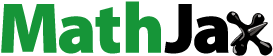
Abstract
The aim of this study was to investigate the role of chromatin assembly factor 1 subunit A (CHAF1A) in the pathogenesis of non-small cell lung cancer (NSCLC), as well as its association with disease prognosis. The expression levels of CHAF1A in NSCLC tissues were detected by immunohistochemical staining. The relationship between CHAF1A and patient survival was analysed. The effects of CHAF1A knock-down on cell proliferation, cell cycle, cell clones and cellular apoptosis were also analysed. The results showed that CHAF1A staining was observed in the cell nuclei in the NSCLC tissue, with higher H-score. In the CHAF1A low-expression group, the 5-year survival rate was significantly higher and the recurrence rate was significantly lower compared with the high-expression group. Tumour size, lymph node metastasis, clinical staging and H-score represented influencing factors related to the 5-year survival. CHAF1A expression was significantly associated with the 5-year overall survival and metastasis and recurrence of NSCLC. In addition, CHAF1A knock-down inhibited the proliferation and changed the cell cycles, cell clones and cellular apoptosis in the lung cancer cells. The results indicated that higher CHAF1A expression is related to shortened survival period of NSCLC, and CHAF1A knock-down inhibited the proliferation of lung cancer cell lines. CHAF1A might represent a disease prognostic predictor in clinic.
Introduction
Lung cancer is the leading cause of death among all cancers worldwide, which severely threatens people’s health and brings heavy medical burden. In 2017, there were 1.8 million new cases of lung cancer throughout the world, accounting for 13% of the new cancer cases. Lung cancer represents the primary cause of cancer-induced death in males [Citation1]. Due to the high malignancy and the high recurrence and metastasis rates, as well as the late diagnosis, the 5-year survival rate of lung cancer is only 18% [Citation2].
Lung cancer cases can be divided into small cell lung cancers and non-small cell lung cancers (NSCLC), the NSCLC accounting for 80% of the primary lung cancer cases. In recent years, with the rapid development of high-throughput sequencing technology, the discovery of molecular targets for the epidermal growth factor receptor (EGFR) and anaplastic lymphoma kinase (ALK) and the development of corresponding target drugs have expanded the traditional treatment strategies of lung cancer, exhibiting satisfactory therapeutic effects in the treatment of lung adenocarcinoma [Citation3,Citation4]. However, the high heterogeneity of lung cancer and the complex epigenetic background make individual treatments very difficult. Therefore, it is of great importance to find new therapeutic targets and discover the prognostic markers for lung cancer to achieve individual treatment.
Chromatin assembly factor 1 (CAF-1) is a heterotrimeric complex consisting of p150, p60 and p48 subunits [Citation5]. During DNA synthesis, chromatin assembly factor 1 subunit A (CHAF1A) interacts with proliferating cell nuclear antigen (PCNA), recruiting histones to the replication fork to form new nucleosomes and high-level chromatin structure [Citation6]. In addition to the DNA synthesis, CHAF1A also participates in the PCNA-independent DNA damage repair process [Citation7]. In recent years, a number of studies have shown that the histone chaperone CHAF1A is closely related to cell proliferation, and the out-of-control and rapid cell proliferation represents important mechanisms of tumour malignancy [Citation8]. CHAF1A plays important roles in the pathogenesis and development of malignant tumours, such as breast cancer [Citation9], colon cancer [Citation10], liver cancer [Citation11] and gliomas [Citation12]. Based on these findings, CHAF1A may represent an oncogene for malignant tumours. However, it is still unclear whether CHAF1A is involved in the pathogenesis of NSCLC.
In this study, the potential roles of CHAF1A in the pathogenesis and development of NSCLC were investigated. The expression levels of CHAF1A were detected in 123 cases of NSCLC, and its association with the disease prognosis was also analysed.
Subjects and methods
Subjects and clinical samples
The formalin-fixed and paraffin-embedded tissues from a total of 123 cases of NSCLC were included in this study. These patients underwent surgical resection in the Shanxi Tumor Hospital, from January 2010 to June 2011. These patients had received no radiotherapy and chemotherapy before surgery. Lung cancer staging was performed according to the TNM staging criteria from the seventh edition of AJCC/UICC in 2009 [Citation13]. These patients were followed up by telephone in June 2016. Clinical and pathological data are shown in .
Table 1. Clinical and pathological data.
