Abstract
The most serious citrus postharvest diseases are citrus green mould caused by Penicillium digitatum and citrus blue mould caused by Penicillium italicum. Marine microorganisms have become a new measure in the control of these diseases. In this study, we isolated 56 marine actinomycetes, and strain AM-4 isolated from Drupa granulata exhibited the strongest antagonistic activity against P. digitatum. Molecular, morphological, cultural and physio-biochemical analysis identified strain AM-4 as Streptomyces chumphonensis. Strain AM-4 also exhibited antagonistic activity against 11 other phytopathogens, with an inhibition zone width ranging from 0.65 ± 0.04 to 2.35 ± 0.02 cm. The mycelial growth inhibition rates of the fermentation liquid of strain AM-4 against P. digitatum and P. italicum were 66.23% and 61.42%, respectively, and the conidial germination inhibition rates were 65.77% and 62.13%, respectively. The relative control efficacy of the gradient-diluted fermentation extracts of strain AM-4 against citrus green mould caused by P. digitatum ranged from 77.95% to 92.91% at 5 days post inoculation and 56.30% to 87.22% 10 days after inoculation. The relative control efficacy of the gradient diluted fermentation extracts of strain AM-4 against citrus blue mould caused by P. italicum ranged from 61.32% to 84.91% 5 days after inoculation and from 51.34% to 80.92% 10 days after inoculation. The relative control efficacy of the fermentation extracts of strain AM-4 was even better than prochloraz against citrus green mould caused by prochloraz-resistant P. digitatum. Therefore, as a biological control strain, AM-4 has a good prospect for development and utilization potential.
Introduction
Great economic loss of citrus fruit occurs postharvest during its storage, transport and stocking before sale. Decay is often caused by physiological disorder of the fruit tissue, pathogenic microorganism invasion and mechanical damage. Among them, losses of untreated fruit from fungal decay have been estimated to be as high as 90% during postharvest handling and marketing [Citation1]. The known citrus postharvest diseases caused by pathogenic fungi include green mould caused by Penicillium digitatum, blue mould caused by Penicillium italicum, Diplodia stem-end rot caused by Lasiodiplodia theobromae, Phomopsis stem-end rot caused by Diaporthe citri, anthracnose caused by Colletotrichum gloeosporioides and sour rot caused by Geotrichum citri-aurantii [Citation2]. Blue and green moulds, specifically, are severe postharvest diseases of citrus fruits, which are responsible for losses of up to 25% of the total production worldwide [Citation3].
At present, the control of citrus postharvest diseases mainly depends on chemical pesticides, including prochloraz, imazalil and iminoctadine tris (albesilate). However, in addition to environmental pollution and pesticide residues, the long-term and large use of chemical pesticides may also induce pathogenic microorganisms to develop resistance. For example, some studies have shown that P. digitatum has developed universal resistance to prochloraz and imazalil [Citation4–6], thus their control efficacy on citrus green mould has declined or even frequently failed [Citation7]. Consequently, the discovery and development of new biocontrol agents for the prevention and control of these citrus postharvest diseases is necessary.
More and more attention has been paid to microbial pesticides due to them having the characteristics of high efficiency, low toxicity, low residue and difficulty in generating resistance. At present, a number of antagonistic microorganisms against citrus postharvest pathogenic fungi have been isolated from the terrestrial environment, such as the rhizosphere, plant interior, fruit surface and soil, which are mainly bacteria and yeast; a few are terrestrial actinomycetes [Citation8–10]. However, it has become increasingly difficult to discover novel microorganisms, because terrestrial resources have been extensively screened and studied [Citation11, Citation12].
The environmental conditions of the ocean differ vastly from terrestrial environments and thus, marine microorganisms have unique and divergent characteristics compared to their terrestrial counterparts [Citation13–16]. Consequently, marine microorganisms have attracted much attention for the isolation of novel microorganisms and compounds that could be used to combat plant pathogens [Citation17, Citation18]. However, there has been a lack of studies regarding the application of marine actinomycetes and their metabolites in the control of citrus postharvest decay to date.
Here, in order to screen the strains with strong antagonistic activity, broad inhibition spectrum and potential as biological control strains against citrus postharvest diseases, marine actinomyces were isolated from samples collected from the seashore of Dachen Island located in Zhejiang Province, China. Their antagonistic activities were determined by the plate stand-opposite culture method and the control efficacy of the crude extracts of their fermentation products were studied on two postharvest diseases, citrus blue and green mould.
