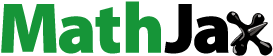
Abstract
Bacillus amyloliquefaciens LZN01 shows antagonistic behaviour against Fusarium oxysporum f. sp. niveum (Fon). This study aimed to analyze the antifungal activity and the functional components of cell-free supernatant from B. amyloliquefaciens LZN01 that inhibited Fon growth. The results showed that the cell-free supernatant (CFS) from the death phase had stronger antifungal activity against Fon compared to those from the exponential stage and stationary phase. The analyses from scanning and transmission electron microscopy demonstrated that treatment with CFS led to cellular morphological changes, including concave formations on the conidial surface, the disruption of cell walls and membranes, the leakage of intracellular contents and the aggregation of organelles. Confocal laser scanning microscopy revealed that the CFS damaged membrane integrity. Myriocin, sphingofungin E, sphingofungin F, 3-methyl-2-oxovaleric acid, gabapentin and sphingofungin C were confirmed as the major differential metabolites by ultra-high-performance liquid chromatography-LTQ orbitrap (UHPLC-LTQ Orbitrap) MS analysis. Quantitative analysis showed that the myriocin content in the CFS was 0.6 μg/mL, and its minimum inhibitory concentration (MIC) was 1.25 μg/mL. It can be speculated that some antifungal compounds in the CFS, including myriocin, sphingofungin E, sphingofungin F and sphingofungin C, play major roles in inhibiting the growth of Fon and the synergistic effects among these antifungal compounds are important in suppressing Fon reproduction. This study suggests that B. amyloliquefaciens LZN01 is a promising biological agent against Fon as its CFS exerts antifungal activity by deforming conidial structures and damaging membranes and myriocin was one of the major functional components of the CFS.
Introduction
Fusarium oxysporum f. sp. niveum (Fon) is a soil-borne pathogen that causes Fusarium wilt of watermelon and severe losses to watermelon yield in all cultivation regions of the world. Fon enters the plant through the roots of the watermelon, where it inhabits the xylem, resulting in watermelon wilt and eventual plant death [Citation1]. The chlamydospores of Fon can survive for as long as 8 years in the soil and the intensive cultivation of watermelon on limited agricultural lands causes Fon to persist longer in fields, making the control of Fon difficult [Citation2]. Different methods, such as disease-resistant cultivars [Citation3], grafting [Citation4,Citation5] and intercropping [Citation6] were utilized to overcome Fusarium wilt of watermelon.
Biocontrol is an effective and sustainable alternative method to suppress the growth of Fon [Citation7,Citation8]. Different microbial species have been used to control soil-borne diseases, including Pseudomonas spp. [Citation9], Paenibacillus spp. [Citation10], Trichoderma spp. [Citation7], Streptomyces spp. [Citation8], Bacillus spp. [Citation11], and nonpathogenic Fusarium spp. [Citation12]. The antagonistic mechanisms of the strains involved the production of antibiotics, antifungal compounds, cell wall-degrading enzymes, hydrogen cyanide, catalase, siderophores and plant growth-promoting hormones; the induction of systemic resistance in plants; the increase of the phosphorus and potassium absorption in the plant; and the competition for nutrients and space [Citation9,Citation13,Citation14]. A number of Bacillus species, especially Bacillus subtilis and Bacillus amyloliquefaciens, possess antimicrobial activity, and their antifungal compounds are responsible for the suppression of plant pathogens [Citation15]. Lipopeptide extracts from B. amyloliquefaciens FZB42 displayed antifungal activity against Fusarium oxysporum and Fusarium solani [Citation16], B. amyloliquefaciens L3 produced 2-nonanone and 2-heptanoneplayed important roles in controlling the growth of Fon [Citation17]. In previous studies, wheat as a companion crop decreased the Fon population, changed the abundance of microbial communities in the rhizosphere of watermelon and reduced Fusarium wilt in watermelon [Citation18]. B. amyloliquefaciens LZN01, as a biocontrol agent against Fon, was isolated from the rhizosphere of wheat in the watermelon/wheat companion system.
Mass spectrometry (MS)-based metabolomic analysis has become a method to study the changes in the metabolome of various microorganisms during their different growth phases, such as B. subtilis [Citation19], Skeletonema marinoi [Citation20] and Lactobacillus sakei [Citation21]. However, the changes in the metabolome of the B. amyloliquefaciens during different growth phases were not investigated.