Ethics statement
All patients signed informed consent forms and the study was approved by Shanxi Tumor Hospital.
Cell line
Lung cancer cell line H1299 was purchased from Shanghai Cell Bank (Shanghai, China). These cells were incubated with 1640 medium, supplemented with 10% fetal bovine serum and penicillin/streptomycin, in a 5% CO2, 37 °C incubator.
Immunohistochemical staining
The tissues were cut into 4-μm sections, and stained according to the routine immunohistochemical staining procedures. Rabbit anti-CHAF1A antibody (ab126625; 1:200 dilution; Abcam, Cambridge, UK) were used for staining. The sections were analysed with the H-score integral semi-quantitative analysis with the Scanscope digital pathological scanning system (Aperio, Vista, CA, USA). H-score integral was determined by the staining intensity multiplied by the percentage of positive cells (0–300). Staining intensity was defined as follows: 0, negative; 1, weak positive; 2, positive; and 3, strong positive. The positively stained cells were counted and averaged from 5 randomly selected visual fields with ×100 magnification in each section, under a Leica Microsystems (Leica Microsystems GmbH, Wetzlar, Germany).
Quantitative teal-time polymerase chain reaction (RT qPCR)
Total RNA was extracted using Trizol. The cDNA was synthesized by reverse transcription using the Promega M-MLV kit. Quantitative real-time PCR was performed on a PCR system (Applied Biosystems, Foster City, CA, USA) using the following primer sequences: CHAF1A, forward 5′-AGGGAAGGTGCCTATGGTG-3′ and reverse 5′-CAGGGACGAATGGCTGAGTA-3; and GAPDH, forward 5′-TGACTTCAACAGCGACACCCA-3′ and reverse 5′-CACCCTGTTGCTGTAGCCAAA-3′. The expression of target genes was calculated using the 2-ΔΔCt method. The glyceraldehyde 3-phosphate dehydrogenase (GAPDH) gene was used as the internal reference.
Cell transfection of siRNA
The siRNA sequence of CHAF1A was designed based on the BLOCK-iTTM RNAi Designer website. The siRNA sequence targeting on CHAF1A was 5′-GACTCAATTCCTGTGTAAA-3′. Negative Control sequence of TTCTCCGAACGTGTCACGT was used. The plasmid was constructed -and lentivirus was packaged. In total, 3–5 × 104 cells from the logarithmic growth phase were harvested and suspended. Transfection was performed with the Lipofectamine 2000. After 72 h, the expression of the reporter gene was observed by fluorescence microscopy, and the infection rate was determined. The specificity of the designed sequences were demonstrated by the knock-down efficiency.
Cell proliferation detection
Cells were planted onto culture plates at a density of 2000 cells/well. Starting from the second day, the Cellomis test was performed once daily on each plate, for 1–5 continuous days. A cell proliferation curve for 1–5 days was plotted. After the cell transfection, cells were planted onto a 6-well plate, at a density of 400–1000 cells/well. Two weeks later, the cells were fixed, stained and counted. The cell proliferation ability was assessed by determining the cloning rate. Then the cells were harvested, and stained with PI and RNaseA. Cell cycle stages and apoptosis were detected by flow cytometry on a BD FACSCalibur instrument (BD Biosciences, Franklin Lakes, NJ, USA). Experiments were performed in triplicates.
Statistical analysis
Data were expressed as mean values with standard deviation (SD). SPSS software was used for statistical analysis. The t-test was performed for group comparison. The H-scoring of the tumour and surrounding tissue was performed with the paired t-test. The log-rank test and COX model analysis were used for survival analysis. Kaplan-Meier survival curves were plotted. P < 0.05 was considered statistically significant.
Results and discussion
Over-expression of CHAF1A in NSCLC tissues
The expression levels of CHAF1A were first investigated by immunohistochemical staining in 123 cases of NSCLC tissue and 123 cases of normal lung tissue. Our results showed that CHAF1A was predominantly stained positive in the nuclei of the NSCLC tissue, while CHAF1A staining was rare or barely detectable in normal control tissues (). Moreover, the H-score integral for CHAF1A was significantly higher in the lung cancer tissue than the normal lung tissue (P < 0.05; ). These results indicate that CHAF1A is over-expressed in the NSCLC tissues.