Materials and methods
Sampling and isolation of marine actinomycetes
Marine samples, consisting of fish, shellfish and shrimp, as well as intertidal sand and mud, were collected along the seashore of Dachen Island (). These samples were kept on ice in a cooler at the field and then brought to the laboratory within 24 h.
Table 1. Marine actinomycetes isolated with different media and procedures.
The formulation of each medium that was used to isolate actinomycete is provided in . Artificial seawater was prepared by dissolving 40 g of sea salt (Sigma-Aldrich, St. Louis, MO, USA) in 1000 mL sterile water. All six media were supplemented with the antibiotics nalidixic acid (15 µg/mL), nystatin (50 µg/mL) and actinomycin (50 µg/mL). Two procedures were used to isolate actinomycetes from the samples. Procedure A: 1 g of each sample was suspended in 4 mL of sterile water, which was shaken vigorously at 55 °C for 10 min and diluted to a 1:4 ratio. Procedure B: 1 g of each sample was suspended in 20 mL sterile seawater, mixed with 20 glass beads (3–5 mm in diameter), shaken vigorously at 42 °C for 30 min and serially diluted in sterile seawater to 10−3. Twenty µL of diluted samples were then spread evenly over Petri dishes containing one of the six different culture media and incubation was carried out at 28 °C for 2–3 weeks until actinomycetes colonies were observed. Actinomycetes colonies were purified by inoculating aerial mycelia over the surface of actinomycete isolation agar.
Table 2. Composition of media used to isolate marine actinomycetes.
Phytopathogens and cultivation
The P. digitatum, P. italicum, C. gloeosporioides and D. citri were isolated from diseased citrus fruits. The Pestalotiopsis mangiferae was isolated from diseased Chinese bayberry branches. The Alternaria solani was isolated from diseased tomato leaves. The Colletotrichum orbiculare was isolated from diseased watermelon fruits. The Colletotrichum graminicola and Fusarium graminearum were isolated from diseased maize leaves and stalks, respectively. The Botrytis cinerea and Fusarium oxysporum f. sp. cucumerium were isolated from diseased cucumber fruits and roots, respectively. The Gibberella zeae were isolated from diseased wheat heads. Pure cultures of the above phytopathogens were maintained in PDA slants and stored at 4 °C. One mycelial plug of each pathogen was centrally inoculated in PDA medium and cultured at 28 °C for 5–7 days for further use.
Screening of antagonistic actinomycetes
Pathogenic P. digitatum, the main postharvest pathogen, was used as an indicative fungus to screen antagonistic actinomycetes. P. digitatum and the isolated marine actinomycetes were inoculated on potato dextrose agar (PDA) and Gauze’s medium No. 1, respectively, and cultured at 28 °C for 5–7 days. A 5 mm in diameter mycelial plug was excised from the growing margins of the colony using a sterile hole puncher. The P. digitatum plug was transferred to the center of a fresh PDA plate and the marine actinomycetes plugs were placed around the pathogen plug. The PDA plate that was inoculated with only the P. digitatum plug was used as a control. The inoculated plates were then cultured at 28 °C in an incubator. Three replicates for each treatment were conducted. The width of the inhibition zone was measured when the control P. digitatum colony grew to the edge of the Petri dish. Actinomycete strains with strong antagonistic activity were screened according to the inhibition zone width. The inhibitory effect of the screened antagonistic strains against other plant pathogens were tested using the same method.
Inhibition activity of the fermentation fluid
The antagonistic strain was inoculated into liquid Gauze’s medium No. 1 and incubated at 28 °C on a shaking incubator at 200 rpm/min for 7 days. The product of the fermentation was centrifuged at 12,000 rpm/min for 10 min and then vacuum-filtered through a 0.22 μm sterile filter using a Buchner funnel. A 2 mL aliquot of filtrate was mixed evenly with 10 mL of melted PDA medium, poured into a sterile Petri dish and allowed to solidify. A 5 mm in diameter mycelial plug was excised from the growing margins of both the P. digitatum and P. italicum colonies and transferred to the center of PDA plates containing the sterile fermentation fluid of the antagonistic strain. PDA plates without fermentation fluid were inoculated with P. digitatum and P. italicum as controls. Three replicates were conducted for each treatment. The plates were then cultured at 28 °C in an incubator for 7 days, the diameter of phytopathogenic colonies were measured using the crossing method and the inhibition rate of the mycelial growth was calculated according to the previously described method [Citation19].