In this study, our objectives were: (1) to evaluate the capability of cell-free supernatant (CFS) from B. amyloliquefaciens LZN01 at different growth phases to inhibit Fon mycelial growth, (2) to determine the effect of CFS from B. amyloliquefaciens LZN01 on the microscopic morphology of conidia and membrane integrity of Fon, (3) to analyze the differential metabolites of B. amyloliquefaciens LZN01 at different growth phases by ultra-high-performance liquid chromatography-LTQ orbitrap (UHPLC-LTQ Orbitrap) MS and (4) to determine the minimum inhibitory concentration (MIC) of the major functional component.
Materials and methods
Materials
B. amyloliquefaciens LZN01 and Fon used in the study were provided by the Laboratory of Microbial Ecology, Department of Life Sciences, Agriculture and Forestry, Qiqihar University in China.
Cultivation of B. amyloliquefaciens LZN01
The growth of B. amyloliquefaciens LZN01 was evaluated [Citation22]. Briefly, B. amyloliquefaciens LZN01 was inoculated into Luria-Bertani (LB) medium and cultured for 12 h. Next, 1 mL cultures were used to inoculate different flasks containing 100 mL LB. The cultures were incubated at 30 °C, shaking at 130 rpm, and cell growth was monitored every hour by measuring the OD600nm.
Together up, 1 mL cultures were inoculated into LB medium and cultivated at 30 °C, with shaking at 130 rpm for 12 h, 24 h, 48 h, 60 h or 72 h. The culture was centrifuged at 12,000 rpm for 15 min at 4 °C. The supernatant was collected and sterilized through a membrane filter (0.22 μm), which was used as the CFS at 12 h, 24 h, 48 h, 60 h and 72 h.
Fon mycelial growth
To assess the effect of the CFS from different culture times on Fon mycelial growth, peri dish experiments were performed [Citation23]. The CFS (10 mL) at each time point (12 h, 24 h, 36 h, 48 h, 60 h and 72 h) and autoclaved potato-dextrose-agar medium (PDA, 5 mL) were mixed and added to a petri dish (90 mm diameter). The supernatant of LB medium was used as a control. The petri dishes were inoculated with 4 mm diameter agar plugs cut from the edge of 1-week-old Fon colonies. The inoculated plates were placed upside down and incubated in a growth chamber at 28 °C. The colony diameters were measured in two directions on each plate after incubation for 5 days. The inhibition ratio was calculated using the formula (1):
where C is the colony diameter of the control plate and T is the colony diameter of treatment plate after culturing for 5 days. Each treatment consisted of five replicates.
Scanning and transmission electron microscopy observation of conidial morphologies
Fon mycelium was grown in dish plates with PDA in the dark at 28 °C for 7 days to induce sporulation as described previously [Citation24]. The plates were drenched with sterile distilled water and the conidia were carefully freed from the culture surface with a fine brush. Afterwards, the suspension was filtered through four layers of sterile cheesecloth to remove mycelial fragments. The conidia concentration was determined using a hemocytometer.
To observe the morphological changes of conidia caused by the CFS, electron microscopy was used. The CFS from B. amyloliquefaciens LZN01 cultured for 72 h was mixed with Fon conidial suspensions at a ratio of 1:1 and 3:1 (V/V) (final contents of the CFS were 50% and 75%). Moreover, to serve as a control, the CFS of LB medium cultured 72 h that did not have the LZN01 isolate added was mixed with the Fon conidial suspensions at the same proportions. After culturing for 12 h, scanning and transmission electron microscopy analyses were used to determine the effects of the CFS on the conidia of Fon at the ultrastructural level. For scanning electron microscopy (SEM), conidia treated with the CFS were centrifuged and prefixed with 2.5% glutaraldehyde. Fixed cells were rinsed three times for 10 min with 100 mM phosphate buffer, postfixed for 3 h in 1% osmium tetraoxide and dehydrated through an ethanol gradient. The samples were then coated with gold and analyzed on a scanning electron microscope (S-3400, Hitachi, Japan). For transmission electron microscopy (TEM) analysis, samples were embedded in Epon 812, sectioned using an ultra-microtome and examined under a Hitachi H-7650 transmission electron microscope.
