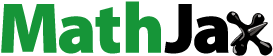
Abstract
This study investigated the effects of hyperoside pre-treatment on the oxidative stress, inflammatory response and apoptosis in rats with cerebral ischemia-reperfusion (I/R) injury. SD rats were randomly divided into sham, I/R, hyperoside (I/R + Hyp) and nimodipine (I/R + Nim) groups. The rats in the hyperoside group and the nimodipine group were intra-gastrically given hyperoside (50 mg/kg/day) and nimodipine (15 mg/kg/day), respectively, 15 days before operation. A rat cerebral ischemia model was established by suture-occlusion 1 h after the last administration. The thread embolus was removed 2 h after the ischemia for the 24 h reperfusion. The results showed that compared with that in the I/R model group, the neurologic function of the hyperoside group rats was significantly improved and the cerebral infarction volume ratio decreased significantly. The total antioxidant capacity, the activity of superoxide dismutase and glutathione peroxidase in the brain tissue of the hyperoside group rats were significantly higher than those in the I/R model group. The content of malondialdehyde, interleukin-1β and tumour necrosis factor-α in the brain tissue of the hyperoside group rats was significantly lower than that in the I/R model group. Compared with that in the I/R model group, the expression of Bcl-2 mRNA, and p-PI3K and p-AKT proteins in the hyperoside group increased significantly, whereas that of Bax and caspase-3 mRNA decreased significantly. These results suggest that hyperoside had a significant protective effect against cerebral I/R injury in rats, which is related to its inhibiting the oxidative stress, alleviating the inflammatory response and having anti-apoptotic effects.
Introduction
Cerebrovascular diseases are common diseases threatening human health, especially among the middle-aged and aging population [Citation1]. Ischemic cerebrovascular diseases account for about 80% of cerebrovascular diseases [Citation2]. The brain is organ of the human body that is the most sensitive to hypoxia, and cerebral ischemia will lead to the damage of local brain tissues and their function, so the timely recovery of blood reperfusion to these tissues is helpful to alleviate the cerebral ischemia injury. However, the recovery of blood flow after ischemia may lead to further damage and dysfunction of tissues in some cases, which is called ischemia-reperfusion (I/R) injury [Citation3]. The prevention and treatment of cerebral I/R injury has become a key link in the treatment of ischemic cerebrovascular diseases [Citation4]. At present, drugs used to prevent and treat cerebral I/R injury often exert their therapeutic effect through a single mechanism and may produce severe side effects at the same time, while traditional Chinese medicines can play a role on many levels and links [Citation5, Citation6]. Therefore, it is of great theoretical and clinical value to study and elucidate the effect and mechanism of action of traditional Chinese medicines used for the prevention and treatment of cerebral I/R injury.
Hyperoside, also known as quercetin-3-O-β-d-galactopyranoside, is found in the fruit or whole plant of Hypericaceae, Rosaceae, Campanulaceae and Celastraceae plants, and belongs to the group of flavonol glycosides, with hepatoprotective, anticancer, bacteriostatic and memory-improving properties [Citation7–9]. In addition, hyperoside can protect against myocardial I/R injury by inhibiting endoplasmic reticulum stress and activating the Nrf2 signalling pathway [Citation10]. Previous studies have found that hyperoside also has inhibits oxidative stress, reduces inflammatory response and apoptosis [Citation11–13], and it is oxidative stress, inflammatory response and apoptosis that are closely related to the occurrence and development of cerebral I/R injury [Citation14, Citation15]. The protective effect of hyperoside against the cerebral I/R injury has been reported, but the underlying mechanism has not been clarified [Citation16, Citation17]. The purpose of this study was to investigate whether hyperoside has a protective effect against cerebral I/R injury in rats and to clarify the underlying mechanism of action to provide a new strategy for the clinical treatment of cerebral ischemia reperfusion injury.
