Abstract
Green fabrication of nanoparticles has been established by means of a simple, economical and environmentally pleasant process. The present investigation describes the use of Myrtus communis plant leaves extract for the biosynthesis of the silver nanoparticle. The surface plasmon resonance peak at 435 nm, distinctive for silver, determined the synthesis of AgNP. The morphology of the particles was spherical and in size with an average diameter of ∼ 15 nm. Energy dispersive X-ray Fourier Transformed Infrared and X-ray diffraction techniques investigated the crystalline structure, purity and functional groups. The biogenic nanoparticles revealed the antibacterial efficacy against both gram-positive bacteria and gram-negative bacteria, suggesting its prospective applicability as an effective antibacterial agent.
Introduction
Nanoparticle research in the short span of time has grown into one of the utmost important areas in present day science. It has fascinated great curiosity in the areas of biology and medicine [Citation1], due to the idiosyncratic properties of nanoparticles, such as optical, catalytic, electrical, antimicrobial and cancer therapeutic properties. At present, metal nanoparticles with their consummate features are widely studied owing to their possible applicability in various areas such as biological tagging, pharmaceutical applications, coatings and packaging [Citation2, Citation3]. Moreover, because of their physical and chemical features, noble metal nanoparticles are broadly used in the biomedical, electronic and industrial fields [Citation4, Citation5]. Among all the noble metals, silver nanoparticles (AgNP) have engrossed intensive curiosity due to their unique features in catalysis and conductive nature, but precisely their widespread array of encouraging bioactivities. These include antibacterial, anti-fungal, anti-cancer, anti-oxidant and anti-inflammatory efficacy. Silver and its derivatives are being utilized as potent antimicrobial agents so far in therapeutic and preventative health care system [Citation6, Citation7].
Numerous effective synthetic procedures have been devoted to the production of silver nanoparticles, which include physical and chemical procedures, such as chemical, photo-chemical and electrochemical reduction of silver ions. These conventional procedures used hazardous reducing agents during the synthesis and raise environmental and biological risk issues. Green chemistry or biological methodology has received greater attention for environmentally friendly, economical synthesis, which can be performed without the addition of any external reducing or stabilising agent as compared to other available methods [Citation8–11]. A number of green synthetic approaches are available for metals, and in particular silver nanoparticles, using microorganisms. However, there are several issues, including the maintaining of the microbial cultures and multiple purification steps. Plant extract is considered as a sustainable and rapid synthesis strategy for reduction/capping of metallic nanoparticles. At the same time, it eliminates the above concerns and makes non-toxic and biocompatible nanostructures [Citation12–15]. Several investigations exist in literature about the fabrication of silver nanoparticles with possible antibacterial and anticancer bio-activities [Citation16].
Myrtus communis L. (Myrtaceae) is an evergreen shrub that nurtures in the Mediterranean and Middle-Eastern region. It is an evergreen shrub of about 1.8–2.4 m height with small foliage. The leaves are 3–5 cm long with pleasantly fragrant essential volatile oils containing tannins and flavonoids, which are known for their antifungal, antibacterial and antioxidant activities, effective in dealing with cardiovascular disorders [Citation17].
Some of the major uses of leaves are in traditional medicine as antiseptic means in villages, where it is consumed as a decoction. Decoction of leaves and fruits is utilized orally for the cure of disbiosis, hypoglycaemia, stomachaches, cough, constipation, poor appetite, externally for wound healing, urinary infections, enemas and against respiratory diseases [Citation18].
M. communis leaf and bark extracts contain different active secondary metabolites and showed cytotoxic, analgesic and neuropharmacological activities [Citation19]. Fabrication of nanoparticles has not been explored for this plant, where, the extract will be utilized for the production of nanomaterials without adding external reducing and capping molecule. We assume that M. communis can be effectively used for the generation of silver nanoparticles coupled with biological activities. Considering the potential applicability, the present study concentrated on the green fabrication of the silver nanoparticle by M. communis leaf extract, its complete characterization by multiple techniques and the evaluation of their antibacterial activity.