Over-expression of CHAF1A correlates with poor prognosis of NSCLC
The association between CHAF1A expression and NSCLC prognosis was then investigated. The cases were divided into two groups: CHAF1A high- and low-expression according to the 10th percentile of the H-score. Log-rank test and COX model analysis were performed to assess the relationship between CHAF1A expression and patient survival, and the survival curve was plotted. Our results showed that the 5-year survival rate for the CHAF1A low-expression group was 81.8%, significantly higher than that for the CHAF1A high-expression group (54.5%) (P < 0.05). Moreover, the recurrence rate was significantly higher (P < 0.01)in the high-expression group (59.4%) than in the low-expression group (18.2%).
The Kaplan-Meier curves based on the CHAF1A expression levels in these NSCLC patients are shown in . Analysis of the association between the clinicopathological parameters and the 5-year survival in these NSCLC patients showed that the tumour size, lymph node metastasis, clinical staging and H-score represented influencing factors related to the 5-year survival. Moreover, gender, pathological type, differentiation degree, tumour size, lymph node metastasis, clinical staging and H-score represented influencing factors for metastasis and recurrence. The results from the log-rank test for the disease-free survival and overall survival of the patients are shown in . Excluding survival-independent covariates, COX regression analysis showed that the risk of metastasis and recurrence within 5 years for the CHAF1A high-expression group was 3.458-fold higher than that for the low-expression group (95% CI, 1.447–8.265). Moreover, the risk of death within 5 years for the CHAF1A high-expression group was 3.059-fold higher than that for the low-expression group (95% CI, 1.085–8.622). The results from the COX regression analysis are shown in . Taken together, these results suggest that the expression of CHAF1A was significantly associated with the 5-year overall survival and metastasis and recurrence of NSCLC, indicating that CHAF1A might represent an independent predictor for poor NSCLC prognosis.
Figure 2. Over-expression of CHAF1A correlates with poor prognosis of NSCLC. Kaplan–Meier curves for the high-expression (A) and low-expression (B) groups.
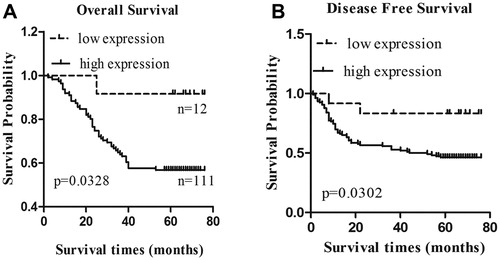
Table 2. Log-rank test of disease-free survival and total survival of NSCLC patients.
Table 3. COX regression analysis.
CHAF1A knock-down inhibits proliferation of lung cancer cells
The H1299 cells were transfected with the lentivirus containing siRNA sequence for CHAF1A. Compared with the control group, the mRNA expression of CHAF1A was significantly inhibited in the H1299 cells transfected with CHAF1A siRNA lentivirus, with an inhibiting rate of 85% (). Moreover, at 1–5 days after transfection, compared with the control group, the cell proliferation was significantly inhibited, suggesting that CHAF1A knock-down could inhibit the proliferation of lung cancer cells (). Furthermore, at day 5 after transfection, in the CHAF1A-siRNA group, the number of cells in the G1 phase was significantly reduced (35.51 ± 1.77 vs. 40.72 ± 0.2%; P < 0.05), whereas that of S-phase cells was significantly increased (63.57 ± 1.9 vs. 52.7 ± 0.89%; P < 0.05), with no significant changes in the number of G2/M-phase cells (P > 0.05) (). In addition, the results from the cell clone counting showed that, compared with the control group, the H1299 clone number in the CHAF1A-siRNA group was significantly declined (P < 0.05) (). Cell colony formation is shown in . The flow cytometry results showed that the apoptotic rate was significantly increased in the CHAF1A-siRNA group compared with the control group (8.42 ± 0.07 vs. 4.54 ± 0.2%; P < 0.001) (). Taken together, these results suggest that CHAF1A knock-down can inhibit the proliferation of H1299 cells, reduce clone formation, induce cell cycle arrest in S phase, and increase cellular apoptosis. Therefore, CHAF1A can promote the growth and proliferation of H1299 cells.
Figure 3. CHAF1A knock-down inhibits proliferation of lung cancer cells. (A) Knock-down efficiency detected by quantitative real-time PCR analysis. Compared with the control group, ** P < 0.01. (B) Cell proliferation assessed at 1–5 days in H1299 cells transfected with CHAF1A-siRNA lentivirus. (C) H1299 cell counts at G1, S and G2/M cell cycle phases detected by flow cytometry at day 5 after CHAF1A-siRNA transfection. (D) Cell clone counts at days 1–3 after CHAF1A-siRNA transfection. (E) Cell colony formation. (F) Apoptotic rate. Compared with the control group, *** P < 0.001.