Assay of Fon membrane integrity
To assess the damage to Fon cell membranes caused by the CFS, confocal laser scanning microscopy (CLSM) analyses using reagents from the LIVE/DEAD BacLight™ Viability kit L7012 (MA, USA) were performed as described by Sun et al. [Citation25] with some modifications. SYTO 9 generally stains live and dead cells in a population, whereas propidium iodide (PI) penetrates cells only when the membrane is damaged, causing a reduction in SYTO 9 fluorescence when both dyes are present. Thus, the cells with intact membranes will exclude PI but be stained by SYTO 9 and emit green fluorescence, whereas the cells with a damaged membrane will be stained by PI and emit red fluorescence. The CFS cultured for 72 h was mixed with the Fon suspensions at a ratio of 3:1 (V/V) and incubated for 12 h, 24 h, 36 h and 48 h. The CFS of LB medium cultured for 72 h was mixed with the Fon suspensions at a ratio of 3:1 (V/V) as a control. The control and the CFS of LZN01-treated cell suspensions were centrifuged at 10,000 rpm for 5 min at 4 °C. Then, the cells were resuspended in a small volume of sterile water. Following the addition of dye solutions (an appropriate mixture of the SYTO 9 and PI stains), the mixtures were incubated for 30 min at room temperature in the dark. After being washed and centrifuged, the cells were examined under a confocal laser scanning microscopy (CLSM) (TCS SP8, Leica, Germany). In all cases, a 63× objective oil immersion lens was used.
UHPLC-LTQ orbitrap MS analysis
B. amyloliquefaciens LZN01 was cultured at 30 °C, with shaking at 130 rpm for 12 h or 72 h in LB broth. B. amyloliquefaciens LZN01 cultures were centrifuged at 12,000 rpm for 15 min at 4 °C. The supernatants were filtered using a syringe filter (0.22 μm) and collected. The supernatant of LB medium cultured for 72 h was used as a control.
For LC-MS analysis, 1 mL of CFS was taken and 20 μL internal standard (L-2-chlorphenylalanine, 0.3 mg/mL, preparation with methanol) was added to lyophilize the samples in a centrifugal tube. The reaction mixtures were vortexed for 30 s with 300 μL methanol-acetonitrile and sonicated for 5 min in an ice bath. Then, the samples were centrifuged at 13,000 rpm for 15 min at 4 °C. After centrifugation, 200 μL of CFS was loaded into the LC-MS vial, and LC-MS analysis was carried out.
Metabolites extracted from B. amyloliquefaciens LZN01 cells and their growth media were analyzed on a UHPLC system (Waters, USA). The samples were injected into an Acquity UHPLC BEH C18 column (100 × 2.1 mm i.d., 1.7 µm; Waters, USA) equilibrated with water containing 0.1% formic acid and eluted in a gradient with acetonitrile containing 0.1% formic acid at a flow rate of 0.4 mL/min for 14.5 min. The eluted metabolites were analyzed using LTQ Orbitrap MS equipped with an electrospray ionization (ESI) source operating in either the positive or negative ion mode. The capillary voltages, sampling cones and collision voltages were set at 3 kV, 40 V and 30 eV, respectively. The capillary and source temperature were set at 350 °C and the desolvation gas flow rate was 45 L/h. Centroid data were collected in the m/z 50–1000 range with a 30,000 resolution. The QC (quality control) sample was prepared by mixing aliquots of all samples to generate a pooled sample, which was analyzed using the same method as the analytic samples. The QCs were injected at regular intervals (every 10 samples) throughout the analytical run to provide a set of data from which repeatability can be assessed.
The positive and negative data were combined to obtain a combined data set that was imported into the SIMCA-P + 14.0 software package (Umetrics, Umeå, Sweden). Principle component analysis (PCA) and (orthogonal) partial least-squares-discriminant analysis (O)PLS-DA were carried out to visualize the metabolic alterations among experimental groups after mean centering and unit variance scaling. Variable importance in the projection (VIP) ranks the overall contribution of each variable to the (O)PLS-DA model and those variables with VIP > 1.0 were considered relevant for group discrimination. In the study, the default seven-round cross-validation was applied with 1/7 part of the samples being excluded from the mathematical model in each round to prevent overfitting.
To assess the effect of differential metabolites on Fon mycelial growth, petri dish experiments were performed and the methods were the same as those in section of Fon Mycelial Growth.