Material and methods
Materials: all chemicals were purchased from Sigma Aldrich (St. Louis, MO)
Biosynthesis of silver nanoparticles
The M. communis plants were collected from the countryside of Alshemasyah (Al-Qassim region, Saudi Arabia). Fresh leaves were collected from the grown plant and brought to the laboratory. The leaves were cleaned under tap water to remove dust particles from the surfaces. Small pieces of washed leaves were then surface sterilized with alcohol (70%) in Laminar Air flow under aseptic conditions. About 10 g of fresh cut leaves were boiled in 200 mL of sterile water at 90 °C for 40 min to get an aqueous extract. The supernatant was allowed to cool down at room temperature, filtered through Whatman No. 1 followed by 0.22 sized filters [Citation20] and further used to synthesize silver nanoparticles by reacting aqueous silver nitrate (1 mmol/L) solution (90 mL) with extract (10 mL) for reduction of ions into nanoparticles
Characterization of nanoparticles
The reaction mixture containing silver nitrate and fresh leaf extract was monitored visually for colour changes (characteristic for silver nanoparticles). For the formation of AgNP, aliquots of the suspension were subjected to optical analysis by UV–Vis spectrophotometer Lambda 35 (Perkin-Elmer, Germany) at a resolution of 1 nm and recording of the spectra in the range of 200 to 800 nm. TEM grid (carbon-coated copper grid) was prepared by drop casting of the nanoparticles suspension and analysed on a JEOL microscope (JEM-1011) system, operated at an accelerating voltage of 120 kV at room temperature. Energy dispersive analysis of X-Ray (EDAX) was performed on Altima IV (Make: Regaku, Japan) over the nanoparticles coated surface in spot profile mode to further confirm purity. X-ray diffraction spectrogram was obtained by casting a thin layer of nanoparticles on a glass substrate and performed on Rigaku Miniflex X-ray diffractometer (Rigaku, Kα (λ = 1.5406 A) in the 2θ range of 20 to 80 °C with a scanning rate of 0.05 mV/s. FTIR spectra of nanoparticles suspension were measured on a Perkin Elmer 1000 (USA) FT-IR instrument in diffuse reflectance (DRS) mode.
Antibacterial activity
Disk diffusion assay was carried out for the antibacterial activity assay [Citation21]. Bacterial cultures of Methicillin-resistant Staphylococcus aureus (MRSA) ATCC 43300 and Escherichia coli ATCC 35218 were inoculated in nutrient broth and incubated at 37 °C for 24 h. Bacterial suspension of 100 µL (0.1 mL) (concentration 105 CFU/mL) was overlayed on nutrient agar plates and incubated at 37 °C for 15 min. Sterile cork borer was used to make wells in the agar, and each well was loaded with 100 µL of plant extract and nanoparticles, whereas 30 µg per disc of gentamicin and vancomycin were treated as positive controls and distilled water, as a negative control. Plates were incubated at 37 °C for 24 h and visually monitored for the inhibition zone and measured in millimetres across the well. The assay was carried out in triplicate for the confirmation of antibacterial activity of AgNP.
Determination of minimum inhibitory concentration (MIC)
Minimal concentration of silver nanoparticles which shows inhibition of visible bacterial growth or turbidity was referred as MIC.
To obtain aqueous AgNP, we prepared serial dilutions of the initial concentration of 200 µg/mL in Mueller-Hinton broth on a 96-well microtiter plate. Then, 100 µL of bacterial inoculum (methylene resistant S. aureus or E. coli), which was cultured overnight and adjusted to 0.5 Mc Farland units, was added to each well. The experiment was performed in triplicates. The culture plate was incubated overnight at 37 °C for 24 h. Vancomycin and gentamicin were used as positive controls and Mueller-Hinton broth was used as negative control. Forty microlitres of 2,3,5-triphenyltetrazohum chloride (TTC) with a final concentration of 2 mg/mL dissolved in water was added to the overnight cultured plates for 30 min at 37 °C [Citation22].
Determination of minimum bactericidal concentration (MBC)
Lowest concentration of silver nanoparticles that kill 100% of bacteria is known as MBC, which is determined same as MIC carried out on a 96-well plate. Hundred microlitre from the wells with no turbidity or bacterial growth was cultured on Mueller-Hinton agar plates to test bactericidal activity of silver nanoparticle incubated at 37 °C for 24 h. MBC value is stated as the lowest concentration causing bactericidal effect with no visible viable colonies on an agar plate.
Results and discussion
The growth of nanotechnology is projected towards the technological development and provides an understanding of the fundamental properties of objects. Plants are a rich source for pyto-constituents and are being regularly used in the development of greener routes for production of nanoparticles. To address this, we have collected M. communis and got leaves extract as shown in .
The leaf extract when incubated with silver nitrate, exhibited a gradual conversion in colour (yellow to brown) (). The mixture without silver nitrate (Control) did not demonstrate any deviation in colour. The UV-visible spectrum in shows a strong peak at 435 nm by the surface plasm on resonance [Citation23], which confirmed formation of the silver nanoparticle. This may have occurred due to the reduction of silver ion by bioactive molecules present in the reaction mixture. The biomolecule signature around 280 nm depicts proteinaceous nature. Inset in shows capping of nanoparticles surface. Zeta potential of silver nanoparticles was found to be –24.6 mV () which makes nanoparticles stable for longer time without flocculation in reaction mixture.
Figure 2. Flasks before and after reacting with silver nitrate (A), UV-Visible spectrum of the silver nanoparticle and capping of nanoparticle surface (B) and Zeta potential of nanoparticles (C).