Materials and methods
Materials
Hyperoside was purchased from Chengdu Push Bio-Technology Co., Ltd. (Sichuan, China) and tested by high performance liquid chromatography (HPLC), with a purity ≥98%. 2,3,5-Triphenyltetrazolum chloride (TTC) was purchased from Sigma-Aldrich Company (St. Louis, MO, USA). M-MLV reverse transcription kit was purchased from Takara Biomedical Technology (Beijing) Co., Ltd (Beijing, China). Total antioxidant capacity (T-AOC), superoxide dismutase (SOD), glutathione peroxidase (GSH-Px) and malonaldehyde (MDA) kits were purchased from Nanjing Jiancheng Bioengineering Institute (Jiangsu, China). Interleukin-1β (IL-1β) and tumour necrosis factor-α (TNF-α) kits were purchased from R&D Company (MN, USA). Phosphorylated-phosphatidyl inositol-3-hydroxykinase (p-PI3K), PI3K, phosphorylated-protein kinase B (p-AKT) and AKT polyclonal antibodies were purchased from Santa Cruz Company (CA, USA).
Animal grouping, drug administration and animal model establishment
Healthy male SD rats (n = 64), weighing 180–220 g, were purchased from Jilin University Experimental Animal Center, and raised routinely in a clean class animal room, with food and water ad libitum. SD rats were divided into sham group, I/R model group, hyperoside group (I/R + Hyp) and nimodipine group (I/R + Nim) after they were acclimatized to the laboratory environment for one week; 16 rats in each group. The rats in the hyperoside and nimodipine groups were intra-gastrically given hyperoside (50 mg/kg/day) and nimodipine (15 mg/kg/day), respectively, and those in the sham and I/R groups were intra-gastrically given the same volume of the solvent, once daily for 15 days. The rat cerebral ischemia model was established by suture-occlusion 1 h after the last administration, and 2 h after the ischemia, the thread embolus was removed for the 24 h reperfusion.
Ethics statement
The animal experiments were approved by the Institutional Animal Care and Use Committee of Jilin University. All the experimental procedures were performed in accordance with the Regulations for the Administration of Affairs Concerning Experimental Animals approved by the State Council of the People’s Republic of China.
Neurobehavioral scoring and cerebral infarction volume ratio measurement
After 24 h reperfusion, 8 rats from each group were randomly selected and scored according to the neurobehavioural scoring method proposed by Bederson et al. [Citation18], in which the rats with resistance of their front paws to the lateral pressure and a turn to the left were scored as 3 points, those with resistance but without the turn as 2 points, those that could not fully extend the contralateral front paw as 1 point, and those without symptoms of nervous system injury as 0 points. After the neurobehavioural scoring was finished, the rats were anesthetized with pentobarbital sodium (2%) and decapitated. The brain tissues were removed and placed in a refrigerator at −20 °C for 20 min, and then were successively cut into 2 mm coronal sections. The sections were placed in 2% TTC phosphate buffer solution at 37 °C and in a dark place for 20 min for staining. Then the stained sections were quickly moved to pre-cooled (4 °C) paraformaldehyde solution (4%) and were fixed for 30 min. The fixed sections were photographed and observed, in which the white area was considered as the infarct area and the red tissue as the normal brain tissue. The image was analyzed and the volume ratio of cerebral infarction was calculated using the Image Pro Plus 6.0 image processing software.
Determination of antioxidant activity and inflammatory factor content in brain tissue
Eight rats were randomly selected in each group. The rats were anesthetized with pentobarbital sodium, and then decapitated and their brain tissues were taken. The brain tissue was accurately weighed and cut into pieces with sterile scissors. The cut brain tissue was placed in normal saline precooled to 4 °C in a ratio of 1:9, then was homogenized to take the supernatant, and the supernatant was sub-packed and cryopreserved. According to the procedure in the instruction of the kits, the activity of GSH-Px and the content of MDA in the brain tissue were determined by spectrophotometry (Sigma-Aldrich, St. Louis, MO, USA), and the contents of IL-1β and TNF-α were measured by radioimmunoassay.