P. digitatum and P. italicum were each inoculated on fresh PDA plates and then incubated at 28 °C until conidia formed. A conidial suspension was prepared by rinsing the plate with 10 mL of sterile water followed by filtration through sterile gauze. A 10-µL aliquot of conidial suspension and 40 µL of sterile fermentation fluid were mixed evenly with 50 µL of potato dextrose broth (PDB) medium. The mixture was deposited onto a sterile glass slide and the slides were placed on U-shaped glass rods in Petri dishes, the bottom of which was covered with a sheet of wet sterilized filter paper. The mixture without fermentation fluid was used as a control. Three replicates were conducted for each treatment. Then, the Petri dishes were incubated at 28 °C in an incubator. After 6 h of incubation, the germination of conidia was observed under a compound light microscope. For each treatment, a total of 400–600 conidia, selected randomly from different microscopic fields, were examined and the conidial germination rate was calculated using the previously described method [Citation19].
Efficacy of fermentation extracts against citrus postharvest disease
The fermentation product of antagonistic strains was centrifuged at 12,000 rpm/min for 10 min and then vacuum-filtered through a sterile filter. The filtrate was collected, mixed with ethyl acetate, and extracted three times. The extraction fractions were combined to obtain the final extraction of the supernatant solution. The actinomycete cell paste was soaked in an 80% acetone aqueous solution overnight and further lysed with ultrasonic shearing. The extractions were combined and concentrated under reduced pressure to remove the acetone. Then, the resulting aqueous phase was extracted using an equal volume of ethyl acetate three times. The extraction fractions from the supernatant solutions and cell pastes were then combined and dried to obtain a whole ethyl acetate extract for the strain. The ethyl acetate extract was dissolved in a small amount of DMSO and then diluted with sterile water to a final concentration of 1500 mg/L.
Fresh citrus fruits of comparable size were used to assess the control efficacy of fermentation extracts against citrus green mould caused by P. digitatum and blue mould caused by P. italicum. Citrus fruits were washed with sterile water to remove surface debris, disinfected with 75% ethanol and then air-dried. Three replicates, each consisting of 24 fruits, were conducted for each treatment. A uniform 3 mm deep and 3 mm in diameter wound was formed in the equatorial region of fruits with a sterile puncher. The four treatments were: (I) antagonist culture extraction, (II) sterile water (negative control), (III) 500 × 25% prochloraz emulsifiable concentrate (positive control) and (IV) 1000 × 40% iminoctadine tris (albesilate) wettable powder (positive control). Thirty microliters 500, 1 000 and 1 500 mg/L of the antagonist fermentation extracts, negative, and positive controls were pipetted into each wound and allowed to remain at room temperature for 24 h. The wounds were then inoculated with 15 µL of 1 × 104 conidia/mL suspension of each of the moulds (P. digitatum and P. italicum). The prochloraz-resistant P. digitatum strain (EC50 = 6.73) was inoculated according to the same method. Three replicates were conducted for each treatment. Fruits were placed in tightly closed plastic containers and incubated at 28 °C in an incubator. At 5 and 10 days post inoculation, the disease severity on the citrus fruits was observed and the disease index and relative control efficacy were calculated according to the previously described methods [Citation20, Citation21].
Morphological, cultural, and physio-biochemical characteristics
Actinomycetes were purified by inoculating aerial mycelia from a single colony over the surface of actinomycete isolation agar. Then a 20-µL aliquot of the spore suspension from purified antagonistic actinomycetes was placed onto Gauze’s medium No. 1 and spread evenly using a sterile triangular spreader. A sterile coverslip was inserted obliquely into the agar plate and then the plates were incubated at 28 °C. At 5, 7, and 10 days after incubation, the cover slips were placed on a slide. Then, the morphological characteristics of mycelia and the spores of the antagonistic strains were examined under an Eclipse E200 light microscope (Nikon Co. Ltd., Tokyo, Japan).
Antagonistic actinomycetes were inoculated on Gauze’s medium No. 1, glycerol-asparagine agar, inorganic salt-starch agar, yeast extract-malt extract agar, oatmeal agar, nutrient agar and glucose-asparagine agar [Citation22]. Three replicates were conducted for each treatment. The plates were then incubated at 28 °C for 7 days. The cultural characteristics of the antagonistic actinomycete strains were observed closely.
The physiological and biochemical properties of the antagonistic actinomycete strain including carbon source utilization, hydrolysis of starch, coagulation and peptization of milk, liquefaction of gelatin, reduction of nitrate, production of H2S, and production of melanin pigment were evaluated according to the previously described method [Citation22].