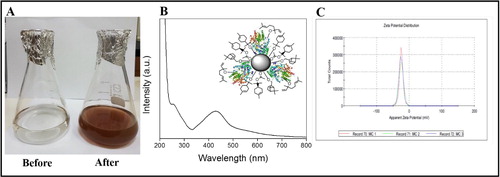
The bio-synthesized silver nanoparticles were further confirmed for structural features such as morphology and crystalline nature by TEM micrograph (). The nanoparticles size dimension ranges from 5 to 30 nm.
TEM micrograph depicts that majority of the particles were spherical in shape. Furthermore, elemental silver in the sample was probed by EDAX analysis illustrated in , the obtained spectrum shows produced silver nanoparticles and the mass % is ∼ 75% of the sample.
The X-ray powder diffraction (XRD) patterns of the formed silver nanoparticles shown in . Bragg reflections with 2θ values and its corresponding lattice planes was shown as 38.15°(111), 44.14°(200), 64.50°(220) and 77.25°(311), shows face centred cubic (fcc) crystal structure of Ag NPs, as per the Joint Committee on Powder Diffraction Standards (JCPDS) Card no.04-0783.
Fourier-transform infrared spectroscopy (FTIR) spectroscopy was used to probe the possible biological moiety. The vibrational frequencies in the range of 400 to 4000 cm−1 are shown in . FTIR analysis revealed the occurrence of diverse functional groups in the mixture. The FTIR signature appear at 3440 (NH2), 2931 (-CH), 2852 (-CH), 1633 (Amide-I), 1038 (-CN), 672 (Halides like Cl, Br and I) were assigned to stretching vibration of –NH band of amino C–H stretch of alkanes, -NH of an amide group present in the reaction mixture, C-O stretching of carboxylic acid and derivatives, C-N of aliphatic amines or alcohol/phenol, N-H deformation of amines and C-H deformation of alkynes, respectively.
Silver nanoparticles possess promising applications in antimicrobial effect, biomedical and drug delivery. The propagation of different antimicrobial resistant bacterial strains has been on increase, due to the uncontrolled practices of certain antibiotics. In this setting, the marked antibacterial efficacy of the silver nanoparticles could be valuable for evolving antibacterial drugs against pathogenic bacterial strains. In the present study, the synthesized nanoparticles were assessed for antibacterial activity efficacy MRSA and E. coli by disc diffusion method (). The results revealed that silver nanoparticle exhibited significant inhibition activity against MRSA () and E. coli () with respect to plant extracts. Recent study on Buffer extraction of M. communis showed strong sensitivity against Gram-positive bacteria [Citation24]. Measurements of zone of inhibition was presented in .
Figure 5. Antibacterial activity of silver nanoparticles against methicillin-resistant S. aureus (A) and E. coli (B).
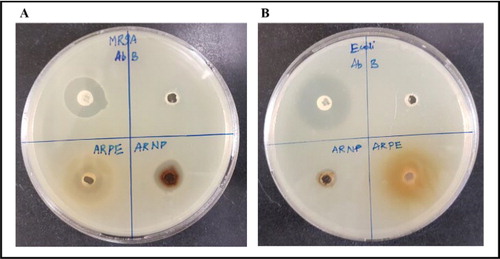
Table 1. Zone of inhibition of plant extract (ARPE) and nanoparticle (ARNP) against methicillin-resistant S. aureus (MRSA) and E. coli.
The colourless compound (TTC) acts as an electron acceptor and is reduced to red colour by a biologically active organism. MIC value of synthesized aqueous silver nanoparticles against MRSA was 12.5 µg/mL, for E. coli - 25 µg/mL in comparison to vancomycin - 3 µg/mL and gentamicin - 6 µg/mL (). Ajdari et al. [Citation24] reported MIC value of E. coli 24.5 µg/mL and of MRSA - 16.3 µg/mL against silver nanoparticles from M. communis leaf extract which coincided with our results. MBC was observed to be 25 µg/mL and 50 µg/mL respectively for MRSA and E. coli in reference to Citation6 µg/mL for vancomycin and 12 µg/mL for gentamicin which were lower values obtained for our study as compared to Ajdari et al. [Citation24].
Table 2. MIC and MBC of silver nanoparticle against E. coli and methicillin-resistant S. aureus (MRSA).
Conclusions
We have established a one-pot synthesis for silver nanoparticles by means of leaves extract of M. communis plant. The synthesis process is simple, reproducible and ecofriendly. Fabrication of nanoparticles was confirmed by analytical techniques, UV-Visible, TEM and XRD. These nanoparticles showed antibacterial efficacy towards Gram-positive and Gram-negative bacteria. This biogenic silver can be useful in different antimicrobial applications such as gel, coatings, bandages and so forth.
Data availability
The data used to support the findings of this study are included in the article. Any specific details will be delivered by corresponding author upon request.