Detection of Bcl-2, Bax and caspase-3 mRNA expression in brain tissue
The above remaining brain tissue was homogenized by repeated grinding under low temperature in ice-water baths, and the total RNA was extracted by Trizol method and reverse transcripted to cDNA by using M-MLV reverse transcription kit. The expression levels of apoptosis-related genes Bcl-2, Bax and caspase-3 were detected by the SYBR method, which was completed in a Light Cycler 480 real-time quantitative polymerase chain reaction system (Roche, Basel, Switzerland), and glyceraldehyde-3-phosphate dehydrogenase (GAPDH) was used as the internal reference gene. The relative expression of the target gene was calculated by the 2−ΔΔCt method. The required primers were synthesized by Sagon Biotech (Shanghai) Co., Ltd and the sequences of the primers are shown in .
Table 1. Primers used in real-time qPCR.
Detection of p-PI3K and p-AKT protein expressions in brain tissue
Brain tissue remaining after the above experiments was homogenized by repeated grinding under low temperature in ice-water baths. Coomassie brilliant blue staining was used for the protein quantification. Perpendicular plate sodium dodecyl sulphate polyacrylamide gel electrophoresis (SDS-PAGE) was performed, then the proteins were electro-transferred to polyvinylidene fluoride or polyvinylidene difluoride (PVDF) membranes and the membranes were blocked with skimmed milk powder for 2 h. The membranes were incubated with the first antibodies for 24 h, then washed with PBST 2 times, 10 min each time, and placed in diluted p-PI3K, PI3K, p-AKT and AKT (1:1000 dilution) solutions at 4 °C overnight. After they were washed with phosphate buffered solution Twen-20 (PBST), the membranes were put into the diluted solutions containing the second antibodies labelled by horseradish peroxidase (1:500 dilution), and then incubated at room temperature for 2 h. The membranes were washed with PBST again, then developed with diaminobenzidine (DAB) and photographed by a gel imaging system (Bio-rad, Hercules, CA, USA), and the results were scanned and analyzed by computer.
Statistical analysis
Statistical software SPSS 17.0 was used to analyze the data and the data were expressed as mean values with standard deviation (±SD). The comparison between two groups was conducted by LSD method and one-way analysis of variance was used for the comparison between groups, in which P < 0.05 or P < 0.01 was considered statistically significant.
Results and discussion
Effects of hyperoside on neurobehavioural score and infarct volume ratio in I/R rats
A focal cerebral ischemia animal model is often used in the experimental study of cerebral I/R injury. This model does not need craniotomy, can reduce the intracranial infection, has little effect on the whole body and is a reliable method, with good reproducibility [Citation19]. The rats in the sham group did not show neurological deficits. Compared with that in the sham group, the neurobehavioural score of the rats in the I/R model group was significantly higher (P < 0.01); compared with that in the I/R model group, the neurological score of the rats in hyperoside and nimodipine groups was significantly lower (P < 0.01) (), indicating that hyperoside could improve the neurobehavioural deficits induced by I/R. The TTC staining results showed that the cerebral infarct volume ratio of the rats in the I/R model group was significantly higher than that in the sham group (P < 0.01), and the cerebral infarct volume ratio of the rats in the hyperoside and nimodipine groups was significantly lower than that in the I/R model group (P < 0.05 or P < 0.01) (), suggesting that hyperoside has a significant protective effect against the cerebral I/R injury in rats.
Effects of hyperoside on T-AOC, SOD, GSH-Px activities and MDA content in brain tissue of I/R rats
Oxidative stress is one of the main pathological mechanisms of cerebral I/R injury [Citation20]. The metabolism of brain tissue is vigorous, in which a high oxygen demand is required and a relatively large amount of reactive oxygen species (ROS) is produced, but the brain tissue has basically no oxygen storage and energy storage function, which is completely dependent on the blood supply of oxygen and glucose to provide the energy needed for the activity of brain cells. Moreover, the brain is short of antioxidants and is most vulnerable to free radical injury, so the production of ROS induced by oxidative stress is an important link in the mechanism of cerebral I/R injury [Citation21]. In addition, ROS increase sharply during cerebral I/R, resulting in the activation of caspases and the release of cytochrome C from mitochondria, leading to apoptosis, which exacerbates the brain injury [Citation22]. At the same time, ROS can also be produced in metabolic reaction and central nervous system stress, which can directly participate in the oxidative damage of large molecules such as nucleic acid, lipids and proteins to further lead to the death of cells [Citation23]. Jiang et al. [Citation24] found that ginsenoside CK could lower the level of oxidative stress in the brain tissue of rats with cerebral I/R injury. In this study, the T-AOC, SOD and GSH-Px activities decreased, and the MDA content increased in the brain tissue of the rats in the I/R model group, showing significant differences compared with those in the sham group (P < 0.01). T-AOC, SOD and GSH-Px activities in the brain tissue of the rats in the hyperoside and nimodipine groups were significantly higher than those in the I/R model group, and the content of MDA in the brain tissue of the rats in the hyperoside and nimodipine groups was significantly lower than that in the sham group (P < 0.05 or P < 0.01) (), suggesting that hyperoside could inhibit the oxidative stress in the brain tissue of I/R model rats.