Molecular analysis
The pure antagonistic actinomycete strain was inoculated into 5 mL of liquid Gauze’s medium No.1 and incubated at 26–28 °C in a shaking incubator for 5–7 days. After incubation, the cultured broth was transferred to a 10-mL tube and centrifuged at 6000 rpm/min for 5 min at room temperature. The cell-free supernatant was discarded, and the cell pellet was dried by removing any remaining liquid. Then, total genomic DNA of the antagonistic strain was extracted according to the previously described method [Citation23].
The 16S rDNA gene of strains was amplified from genomic DNA by polymerase chain reaction (PCR) using the following bacterial universal primers: 27 F (5′-AGAGTTTGATCMTGGCTCAG-3′) and 1492 R (5′-TACGGYTACCTTGTTACGACTT-3′) [Citation24]. The 25-µL PCR reaction mixture contained 5 ng of genomic DNA, 1 × PCR buffer, 1.5 mM MgCl2, 0.2 mM dNTP, 0.6 µM primers and 1 U Taq DNA polymerase. The amplification conditions included an initial denaturation at 94 °C for 5 min, followed by 35 thermocycles each consisting of denaturation at 94 °C for 1 min, annealing at 56 °C for 1 min and extension at 72 °C for 2 min. A final extension at 72 °C for 10 min was also performed. Amplified PCR products were examined by electrophoresis on 1.5% agarose gels pre-stained with GelRed® nucleic acid stain (Biotium, Freemont, CA, USA) and photographed using a gel imaging system.
The target DNA bands were excised from the gel and purified using an AxyPrep™ DNA Gel Extraction Kit (Axygen, Inc., Union City, CA, USA). The purified PCR products were then directly sequenced by Invitrogen (Shanghai Invitrogen Biotechnology Co. Ltd., China). For sequence analyses, the DNA sequences obtained were compared to those deposited in GenBank using BLASTN with default settings. Then, homologous 16S rDNA sequences deposited in GenBank were selected for alignment with the sequence in this study. Finally, multiple sequence alignments were performed using ClustalW and the phylogenetic tree was generated by the neighbor-joining method using MEGA v5.05. Clade stability of the tree was assessed by bootstrap analysis with 1000 replicates.
Statistical analysis
Analysis of variance and multiple comparisons were performed using SAS v9.2 software. The data were expressed as the mean value with standard deviation (±SD). Duncan’s multiple range test was performed at a significance level of P < 0.05.
Results and discussion
Sampling and isolation of marine actinomycetes
Due to long-term growth and propagation in a high-salt, high-pressure, low-temperature and light-free marine environment, marine microorganisms usually have unique metabolic pathways and genetic backgrounds, forming physiological characteristics and characteristics different from those of terrestrial microorganisms. As a result, the isolation and identification of marine microorganisms with novel functions has attracted much attention [Citation25–28].
In this research, 56 isolated pure cultures of actinomycetes were recovered from samples taken from the seashore of Dachen Island, Zhejiang Province, China. The number of isolated actinomycetes varied with different procedures as well as with the use of different isolation media (). Procedure A yielded more actinomycetes, while procedure B resulted in less actinomycetes and greater numbers of other bacteria. Therefore, our results suggest that procedure A is more effective for the isolation of marine actinomycetes. Of the six tested isolation media, M3 medium exhibited the best isolation result and obtained the most actinomycete strains (). We further explored the novel functions of these marine microorganisms.
Screening of antagonistic actinomycetes
To obtain marine microorganisms with control activity against postharvest decay in citrus, P. digitatum – the main postharvest pathogen of citrus, was taken as an indicative fungus to screen antagonistic actinomycetes from the 56 strains using the plate stand-opposite culture method. The results showed that eight strains were able to inhibit the growth of P. digitatum among all the strains and strain AM-4, isolated from the D. granulata, had the largest inhibition zone and exhibited the most potent inhibitory effect against the growth of P. digitatum, with an inhibition zone width of 2.47 ± 0.05 cm ().
Table 3. Inhibition effect of the isolated marine actinomycetes against P. digitatum.
The inhibition spectrum of the strain AM-4 was tested using the plate stand-opposite culture method; this strain indicated a wide inhibition spectrum. It could inhibit mycelial growth of 12 tested phytopathogens to different degrees, especially against P. digitatum, P. italicum, P. mangiferae and C. gloeosporioides, with respective inhibition zone widths of 2.47 ± 0.05, 2.35 ± 0.02, 2.32 ± 0.03 and 2.20 ± 0.02 cm. It exhibited weak inhibition activity against F. oxysporum f. sp. cucumerinum, with an inhibition zone width of 0.65 ± 0.04 cm, indicating that AM-4 strain had a broad inhibition spectrum (). In former reports, the marine actinomycete B5 isolated from the shallow sea also had a similar inhibition spectrum; it had antagonistic effects against 13 plant pathogenic fungi such as F. oxysporum f. sp. niveum [Citation29].