Quantitative analysis of myriocin in the CFS from B. amyloliquefaciens LZN01
Quantitative analysis of myriocin in the CFS was performed using UPLC-Q-Exactive Orbitrap (Thermo, USA). The stock solution of myriocin was prepared in dimethyl sulfoxide (DMSO) at 5 mg/mL. The working solutions at 1000, 200, 40, 8, 1.6, 0.32, 0.064, 0.0128 and 0.00256 μg/mL were prepared by serial dilution from the stock solution with methanol. Triplicates were prepared and analyzed at no less than seven appropriate concentration levels. The calibration linearity for myriocin was evaluated by plotting the peak area of multiple reaction monitor transitions of standards against the concentrations. Regression analysis was used to calculate linear regression equations for standards using SPSS 20.0. The preparation of the CFS was the same as that described in Fon mycelial growth section. The chromatographic separation was performed on Agilent SB-C18 (100 mm × 3.0 mm, 1.8 μm). The column temperature was maintained at 22 °C. The mobile phase consisted of water containing 0.1% formic acid (A) and methanol (B). The mobile phase flow rate was set to 0.3 mL/min.
Determination of the MIC of myriocin against Fon
The minimum inhibitory concentration (MIC) of myriocin against Fon was determined using a standard microdilution method [Citation25]. To ensure the solubility of myriocin, myriocin was dissolved in DMSO and then diluted to its final desired concentration with the DMSO concentration at less than or equal to 1% [Citation26]. Briefly, the initial concentration of myriocin was 3.2 mg/mL and was serially diluted to 6.25 μg/mL for use. Ten microlitres of myriocin solution was added to the wells of a 96-well microtiter plate. Then, 10 μL Fon conidial suspension (106 CFU/mL) from log-phase was added and 180 μL potato dextrose (PD) medium was added. The absorbance at 615 nm was recorded using a microplate reader Victor X (PerkinElmer Inc., USA) after the cultures were incubated for 24 h at 30 °C. The MIC was defined as the lowest myriocin concentration that inhibited 80% of conidial growth. The sample treated with amphotericin B was considered as positive control. The sample treated with sterile water was considered as negative control. The inhibition percentage of conidial growth was calculated with the following formula:[Citation27] inhibition (%) = {(OD615 nm of sample treated with amphotericin B – OD615 nm of sample treated with myriocin)/(OD615 nm of sample treated with amphotericin B – OD615nm of sample treated with sterile water)} × 100%. The MIC values were determined by three independent assays.
Results and discussion
Effect of CFS from B. amyloliquefaciens LZN01 on Fon mycelial growth
The growth dynamics of B. amyloliquefaciens LZN01 was monitored in liquid LB medium. As shown in , the lag phase of B. amyloliquefaciens LZN01 was from 0 to 4 h. The growth exponential stage was from 4 to 32 h. The stationary phase was from 32 to 36 h. The growth of B. amyloliquefaciens LZN01 entered the death phase after 36 h.
Figure 1. Curve of B. amyloliquefaciens LZN01 growth (A) and the effects of the CFS on Fon mycelial growth (B). 12 h, 24 h, 36 h, 48 h, 60 h and 72 h: the CFS from B. amyloliquefaciens LZN01 in relation to the incubation time.
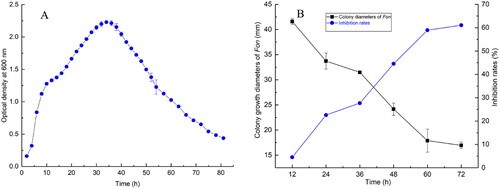
Bacillus species are commonly found in nature and possess antifungal effects against various fungal plant pathogens [Citation16,Citation17]. To assess the effect of the CFS from B. amyloliquefaciens LZN01on Fon mycelial growth, the CFS at12 h, 24 h, 36 h, 48 h, 60 h and 72 h was prepared and petri dish experiments were performed. The results showed that the CFS significantly inhibited the mycelial growth of Fon and the inhibition rate of the CFS at 12 h, 24 h, 36 h, 48 h, 60 h and 72 h was 4.50%, 22.58%, 27.70%, 44.54%, 58.92% and 61.06%, respectively (). This result suggested that B. amyloliquefaciens LZN01 secreted different amounts of antimicrobial substances in different growth stages.