Effects of hyperoside on IL-1β and TNF-α contents in the brain tissue of I/R rats
Inflammatory response is another important mechanism in cerebral I/R injury. Local inflammatory reaction can lead to a series of tissue lesions, causing the overload of free radicals and the activation of toxic enzymes in the brain. In this, various inflammatory mediators that are closely related to each other activate each other, further aggravating the brain damage [Citation25]. Inflammatory cells such as astrocytes can secrete a large amount of IL-1β and IL-1β involved in the process of inflammatory reaction and inflammatory cascade expansion at the early stage of cerebral ischemia [Citation26]. TNF-α, a pro-inflammatory cytokine produced by monocytes and macrophages, can be over-secreted due to the activation of inflammation-related cells in cerebral ischemia, which may stimulate the local inflammatory response of brain tissue to cause vascular injury and thrombosis, aggravating the brain injury [Citation27]. IL-1β and TNF-α do not play an independent role in I/R inflammation, and when IL-β is activated, it will promote the secretion of TNF-α, and then IL-1β and TNF-α interact to trigger the activation of other inflammatory factors, thus further exacerbating the degree of brain injury [Citation28]. As shown in , compared with those in the sham group, the IL-1β and TNF-α contents in the brain tissue of the rats in the I/R model group were significantly increased (P < 0.01); and compared with those in the I/R model group, the IL-1β and TNF-α contents in the brain tissue of the rats in the hyperoside and nimodipine groups were significantly reduced (P < 0.01), indicating that hyperoside could mitigate the response of the brain tissue of rats to the inflammation induced by I/R.
Effects of hyperoside on the expression of Bcl-2, Bax and caspase-3 mRNA in the brain tissue of I/R rats
Apoptosis is also an important mechanism associated with cerebral I/R injury. In cerebral ischemia, the neuronal death around the ischemic central area is dominated by apoptosis [Citation29]. Caspase family members are the key proteins that dominate the apoptosis pathway. They can be activated through both a mitochondrial dependent pathway and a non-mitochondrial dependent pathway, leading to a cascade of apoptosis, of which caspase-3 is the gold index used to detect apoptosis [Citation30]. Bcl-2 family members also play an important role in the regulation of apoptosis. Bcl-2 family is divided into anti-apoptotic proteins and pro-apoptotic proteins. These two groups of proteins maintain a dynamic balance when the body is in a normal physiological state, but when the cells are in the stress state of cerebral ischemia, the expression of anti-apoptotic protein Bcl-2 decreases, while the expression of pro-apoptotic protein Bax increases [Citation31]. As shown in , compared with that in the sham group, the expression of Bcl-2 mRNA decreased, while that of Bax and caspase-3 mRNA increased significantly in the brain tissue of the rats in the I/R model group (P < 0.01); and the expression of Bcl-2 mRNA in the brain tissue of the rats in the hyperoside and nimodipine groups was significantly higher than that in the I/R model group (P < 0.05 or P < 0.01), and the expression of Bax and caspase-3 mRNA was significantly lower than that in the I/R model group (P < 0.01), indicating that hyperoside should have an anti-apoptotic effect in the brain tissue of the I/R model rats.