Table 4. Inhibition of the marine actinomycete strain AM-4 against the tested phytopathogens.
Morphological, cultural, physio-biochemical and molecular characteristics
Strain AM-4 could grow on six different media plates with the exception of oatmeal agar (see Materials and methods). White aerial mycelia were formed when grown on five media types except for nutrient agar. Light yellow substrate mycelia were formed when grown on all six agar plates. Grey green to light olive brown soluble pigments were produced when grown on yeast extract-malt extract agar or nutrient agar plates, but not on the other growth media. On Gauze’s medium No. 1, this strain is an aerobic, mesophilic, Gram-positive actinomycete and it grew robustly with white aerial mycelia (). Under a light microscope, its sporotrichial were long chain-shaped and straight. Its spores were oval-shaped with rough surfaces and measured 0.51 × 0.82–1.1 μm ().
Figure 1. Cultural and morphological characteristics of the marine actinomycete strain AM-4. (A) Cultural characteristic of strain AM-4 on Gauze’s medium No.1. (B) Sporotrichial characteristics of strain AM-4 observed through a light microscope.
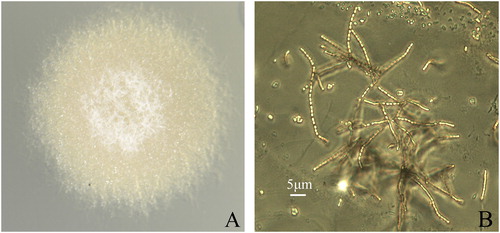
Carbon source utilization was tested using the basal salt medium supplemented with a final concentration of 1% of the tested carbon sources. Among the six carbohydrate substrates that were tested, only glucose could be utilized. Starch hydrolysis was demonstrated with the aid of Lugol's iodine. A clear ring was observed around the colony of strain AM-4, indicating that the strain could produce amylase to hydrolyse starch. Skim milk was used to assess coagulation and peptonisation of milk. Strains AM-4 could coagulate and slowly peptonise milk. Glucose–peptone–gelatin medium was used to examine gelatin liquefaction. Strain AM-4 could not liquefy the gelatin. Nitrate reduction was determined by using a color reaction of diphenylamine and Griess reagents. A red color was observed in the medium after incubation with strain AM-4, indicating that the strain could reduce nitrate. Production of H2S and melanin was determined on Tresner's agar and tyrosine agar respectively. Melanin was not observed on the medium after 7 d culture, indicating that strain AM-4 did not produce H2S and melanin.
The 16S rDNA gene sequence of strain AM-4 showed high similarity to those of multiple species in the genus Streptomyces and exhibited 99.9% similarity to those of Streptomyces chumphonensis strains in GenBank. To further identify the taxa of strain AM-4, its 16S rDNA gene sequence plus the reference sequences were subjected to a phylogenetic analysis. The results showed that strain AM-4 clustered together with the type strain of S. chumphonensis, suggesting that strain AM-4 is most closely related to S. chumphonensis (). Their morphological and physio-biochemical characteristics were also similar [Citation30].
Based on the morphological, cultural and physio-biochemical characteristics and 16S rDNA gene sequence analysis, strain AM-4 was identified as S. chumphonensis, a new species reported by Phongsopitanun et al. [Citation30], which was isolated from marine sediments in Chumphon province, Thailand.
Inhibition activity of the fermentation fluid
The sterile fermentation fluid of the strain AM-4 was prepared to test the inhibitory activity against mycelial growth and conidial germination of citrus postharvest pathogens (P. digitatum and P. italicum). In the beginning, mycelia of the pathogens grew slowly on the PDA plates containing sterile fermentation fluid of strain AM-4, and some wrinkles formed on their colonies. After 7 days of incubation, the diameters of P. digitatum and P. italicum colonies on the PDA plates containing fermentation fluid were only 1.77 ± 0.06 and 2.08 ± 0.06 cm, respectively (). The mycelial growth inhibition rates of strain AM-4 against P. digitatum and P. italicum were 66.23% and 61.42%, respectively. The results demonstrated that strain AM-4 fermentation fluid had inhibition activities against the mycelial growth of P. digitatum and P. italicum.