Effect of CFS from B. amyloliquefaciens LZN01 on the microscopic morphology of Fon conidia
The conidial structures of Fon were observed using SEM and TEM (). SEM imaging showed that Fon conidia were replete and intact and grew normally when untreated with the CFS (). When treated with the CFS, damaged and deformed structures of conidia were observed ().The number and degree of damaged conidia increased with the increasing proportion of CFS (). TEM micrographs of the conidia untreated with the CFS showed that the cell walls were intact, the cell membranes were well-defined and all cell components, especially the cellular organelles, such as the cell nucleus and vacuole, were visibly arranged appropriately and had homogeneous electron density in the cytoplasm (). In contrast, Fon conidia treated with the CFS exhibited abnormalities, including disruption of cell walls and membranes (white arrows), leakage of intracellular contents (red arrows), the appearance of larger vacuoles and the aggregation of organelles (). Most antimicrobial substances target the cell membranes of pathogenic microorganisms, lyse the cell membranes and lead to death [Citation28]. This result indicated that antifungal substances in the CFS clearly affected the growth of Fon through morphological changes, collapse of conidial surface structures and disruption of membranes.
Figure 2. SEM and TEM micrographs of Fon conidia treated with the CFS from B. amyloliquefaciens LZN01: (A) 50% CFS from LB medium at 72 h (SEM), (B) 50% CFS from B. amyloliquefaciens LZN01 at 72 h (SEM), (C) 75% CFS from LB medium at 72 h (SEM), (D) 75% CFS from B. amyloliquefaciens LZN01 at 72 h (SEM), (E) 50% CFS from LB medium at 72 h (TEM), (F) 50% CFS from B. amyloliquefaciens LZN01 at 72 h (TEM), (G) 75% CFS from LB medium at 72 h (TEM), (H) 75% CFS from B. amyloliquefaciens LZN01 at 72 h (TEM).
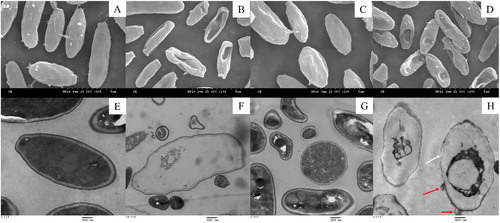
Effect of CFS from B. amyloliquefaciens LZN01 on Fon cell membrane integrity
Cells without a cell membrane or damaged cell membrane could be designated as dead cells [Citation29]. The cell membrane structural integrity was analyzed by CLSM combined with fluorescent probes SYTO 9 and PI, which could distinguish intact from membrane-damaged cells. After 12 h of treatment with the CFS, a small amount of yellow and red fluorescence was observed (), which may be attributed to the increase in cytoplasmic membrane permeability. After 24 h of treatment, the red intensity was enhanced as a fraction of the treated cells lost membrane integrity (). After 36 h, a small fraction of the treated cells emitted green fluorescence (). After 48 h, an overwhelming fraction of the Fon conidia and mycelia emitted red or yellow fluorescence with little green fluorescence (). The obtained data suggested that antifungal substances in the CFS damaged the cell wall or cell membrane of Fon, which could lead to leakage of nucleic acid and proteins, destruction of the balance of cell metabolism, and finally, to apoptosis of Fon. The results were consistent with TEM analysis ().
Multivariate statistical analysis of metabolites from B. amyloliquefaciens LZN01
The profiles of B. amyloliquefaciens LZN01 and medium metabolites detected by LC-MS and UHPLC-LTQ Orbitrap MS were statistically analyzed by multivariate statistical analysis, including PCA and (O)PLS-DA, to visualize the differences among the samples and to find metabolites contributing to these differences (). Prior to (O)PLS-DA analysis, significant outliers identified by the PCA-Hotelling’s T2 test, at a 95% confidence interval of the modeled variation, were excluded from further analysis. B. amyloliquefaciens LZN01 (12 h and 72 h) and medium sample (CK) were clearly separated on the first two-component (O)PLS-DA score plots. The (O)PLS-DA model quality parameters were as follows: R2X = 0.942, R2Y = 0.992 and Q2 = 0.979 for 12 h-72 h; R2X = 0.929, R2Y = 0.991 and Q2 = 0.973 for 72 h-CK; and R2X = 0.909, R2Y = 0.991 and Q2 = 0.955 for 12 h-CK.
Figure 4. Principle component analysis (A) and (orthogonal) partial least squares discriminant analysis (B, C and D) score plots of the datasets obtained by the LC-MS and UHPLC-LTQ Orbitrap MS analysis of B. amyloliquefaciens LZN01 and its growth medium. The quality of the (O)PLS-DA models was evaluated by R2X, R2Y, Q2Y and p values and validated by 200 permutation tests.
