, Qiu J, Wang D, et al. Traditional Chinese medicine curcumin sensitizes human colon cancer to radiation by altering the expression of DNA repair-related genes. Anticancer Res. 2018;38:131–136.
- Chen ST, Lee TY, Tsai TH, et al. Traditional Chinese medicine Danggui Buxue Tang inhibits colorectal cancer growth through induction of autophagic cell death. Oncotarget. 2017;8:88563–88574.
- Mhaidat NM, Alali FQ, Matalqah SM, et al. Inhibition of mek sensitizes paclitaxel-induced apoptosis of human colorectal cancer cells by downregulation of grp78. Anti-Cancer Drugs. 2009;20:601–606.
- Mollica A, Stefanucci A, Feliciani F, et al. Delivery methods of camptothecin and its hydrosoluble analogue irinotecan for treatment of colorectal cancer. Curr Drug Deliv. 2012;9:122–131.
- Lou CW, Chang CY, Wu ZH, et al. The optimal extracting process, manufacturing technique and biological evaluation of lithospermum erythrorhizon, microcapsules. Mater Sci Eng C Mater Biol Appl. 2015;48:165–171.
- Yoo HG, Lee BH, Kim W, et al. Lithospermum erythrorhizon extract protects keratinocytes and fibroblasts against oxidative stress. J Med Food. 2014;17:1189–1196.
- Rajasekar S, Park DJ, Park C, et al. In vitro and in vivo anticancer effects of lithospermum erythrorhizon extract on b16f10 murine melanoma. J Ethnopharmacol. 2012;144:335–345.
- Yang Y, Zhao D, Yuan K, et al. Two new dimeric naphthoquinones with neuraminidase inhibitory activity from. Nat Prod Res. 2015;29:908–913.
- Pietrosiuk A, Furmanowa M, Skopińiskarózewska E, et al. The effect of acetylshikonin isolated from lithospermum canescens roots on tumor-induced cutaneous angiogenesis. Acta Pol Pharm. 2014;61:379–382.
- Cho SC, Bu YC. Acetylshikonin inhibits human pancreatic panc-1 cancer cell proliferation by suppressing the nf-κb activity. Biomol Ther. 2015;23:428–433.
- Moon J, Koh SS, Malilas W, et al. Acetylshikonin induces apoptosis of hepatitis B virus x protein-expressing human hepatocellular carcinoma cells via endoplasmic reticulum stress. Eur J Pharmacol. 2014;735:132–140.
- Zeng Y, Liu G, Zhou LM. Inhibitory effect of acetylshikonin on human gastric carcinoma cell line SGC-7901 in vitro and in vivo. World J Gastroenterol. 2009;15:1816–1820.
- Lee HJ, Lee HJ, Magesh V, et al. Shikonin, acetylshikonin, and isobutyroylshikonin inhibit vegf-induced angiogenesis and suppress tumor growth in lewis lung carcinoma-bearing mice. Yakugaku Zasshi. 2008;128:1681–1688.
- Burgard B, Schöpe J, Holzschuh I, et al. Solarium use and risk for malignant melanoma: Meta-analysis and evidence-based medicine systematic review. Anticancer Res. 2018;38:1187–1199.
- Aimvijarn P, Palipoch S, Okada S, et al. Thai water lily extract induces B16 melanoma cell apoptosis and inhibits cellular invasion through the role of cellular oxidants. Asian Pac J Cancer Prev. 2018;19:149–153.
- Hepner A, Salgues A, Anjos CAD, et al. Treatment of advanced melanoma - a changing landscape. Rev Assoc Med Bras (1992). 2017;63:814–823.
- Liu J, Qu X, Shao L, et al. Pim-3 enhances melanoma cell migration and invasion by promoting STAT3 phosphorylation. Cancer Biol Ther. 2018;25:1–9.
- Golan K, Vagima Y, Goichberg P, et al. MT1-MMP and RECK: opposite and essential roles in hematopoietic stem and progenitor cell retention and migration. J Mol Med. 2011;89:1167–1174.