Table 5. Inhibition activity of the fermentation fluid of the strain AM-4 against P. digitatum and P. italicum.
The conidia of P. digitatum and P. italicum germinated slowly in the PDB media containing the sterile fermentation fluid of strain AM-4. After 6 h of incubation, the germination rates of P. digitatum and P. italicum conidia in the PDB media containing fermentation fluid were only 25.82 ± 2.15 and 29.35 ± 1.34%, respectively, and their germ tubes were short. In contrast, the conidial germination rates of P. digitatum and P. italicum in the control media were relatively higher () and their germ tubes were long and some had grown into mycelia.
Similarly, the metabolite of the marine mould 1-B6-10, isolated from seabed sediments, also had a significant inhibitory effect on the mycelial growth and conidial germination of the plant pathogens F. oxysporum and F. batatasis [Citation31], suggesting that strain AM-4 has a similar inhibition mechanism to strain 1-B6-10.
Efficacy of fermentation extracts against citrus postharvest disease
There are a few studies on the use of marine microorganisms to control plant diseases and they have shown good potential for exploitation and utilization [Citation17, Citation32–34]. In this research, control efficacy of fermentation extracts from the antagonistic strain AM-4 against citrus postharvest decay was further tested and the results are shown in . The relative control efficacy of the gradient-diluted fermentation extracts of strain AM-4 against citrus green mould caused by P. digitatum reached 77.95–92.91% 5 days after inoculation and 56.30–87.22% 10 days after inoculation. The relative control efficacy of the gradient-diluted fermentation extracts against citrus blue mould caused by P. italicum reached 61.32–84.91% 5 days after inoculation and 51.34–80.92% 10 days after inoculation. The fermentation extracts (1000 and 1500 mg/L) of the strain AM-4 had a similar control efficacy as the positive control, i.e. prochloraz (500 × 25% prochloraz) against citrus green mould and blue mould caused by prochloraz sensitive pathogens. But interestingly, the relative control efficacy of the fermentation extracts (1000 and 1500 mg/L) of the strain AM-4 are higher than prochloraz (500 × 25% prochloraz) against citrus green mould caused by prochloraz-resistant P. digitatum strains. While the positive control – iminoctadine tris (albesilate) wettable powder had the control efficacy of 100% against both green and blue moulds. The results demonstrated that iminoctadine tris (albesilate) is the most effective test preparation against green and blue moulds in citrus, while fermentation extracts of the actinomycete strain AM-4 also inhibited good control efficacy against the moulds, especially against the green moulds caused by prochloraz-resistant P. digitatum strains.
Table 6. Control efficacy of fermentation extracts of the marine actinomycete strain AM-4 against citrus green and blue moulds.
Nowadays, prochloraz is widely used on the control of citrus postharvest diseases. However, the long-term and large use of this chemical pesticide not only leaves behind environmental pollution and pesticide residues but also causes pathogenic microorganisms to develop resistance. This study showed that the fermentation extracts of the AM-4 strain exhibited good control efficacy against citrus green mould caused by prochloraz-resistant P. digitatum strains. Therefore, it could be expected that the AM-4 strain may have the potential to reduce the use of prochloraz as an environmentally friendly biological control strain.
Several studies have demonstrated that the mechanisms of actions of microbial antagonists against postharvest fungal pathogens include competition for nutrients and space, antibiosis through antibiotic production, mycoparasitism, production of cell wall lytic enzymes and induction of host resistance [Citation10, Citation35–37]. For example, Serratia plymuthica strain IC1270 inhibits P. digitatum and P. italicum on orange fruit by means of competition for nutrients, while strain IC14 does the same, but by means of interaction with pathogenic cells [Citation38]. Pichia membranefaciens could control citrus postharvest decay caused by P. italicum and P. digitatum through induction of host resistance [Citation39]. The Streptomyces globisporus JK-1 could produce antifungal volatile compounds to control blue mould caused by P. italicum on citrus microcarpa [Citation40]. Bacillus subtilis ABS-S14 and B-912 inhibit the citrus green mould pathogen P. digitatum by producing antibiotics [Citation41, Citation42]. In this study, the sterile fermentation fluid of strain AM-4 could inhibit the mycelial growth and conidial germination of plant pathogens and its crude extracts had a good control efficacy on the postharvest disease of citrus fruits. In addition, strain AM-4 is identified as S. chumphonensis and about 50% of the reported antibiotics produced by microorganisms come from the actinomyces family [Citation43]. Therefore, it was speculated that strain AM-4 might inhibit the plant pathogens by producing antimicrobial substances.