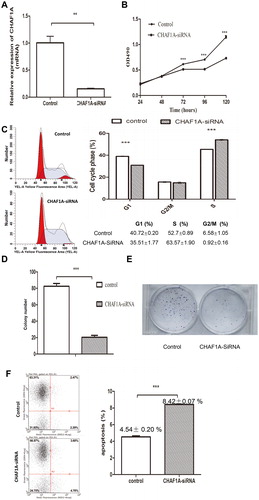
CAF-1 is involved in the DNA replication and damage repair processes, and in gene expression regulation. An important mechanism for malignant tumour pathogenesis and development is the out-of-control and rapid cell proliferation [Citation14]. Studies have reported the over-expression of cell proliferation-regulating genes in many types of tumours [Citation15], as well as in mitotic division accompanied by DNA replication. However, the primary function of CHAF1A is to deliver the newly synthesized H3/H4 dimer to the replication fork during the S phase, and CHAF1A is involved in the formation of nucleosomes and DNA replication. Moreover, CHAF1A has also been closely associated with Ki-67 and proliferating cell nuclear antigen, indicating that it might enhance cell proliferation. Polo et al. [Citation9] have shown that CHAF1A is highly expressed in some proliferating breast cancer cell lines and human breast cancer tissues, whereas it is significantly down-regulated in the quiescent cells. These findings suggest that CHAF1A could serve as a marker for the identification of proliferating and quiescent cells.
Studies concerning cell cycles have shown that CAF-1 is highly expressed in the S phase, moderately expressed in the G1 and G2 phases, indicating that CHAF1A is involved in the DNA repair process. CHAF1A has been shown to protect the newly synthesized DNA from over-degradation during DNA mismatch repair, and participate in nucleotide excision repair and homologous recombination repair [Citation7]. Moreover, CHAF1A dysfunction affects the cell cycle progression and DNA repair, which might lead to the occurrence of tongue cancer [Citation15].
CHAF1A can promote the trimethylation of lysine in the H3 histone, and the change in methylation pattern could lead to silencing of abnormal cancer genes, inhibiting expression of tumour suppressor genes. At the same time, H3K9 histone methyltransferase interacts with DNA methyltransferase to regulate the gene silencing process, which further enhances the interaction between DNA methylation and histone modification to regulate the epigenetic expression of genes [Citation16]. Studies have shown that the expression level of H3K9me3 is reduced after CHAF1A knockdown in the neuroblastoma cell lines, which thereby reduces the H3K9 trimethylation of the target genes associated with cell proliferation, differentiation and survival, further promoting the differentiation of neurons in vivo and in vitro [Citation17]. Based on these findings, CHAF1A could promote the proliferation of tumour cells, affect the DNA repair process, and regulate the epigenetic regulation, to promote the development of malignant tumours. Meanwhile, studies concerning the relationship between CHAF1A and clinical pathology have shown that CHAF1A represents a predictor of poor prognosis of breast cancer [Citation9], colon cancer [Citation10], glioblastomas [Citation12], tongue squamous cell carcinoma [Citation15] and gastric cancer [Citation18]. Based on the study with expanded NSCLC case numbers by Liu et al. [Citation19], the high protein expression of CHAF1A was verified in these NSCLC cases. Higher CHAF1A expression was related to the shortened overall survival and disease-free survival periods of NSCLC. These findings suggest that CHAF1A could represent the prognostic predictor for NSCLC. On the other hand, according to the abundance of CHAF1A mRNA in the lung cancer cell line in the study by Liu et al. [Citation19], functional investigation was performed on the H1299 lung cancer cell line. CHAF1A knock-down can inhibit the proliferation of lung cancer cell lines, induce cellular apoptosis and reduce cell clone formation. However, in the cell cycle experiment, to avoid the poor growth status of cells in the absence of serum, 5% fetal bovine serum was added to the culture solution herein, which may have led to the observed differences in the number of cells that entered S-phase when compared with the Liu et al. [Citation19] study. The mechanism underlying the proliferation-inducing effects of CHAF1A on the H1299 cells is still unknown, which might be related to miR-520b. Down-regulated expression of miR-520b in the NSCLC tissues might play an inhibitory role for CHAF1A [Citation20].