Effects of hyperoside on the expression of p-PI3K and p-AKT proteins in the brain of I/R rats
It has been shown that the PI3K/AKT signal transduction pathway is involved in the neuronal apoptosis in cerebral ischemia [Citation32]. AKT can not only promote the phosphorylation of apoptotic proteins such as caspase-3 to inactivate them, but also promote the transcription and expression of anti-apoptotic genes such as Bcl-2 to make the cells survive [Citation33]. As shown in , compared with that in the sham group, the expression of p-PI3K and p-AKT proteins in the brain tissue of the rats in the I/R model group decreased significantly (P < 0.01), and compared with that in the I/R model group, the expression of p-PI3K and p-AKT proteins increased significantly in the hyperoside and nimodipine groups (P < 0.01), indicating that the protective effect of hyperoside against the cerebral I/R injury in rats is related to the anti-apoptotic effect induced by the activation of the PI3K/AKT pathway.
Figure 5. Effects of hyperoside on the expression of p-PI3K and p-AKT proteins in the brain tissue of I/R rats ( ± SD, n = 8). p-PI3K protein level (A); p-AKT protein level (B). **P < 0.01, vs the sham group; ##P < 0.01, vs I/R group.
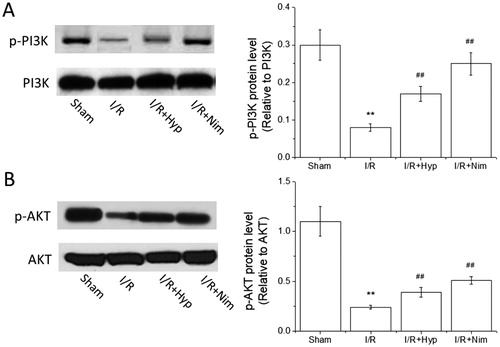
More and more attention has been paid to anti-oxidative stress, alleviation of inflammation and anti-apoptosis in the study on activities of hyperoside. Hyperoside could alleviate the oxidative stress injury induced by H2O2 in endothelial cells in human umbilical vein [Citation34]. Piao et al. [Citation35] found that hyperoside could increase the activity of CAT and GSH-Px to eliminate the ROS in endothelial cells in human umbilical vein, which might protect from the formation of DNA fragments in the cells, thus protecting lung fibroblasts against the oxidative stress induced by H2O2. Hyperoside has not only the effect of anti-oxidative stress, but also the effect of alleviating inflammation. It has been reported [Citation36] that hyperoside could inhibit the high blood sugar-induced inflammation of endothelial cells in human veins and mouse blood vessels, suggesting that hyperoside should be a potential effective drug for the treatment of diabetes and hypertension. According to Kim et al. [Citation37], hyperoside could inhibit the activation of NF-κB to produce an anti-inflammatory effect, and hyperoside also had an anti-apoptosis effect. Zhang et al. [Citation38] found that hyperoside could reduce the apoptosis induced by aspartate in a dose-dependent manner. Hyperoside could also reduce the apoptosis of neurons by activating the PI3K/AKT pathway to regulate the proportion of Bad/Bcl in neurons of newborn rats [Citation39]. It was also indicated in this study that the anti-oxidative stress, alleviation of inflammation and anti-apoptosis of hyperoside should be the main mechanisms through which hyperoside could play a protective role in the model of cerebral I/R injury in rats. However, the in-depth mechanism needs to be further studied.
Conclusions
Hyperoside had a significant protective effect against the cerebral I/R injury in rats. The protective effect of hyperoside against the cerebral I/R injury was related to the inhibition of oxidative stress, alleviation of inflammatory response and inhibition of apoptosis.
Disclosure statement
No potential conflict of interest was reported by the authors.
Additional information
Funding
References
- Donahue MJ, Hendrikse J. Improved detection of cerebrovascular disease processes: introduction to the Journal of Cerebral Blood Flow and Metabolism special issue on cerebrovascular disease. J Cereb Blood Flow Metab. 2018;38:1387–1390.
- Zhang J, Wang G, Zhang F, et al. Improvement of postoperative cognitive dysfunction and attention network function of patients with ischemic cerebrovascular disease via dexmedetomidine. Exp Ther Med. 2018;15:2968–2972.
- Che N, Ma Y, Xin Y. Protective role of fucoidan in cerebral ischemia-reperfusion injury through inhibition of MAPK signaling pathway. Biomol Ther. 2017;25:272–278.