Conclusions
In this study, strain AM-4 was isolated and identified as S. chumphonensis. This strain had strong antagonistic activity against various plant pathogens and its fermentation extracts showed good control efficacy against the main citrus postharvest diseases, especially against the green mould caused by prochloraz-resistant P. digitatum strains. Therefore, strain AM-4 has a good prospect of development and utilization and its application potential in biological control of plant diseases needs to be further explored.
Disclosure statement
No potential conflict of interest was reported by the authors.
Additional information
Funding
References
- Kanetis L, Förster H, Adaskaveg JE. Comparative efficacy of the new postharvest fungicides azoxystrobin, fludioxonil, and pyrimethanil for managing citrus green mold. Plant Dis. 2007;91:1502–1511.
- Zhang J, Timmer LW. Preharvest application of fungicides for postharvest disease control on early season tangerine hybrids in Florida. Crop Prot. 2007;26:886–893.
- Yang S, Peng L, Cheng Y, et al. Control of citrus green and blue molds by Chinese propolis. Food Sci Biotechnol. 2010;19:1303–1308.
- Kinay P, Mansour MF, Gabler FM, et al. Characterization of fungicide-resistant isolates of Penicillium digitatum collected in California. Crop Prot. 2007;26:647–656.
- Sun X, Wang J, Feng D, et al. PdCYP51B, a new putative sterol 14α-demethylase gene of Penicillium digitatum involved in resistance to imazalil and other fungicides inhibiting ergosterol synthesis. Appl Microbiol Biotechnol. 2011;91:1107–1119.
- Wang MS, Sun Y, Chen GQ, et al. Resistance monitoring of Penicillium digitatum from Gannan navel orange to commonly used fungicides. Acta Phytopathol Sin. 2013;43:532–540.
- Jiang LY, Chen GQ, Shi PZ, et al. Efficacy of pyrimethanil in controlling green mould of postharvest citrus. Chin J Pestic Sci. 2010;12:149–154.
- Li H, Ge P, Hao W, et al. Screening and identification of actinomyces MY-5 antagonistic against Penicillium digitatum Sacc. Chin Agric Sci Bull. 2010;26:280–284.
- Guo JH, Qi-Hong TU, Chen CY, et al. Research advances in bio-control of postharvest diseases of citrus with antagonistic microorganisms. Food Sci. 2013;34:351–357.
- Dukare AS, Paul S, Nambi VE, et al. Exploitation of microbial antagonists for the control of postharvest diseases of fruits: a review. Crit Rev Food Sci Nutr. 2018;2:1–16.
- Martin K, Karsten Z. Tapping into microbial diversity. Nat Rev Microbiol. 2004;2:141–150.
- Liu K, Newman M, Mcinroy JA, et al. Selection and assessment of plant growth-promoting rhizobacteria (PGPR) for biological control of multiple plant diseases. Phytopathology. 2017;107:928–936.
- Sponga F, Cavaletti L, Lazzarini A, et al. Biodiversity and potentials of marine-derived microorganisms. J Biotechnol. 1999;70:65–69.
- Li Y, Zheng W, Zheng TL. Advances in research of marine microbial diversity and molecular ecology. Microbiol China. 2013;40:655–668.
- Rocha-Martin J, Harrington C, Dobson AD, et al. Emerging strategies and integrated systems microbiology technologies for biodiscovery of marine bioactive compounds. Mar Drugs. 2014;12:3516–3559.
- Ambavane V, Tokdar P, Parab R, et al. Caerulomycin A—an antifungal compound isolated from marine actinomycetes. AiM. 2014; 04:567–578.
- Nie Y, Liu Y, Li D, et al. Antifungal metabolites from a marine bacterium PY-sw-1 and effects on pathogenic fungi. Acta Phytophy Sin. 2008;35:373–374.
- He H, Ou X, Wang L, et al. Bio-control efficacy of a marine bacterium CIII-1 from mangrove on capsicum bacterial wilt. Acta Phytophy Sin. 2008;35:497–500.
- Li Z, Guo B, Wan K, et al. Effects of bacteria-free filtrate from Bacillus megaterium strain L2 on the mycelium growth and spore germination of Alternaria alternata. Biotechnol Biotecnol Equip. 2015;29:1062–1068.
- Lu L, Du D, Cheng B, et al. Isolation and identification of marine actinomyces A3202 and its control efficacy against postharvest citrus diseases. Acta Phytophy Sin. 2014;41:327–334.