Conclusions
The results from this study suggest that CHAF1A might play an important role in the pathogenesis and development of NSCLC. Higher CHAF1A expression was related to a shorter survival period, and CHAF1A knock-down inhibited the proliferation of lung cancer cell lines. CHAF1A might represent a disease prognostic predictor in clinic.
Disclosure statement
No potential conflict of interest was reported by the authors.
Funding
This work was supported by the Shanxi Provincial Science and Technology Development Project (No. 20150313007-2).
References
- Siegel RL, Miller KD, Jemal A. Cancer statistics, 2017. CA Cancer J Clin. 2017;67:7–30.
- Ettinger DS, Akerley W, Bepler G, et al. Non-small cell lung cancer. J Natl Compr Canc Netw. 2010;8:740–801.
- Soda M, Choi YL, Enomoto M, et al. Identification of the transforming EML4-ALK fusion gene in non-small-cell lung cancer. Nature. 2007;448:561–566.
- Pao W, Miller V, Zakowski M, et al. EGF receptor gene mutations are common in lung cancers from "never smokers" and are associated with sensitivity of tumors to gefitinib and erlotinib. Proc Natl Acad Sci USA.. 2004;101:13306–13311.
- Smith S, Stillman B. Purification and characterization of CAF-I, a human cell factor required for chromatin assembly during DNA replication in vitro. Cell. 1989;58:15–25.
- Osley MA. The regulation of histone synthesis in the cell cycle. Annu Rev Biochem.. 1991;60:827–861.
- Mjelle R, Hegre SA, Aas PA, et al. Cell cycle regulation of human DNA repair and chromatin remodeling genes. DNA Repair (Amst). 2015;30:53–67.
- Hanahan D, Weinberg RA. The hallmarks of cancer. Cell. 2000;100:57–70.
- Polo SE, Theocharis SE, Klijanienko J, et al. Chromatin assembly factor-1, a marker of clinical value to distinguish quiescent from proliferating cells. Cancer Res.. 2004;64:2371–2381.
- Wu Z, Cui F, Yu F, et al. Up-regulation of CHAF1A, a poor prognostic factor, facilitates cell proliferation of colon cancer. Biochem Biophys Res Commun. 2014;449:208–215.
- Xu M, Jia Y, Liu Z, et al. Chromatin assembly factor 1, subunit A (P150) facilitates cell proliferation in human hepatocellular carcinoma. OncoTarget Ther. 2016;9:4023–4035.
- Peng H, Du B, Jiang H, et al. Over-expression of CHAF1A promotes cell proliferation and apoptosis resistance in glioblastoma cells via AKT/FOXO3a/Bim pathway. Biochem Biophys Res Commun.. 2016;469:1111–1116.
- Leslie HS, Mary KG, Christian W. TNM classification of malignant tumours-7th edition. Cancer. 1997;80:1803–1804.
- Whitfield ML, George LK, Grant GD, et al. Common markers of proliferation. Nat Rev Cancer. 2006;6:99–106.
- Staibano S, Mignogna C, Lo Muzio L, et al. Chromatin assembly factor-1 (CAF-1)-mediated regulation of cell proliferation and DNA repair: a link with the biological behaviour of squamous cell carcinoma of the tongue?. Histopathology. 2007;50:911–919.
- Poleshko A, Einarson MB, Shalginskikh N, et al. Identification of a functional network of human epigenetic silencing factors. J Biol Chem. 2010;285:422–433.
- Barbieri E, De Preter K, Capasso M, et al. Histone chaperone CHAF1A inhibits differentiation and promotes aggressive neuroblastoma. Cancer Res. 2014;74:765–774.
- Zhao L, Jiang L, He L, et al. Identification of a novel cell cycle‐related gene signature predicting survival in patients with gastric cancer. J Cell Physiol. 2019; 234:6350–6360.
- Liu T, Wei J, Jiang C, et al. CHAF1A, the largest subunit of the chromatin assembly factor 1 complex, regulates the growth of H1299 human non-small cell lung cancer cells by inducing G0/G1 cell cycle arrest. Exp Therap Med. 2017;14:4681–4686.
- Cai Y, Dong ZY, Wang JY. MiR-520b inhibited metastasis and proliferation of non-small cell lung cancer by targeting CHAF1A. Eur Rev Med Pharmacol Sci. 2018; 22:7742–7749.