- Feng J, Chen X, Guan B, et al. Inhibition of peroxynitrite-induced mitophagy activation attenuates cerebral ischemia-reperfusion injury. Mol Neurobiol. 2018;55:6369–6386.
- Lv X, Zhang Y, Zhang H. Neuroprotective effect of Trichosanthes kirilowii cassia twig on cerebral ischemia-reperfusion injury in rats. Pak J Pharm Sci. 2018;31:1643–1647.
- Cheng X, Hou Z, Sun J, et al. Protective effects of Tongxinluo on cerebral ischemia/reperfusion injury related to Connexin 43/Calpain II/Bax/Caspase-3 pathway in rat. J Ethnopharmacol. 2017;198:148–157.
- Zhang Y, Wang M, Dong H, et al. Anti-hypoglycemic and hepatocyte-protective effects of hyperoside from Zanthoxylum bungeanum leaves in mice with high-carbohydrate/high-fat diet and alloxan-induced diabetes. Int J Mol Med. 2018;41:77–86.
- Zhang Y, Dong H, Zhang J, et al. Inhibitory effect of hyperoside isolated from Zanthoxylum bungeanum leaves on SW620 human colorectal cancer cells via induction of the p53 signaling pathway and apoptosis. Mol Med Rep. 2017;16:1125–1132.
- Sun Y, Sun F, Feng W, et al. Hyperoside inhibits biofilm formation of Pseudomonas aeruginosa. Exp Ther Med. 2017;14:1647–1652.
- Hou JY, Liu Y, Liu L, et al. Protective effect of hyperoside on cardiac ischemia reperfusion injury through inhibition of ER stress and activation of Nrf2 signaling. Asian Pac J Trop Med. 2016;9:76–80.
- Chen Y, Ye L, Li W, et al. Hyperoside protects human kidney-2 cells against oxidative damage induced by oxalic acid. Mol Med Rep. 2018;18:486–494.
- Fan HH, Zhu LB, Li T, et al. Hyperoside inhibits lipopolysaccharide-induced inflammatory responses in microglial cells via p38 and NFκB pathways. Int Immunopharmacol. 2017;50:14–21.
- Zhou YQ, Zhao YT, Zhao XY, et al. Hyperoside suppresses lipopolysaccharide-induced inflammation and apoptosis in human umbilical vein endothelial cells. Curr Med Sci. 2018;38:222–228.
- Godinho J, de Sa-Nakanishi AB, Moreira LS, et al. Ethyl-acetate fraction of Trichilia catigua protects against oxidative stress and neuroinflammation after cerebral ischemia/reperfusion. J Ethnopharmacol. 2018;221:109–118.
- Zeng G, Ding W, Li Y, et al. Morroniside protects against cerebral ischemia/reperfusion injury by inhibiting neuron apoptosis and MMP2/9 expression. Exp Ther Med. 2018;16:2229–2234.
- Chen Z, Ma C, Zhao W. Protective effect of hyperin against cerebral ischemia-reperfusion injury. Yao Xue Xue Bao. 1998;33:14–17.
- Chen HY, Wang JH, Ren ZX, et al. Protective effect of hyperin on focal cerebral ischemia reperfusion injury in rats. J Chin Integr Med. 2006;4:526–529.
- Bederson JB, Pitts LH, Tsuji M, et al. Rat middle cerebral artery occlusion: evaluation of the model and development of a neurologic examination. Stroke. 1986;17:472–476.
- Longa EZ, Weinstein PR, Carlson S, et al. Reversible middle cerebral artery occlusion without craniectomy in rats. Stroke. 1989;20:84–91.
- Yu W, Gao D, Jin W, et al. Propofol prevents oxidative stress by decreasing the ischemic accumulation of succinate in focal cerebral ischemia-reperfusion injury. Neurochem Res. 2018;43:420–429.
- Li X, Guo H, Zhao L, et al. Adiponectin attenuates NADPH oxidase-mediated oxidative stress and neuronal damage induced by cerebral ischemia-reperfusion injury. Biochim Biophys Acta. 2017;1863:3265–3276.