Acknowledgments
Authors extend their appreciation to the Deanship of Scientific Research at King Saud University for providing funding this project through Research Group No. RG-1440-53.
Disclosure statement
No potential conflict of interest was reported by the authors.
References
- Salata OV. Applications of nanoparticles in biology and medicine. J Nanobiotechnol. 2004;2:3.
- De M, Ghosh PS, Rotello VM. Applications of nanoparticles in biology. Adv Mater. 2008;20:4225–4241.
- Radwan SH, Azzazy HM. Gold nanoparticles for molecular diagnostics. Expert Rev Mol Diagn. 2009;9:511–524.
- Barapatre A, Aadil KR, Jha H. Synergistic antibacterial and antibiofilm activity of silver nanoparticles biosynthesized by lignin-degrading fungus. Biores Bioprocess. 2016;3:8.
- Zhang X-F, Liu Z-G, Shen W, et al. Silver nanoparticles: synthesis, characterization, properties, applications, and therapeutic approaches. IJMS. 2016;17:1534.
- Chaloupka K, Malam Y, Seifalian AM. Nanosilver as a new generation of nanoproduct in biomedical applications. Trends Biotechnol. 2010;28:580–588.
- Chen X, Schluesener HJ. Nanosilver: a nanoproduct in medical application. Toxicol Lett. 2008;176:1–12.
- Peralta-Videa JR, Huang Y, Parsons JG, et al. Plant-based green synthesis of metallic nanoparticles: scientific curiosity or a realistic alternative to chemical synthesis?. Nanotechnol Environ Eng. 2016;1:4.
- Ovais M, Khalil AT, Raza A, et al. Green synthesis of silver nanoparticles via plant extracts: beginning a new era in cancer theranostics. Nanomedicine. 2016;12:3157–3177.
- Kim D-Y, Kim M, Shinde S, et al. Temperature Dependent synthesis of tryptophan-functionalized gold nanoparticles and their application in imaging human neuronal cells. ACS Sustain Chem Eng. 2017;5:7678–7689.
- Saratale RG, Shin H-S, Kumar G, et al. Exploiting fruit byproducts for eco-friendly nanosynthesis: Citrus × clementina peel extract mediated fabrication of silver nanoparticles with high efficacy against microbial pathogens and rat glial tumor C6 cells. Environ Sci Pollut Res. 2018;25:10250–10263.
- Atanasov AG, Waltenberger B, Pferschy-Wenzig E-M, et al. Discovery and resupply of pharmacologically active plant-derived natural products: a review. Biotechnol Adv. 2015;33:1582–1614.
- Ankamwar B, Sur UK, Das P. SERS study of bacteria using biosynthesized silver nanoparticles as the SERS substrate. Anal Methods. 2016;8:2335–2340.
- Dahoumane S, Jeffryes C, Mechouet M, et al. Biosynthesis of inorganic nanoparticles: a fresh look at the control of shape, size and composition. Bioengineering. 2017;4:14.
- Shankar SS, Rai A, Ankamwar B, et al. Biological synthesis of triangular gold nanoprisms. Nat Mater. 2004;3:482.
- Venkatesan J, Lee J-Y, Kang DS, et al. Antimicrobial and anticancer activities of porous chitosan-alginate biosynthesized silver nanoparticles. Int J Biol Macromol. 2017;98:515–525.
- Dulger B, Gonuz A. Antimicrobial activity of certain plants used in Turkish traditional medicine. Asian J Plant Sci. 2004;3:104–107.
- Serce S, Ercisli S, Sengul M, et al. Antioxidant activities and fatty acid composition of wild grown myrtle (Myrtus communis L.) fruits. Phcog Mag. 2010;6:9.
- Sumbul S, Ahmad MA, Asif M, et al. Evaluation of Myrtus communis Linn. berries (common myrtle) in experimental ulcer models in rats. Hum Exp Toxicol. 2010;29:935–944.
- Venkatesan J, Kim S-K, Shim MS. Antimicrobial, antioxidant, and anticancer activities of biosynthesized silver nanoparticles using marine algaEcklonia cava. Nanomaterials. 2016;6:235.
- Bauer A, Kirby W, Sherris JC, et al. Antibiotic susceptibility testing by a standardized single disk method. Am J Clin Pathol. 1966;45:493–496.
- Eloff JN. A sensitive and quick microplate method to determine the minimal inhibitory concentration of plant extracts for bacteria. Planta Med. 1998;64:711–713.
- Mulvaney P. Surface plasmon spectroscopy of nanosized metal particles. Langmuir. 1996;12:788–800.
- Ajdari MR, Tondro GH, Sattarahmady N, et al. Phytosynthesis of silver nanoparticles using Myrtus communis L. leaf extract and investigation of bactericidal activity. J Electron Mater. 2017;46:6930–6935.