- Kun-Peng Z, Chun-Lin Z, Xiao-Long M. Antisense lncRNA FOXF1-AS1 promotes migration and invasion of osteosarcoma cells through the FOXF1/MMP-2/-9 pathway. Int J Biol Sci. 2017;13:1180–1191.
- Cho HJ, Park JH, Nam JH, et al. Ascochlorin suppresses MMP-2-mediated migration and invasion by targeting FAK and JAK-STAT signaling cascades. J Cell Biochem. 2018;119:300–313.
- Lu S, Zhang Z, Chen M, et al. Silibinin inhibits the migration and invasion of human gastric cancer SGC7901 cells by downregulating MMP-2 and MMP-9 expression via the p38MAPK signaling pathway. Oncol Lett. 2017;14:7577–7582.
- Xu L, Chen J, Lin W, et al. Melanoma differentiation-associated gene-7 suppresses human gastric cancer cell invasion and migration. Oncol Lett. 2017;14:7139–7144.
- Yang J, Zhu T, Zhao R, et al. Caveolin-1 inhibits proliferation, migration, and invasion of human colorectal cancer cells by suppressing phosphorylation of epidermal growth factor receptor. Med Sci Monit. 2018;24:332–341.
- Tang CP, Zhou HJ, Qin J, et al. MicroRNA-520c-3p negatively regulates EMT by targeting IL-8 to suppress the invasion and migration of breast cancer. Oncol Rep. 2017;38:3144–3152.
- Huang Y, Chen Y, Lin X, et al. Clinical significance of SLP-2 in hepatocellular carcinoma tissues and its regulation in cancer cell proliferation, migration, and EMT. Onco Targets Ther. 2017;10:4665–4673.
- Chen X, Peng H, Xiao J, et al. Benzo(a)pyrene enhances the EMT-associated migration of lung adenocarcinoma A549 cells by upregulating Twist1. Oncol Rep. 2017;38:2141–2147.
- Zhang J, Liu D, Feng Z, et al. MicroRNA-138 modulates metastasis and EMT in breast cancer cells by targeting vimentin. Biomed Pharmacother. 2016;77:135–141.
- Tanaka T, Goto K, Iino M. Sec8 modulates TGF-β induced EMT by controlling N-cadherin via regulation of Smad3/4. Cell Signal. 2017;29:115–126.
- Agajanian M, Runa F, Kelber JA. Identification of a PEAK1/ZEB1 signaling axis during TGFβ/fibronectin-induced EMT in breast cancer. Biochem Biophys Res Commun. 2015;465:606–612.
- Sun C, Tao Y, Gao Y, et al. F-box protein 11 promotes the growth and metastasis of gastric cancer via PI3K/AKT pathway-mediated EMT. Biomed Pharmacother. 2018;98:416–423.
- Gao W, Han J. Overexpression of ING5 inhibits HGF-induced proliferation, invasion and EMT in thyroid cancer cells via regulation of the c-Met/PI3K/Akt signaling pathway. Biomed Pharmacother. 2018;98:265–270.
- Li X, Teng S, Zhang Y, et al. TROP2 promotes proliferation, migration and metastasis of gallbladder cancer cells by regulating PI3K/AKT pathway and inducing EMT. Oncotarget. 2017;8:47052–47063.
- Liu X, Li Z, Song Y, et al. AURKA induces EMT by regulating histone modification through Wnt/β-catenin and PI3K/Akt signaling pathway in gastric cancer. Oncotarget. 2016;7:33152–33164.
- Feng LM, Wang XF, Huang QX. Thymoquinone induces cytotoxicity and reprogramming of EMT in gastric cancer cells by targeting PI3K/Akt/mTOR pathway. J Biosci. 2017;42:547–554.
- Baek SH, Ko JH, Lee JH, et al. Ginkgolic acid inhibits invasion and migration and TGF-β-induced EMT of lung cancer cells through PI3K/Akt/mTOR inactivation. J Cell Physiol. 2017;232:346–354.