- Li W, Tan C, Liu Y, et al. Resveratrol ameliorates oxidative stress and inhibits aquaporin 4 expression following rat cerebral ischemia-reperfusion injury. Mol Med Rep. 2015;12:7756–7762.
- Zhang C, Shen M, Teng F, et al. Ultrasound-enhanced protective effect of tetramethylpyrazine via the ROS/HIF-1A signaling pathway in an in vitro cerebral ischemia/reperfusion injury model. Ultrasound Med Biol. 2018;44:1786–1798.
- Jiang S, Zhang H, Qian M, et al. Effects of ginsenoside CK pretreatment on oxidative stress and inflammation in rats with cerebral ischemia/reperfusion injury. Biotechnol Biotechnol Equip. 2018;32:1606–1612.
- Gao XJ, Xie GN, Liu L, et al. Sesamol attenuates oxidative stress, apoptosis and inflammation in focal cerebral ischemia/reperfusion injury. Exp Ther Med. 2017;14:841–847.
- Wang L, Liu H, Zhang L, et al. Neuroprotection of dexmedetomidine against cerebral ischemia-reperfusion injury in rats: involved in inhibition of NF-κB and inflammation response. Biomol Ther. 2017;25:383–389.
- Wang X, An F, Wang S, et al. Orientin attenuates cerebral ischemia/reperfusion injury in rat model through the AQP-4 and TLR4/NF-κB/TNF-α signaling pathway. J Stroke Cerebrovasc Dis. 2017;26:2199–2214.
- Saito K, Suyama K, Nishida K, et al. Early increases in TNF-alpha, IL-6 and IL-1 beta levels following transient cerebral ischemia in gerbil brain. Neurosci Lett. 1996;206:149–152.
- Huang X, Ding J, Li Y, et al. Exosomes derived from PEDF modified adipose-derived mesenchymal stem cells ameliorate cerebral ischemia-reperfusion injury by regulation of autophagy and apoptosis. Exp Cell Res. 2018;371:269–277.
- Juraver-Geslin HA, Durand BC. Early development of the neural plate: new roles for apoptosis and for one of its main effectors caspase-3. Genesis. 2015;53:203–224.
- Gupta R, Ghosh S. Putative roles of mitochondrial voltage-dependent anion channel, Bcl-2 family proteins and c-Jun N-terminal kinases in ischemic stroke associated apoptosis. Biochim Open. 2017;4:47–55.
- Chang Y, Huang W, Sun Q, et al. MicroRNA-634 alters nerve apoptosis via the PI3K/Akt pathway in cerebral infarction. Int J Mol Med. 2018;42:2145–2154.
- Zhang H, Gao J, Wang M, et al. Effects of scalp electroacupuncture on the PI3K/Akt signalling pathway and apoptosis of hippocampal neurons in a rat model of cerebral palsy. Acupunct Med. 2018;36:96–102.
- Li HB, Yi X, Gao JM, et al. The mechanism of hyperoside protection of ECV-304 cells against tert-butyl hydroperoxide-induced injury. Pharmacology. 2008;82:105–113.
- Piao MJ, Kang KA, Zhang R, et al. Hyperoside prevents oxidative damage induced by hydrogen peroxide in lung fibroblast cells via an antioxidant effect. Biochim Biophys Acta. 2008;1780:1448–1457.
- Ku SK, Kwak S, Kwon OJ, et al. Hyperoside inhibits high-glucose-induced vascular inflammation in vitro and in vivo. Inflammation. 2014;37:1389–1400.
- Kim SJ, Um JY, Lee JY. Anti-inflammatory activity of hyperoside through the suppression of nuclear factor-κB activation in mouse peritoneal macrophages . Am J Chin Med. 2011;39:171–181.
- Zhang XN, Li JM, Yang Q, et al. Anti-apoptotic effects of hyperoside via inhibition of NR2B-containing NMDA receptors. Pharmacol Rep. 2010;62:949–955.
- Zeng KW, Wang XM, Ko H, et al. Hyperoside protects primary rat cortical neurons from neurotoxicity induced by amyloid beta-protein via the PI3K/Akt/Bad/Bcl(XL)-regulated mitochondrial apoptotic pathway. Eur J Pharmacol. 2011;672:45–55.