- Kamble SK, Patil BJ. Evaluation of some phytofungicides against Uromyces appendiculatus F. Strauss. Biosci Discov. 2017;8:507–520.
- Ruan JS, Huang Y. Rapid identification and systematics of Actinobacteria. 1st ed. Beijing (China): Science Press; 2011.
- Coombs JT, Franco C. Isolation and identification of actinobacteria from surface-sterilized wheat roots. Appl Environ Microbiol. 2003;69:5603–5608.
- Gupta VK, Shivasharanappa N, Kumar V, et al. Diagnostic evaluation of serological assays and different gene based PCR for detection of Brucella melitensis in goat. Small Rumin Res. 2014;117:94–102.
- Liu Q, Hu J, Xue D, et al. Bio-active substances derived from marine microorganisms. Ying Yong Sheng Tai Xue Bao. 2002;13:901–905.
- Baharum SN, Beng EK, Mokhtar M. Marine microorganisms: potential application and challenges. J Biol Sci. 2010;10:555–564.
- Shang Z, Wang BG. Methods and advances in studies on antimicrobial substances of marine-derived fungi. Chin Bull Life Sci. 2012;24:997–1011.
- Gudiña EJ, Teixeira JA, Rodrigues LR. Biosurfactants produced by marine microorganisms with therapeutic applications. Mar Drugs. 2016;14:38.
- Shao YP, Fang LP, Wei SP, et al. Studies on antagonistic spectrum and stability of actinomycete B5 strain from Marine. Acta Agric Boreali-Occident Sin. 2007;16:248–251.
- Phongsopitanun W, Thawai C, Suwanborirux K, et al. Streptomyces chumphonensis sp. nov., isolated from marine sediments. Int J Syst Evol Microbiol. 2014;64:2605–2610.
- Li SB. Inhibition activity of the metabolite of marine mold 1-B6-10 to Fusarium spp. Acta Phytophy Sin. 2005;32:379–382.
- Hernández-Montiel LG, Ochoa JL, Troyo-Diéguez E, et al. Biocontrol of postharvest blue mold (Penicillium italicum Wehmer) on Mexican lime by marine and citrus Debaryomyces hansenii isolates. Postharvest Biol Technol. 2010;56:181–187.
- Liu M, Wang J, Jiang J, et al. Advances in the studies on the application of marine microorganism in biological pesticides. J Sep Sci. 2014;30:232–236.
- Kong Q. Marine microorganisms as biocontrol agents against fungal phytopathogens and mycotoxins. Biocontrol Sci Technol. 2018;28:77–93.
- Sharma RR, Singh D, Singh R. Biological control of postharvest diseases of fruits and vegetables by microbial antagonists: a review. Biol Control. 2009;50:205–221.
- Liu J, Sui Y, Wisniewski M, et al. Review: Utilization of antagonistic yeasts to manage postharvest fungal diseases of fruit. Int J Food Microbiol. 2013;167:153–160.
- Di F A, Martini C, Mari M. Biological control of postharvest diseases by microbial antagonists: how many mechanisms of action. Eur J Plant Pathol. 2016;145:711–717.
- Meziane H, Gavriel S, Ismailov Z, et al. Control of green and blue mould on orange fruit by Serratia plymuthica strains IC14 and IC1270 and putative modes of action. Postharvest Biol Technol. 2006;39:125–133.
- Luo Y, Zeng K, Ming J. Control of blue and green mold decay of citrus fruit by Pichia membranefaciens and induction of defense responses. Sci Hort. 2012;135:120–127.
- Li Q, Ning P, Zheng L, et al. Fumigant activity of volatiles of Streptomyces globisporus JK-1 against Penicillium italicum on Citrus microcarpa. Postharvest Biol Technol. 2010;58:157–165.
- Waewthongrak W, Pisuchpen S, Leelasuphakul W. Effect of Bacillus subtilis and chitosan applications on green mold (Penicilium digitatum Sacc.) decay in citrus fruit. Postharvest Biol Technol. 2015;99:44–49.
- Fan Q, Tian SP, Li YX, et al. Postharvest biological control of green mold and blue mold of citrus fruits by Bacillus subtilis. Acta Phytopathol Sin. 2000;30:343–348.
- Radhakrishnan M, Suganya S, Balagurunathan R, et al. Preliminary screening for antibacterial and antimycobacterial activity of actinomycetes from less explored ecosystems. World J Microbiol Biotechnol. 2010;26:561–566.