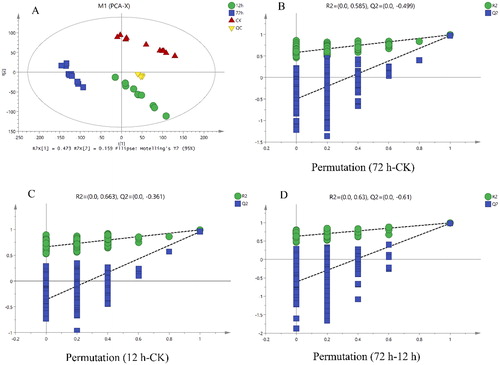
Identification of metabolites
Among metabolites detected by LC-MS and UHPLC-LTQ Orbitrap MS, statistical analysis of the normalized metabolites showed that 195, 133 and 155 metabolites changed significantly for 72 h-CK, 12 h-CK and 12 h-72 h, respectively (Supporting Information Table S1). Variable importance in the projection (VIP) ranks the overall contribution of each variable to the (O) PLS-DA model, and those variables with VIP > 1.0 and p value <0.05 were considered relevant for group discrimination. In this study, the default seven-round cross-validation was applied with 1/7th of the samples being excluded from the mathematical model in each round to prevent overfitting.
Changes in the levels of metabolites identified in the CFS were visualized according to heat maps (Supporting Information Figure S1). The levels of N-palmitoyl alanine, N-palmitoyl glycine, sphingofungin E, sphingofungin F, myriocin, quinapril, 3-methyl-2-oxovaleric acid, phaseolic acid, stemonacetal, decanoylcarnitine, tyrosyl-histidine, pantothenic acid, s-japonin, gabapentin, nicotinuric acid, scillaren A and sphingofungin C were increased in the CFS at 12 h and 72 h compared to the control (CK). Sphingofungin E, sphingofungin F, myriocin,3-methyl-2-oxovaleric acid, gabapentin and sphingofungin C did not exist in the control (, Supporting Information Table S1). Sphingofungin C only existed in the CFS at 12 h, and 3-methyl-2-oxovaleric acid only appeared in the CFS at 72 h.
Analysis of the functional component inhibiting Fon
It has been reported that bacterial antibiotics and other secondary metabolites present in bacterial isolates might be responsible for the inhibition of pathogen growth, for which antibiosis is the major mode of action for disease control [Citation16,Citation17,Citation30]. To verify the functional component inhibiting Fon, some major differential metabolites were measured. Gabapentin is a derivative of gamma-aminobutyric acid, which has antifungal activity against fungi such as Aspergillus niger and Penicillium chrysogenum [Citation31]. In this study, gabapentin at 1 and 2 mg/mL could not inhibit the mycelial growth of Fon, but gabapentin at 5 and 10 mg/mL had the potential to inhibit Fon (). Myriocin was first described by Kluepfel et al. [Citation32] and later isolated from Myriococcum albomyces, Melanconis flavovirens, Isaria sinclairii and Paecilomyces variotii ATCC 74097 [Citation33]. Myriocin exhibits antifungal properties against several pathogens, such as Alternaria solani, Candida albicans, Candida parapsilosis, Candida pseudotropicalis, Candida tropicalis, Ceratocytis ulmi¸ Cryptococcus laurentii, Fusarium oxysporum, Rhizomucor miehei and Streptomyces spp. [Citation33]. The results showed that myriocin at 100 and 200 μg/mL significantly inhibited the mycelial growth of Fon. The inhibition rate of 200 μg/mL myriocin reached 42.67% (), whereas 3-methyl-2-oxovaleric acid could not inhibit the mycelial growth of Fon ().
Quantitative analysis of myriocin in LZN01 CFS
The linear regression equation of myriocin was as follows: R2 = 0.9896. The quantitative analytical result was shown in . The results indicated that myriocin was not detected in CK. Myriocin with m/z at 402.2966 was detected at 9.42 and 9.45 min in the CFS from B. amyloliquefaciens LZN01 at 12 h and 72 h, respectively. The myriocin content in the CFS was approximately 0.6 μg/mL. The results indicated that myriocin was a functional component of B. amyloliquefaciens LZN01that inhibited Fon.
Antifungal activity of myriocin
The MIC of myriocin against Fon was 1.25 μg/mL. To further investigate the antifungal activity of myriocin against Fon, a time-kill assay was carried out to study the concentration and time-dependent killing effect. As shown in , the higher concentration of myriocin led to a slower increase in optical density. The growth of Fon was suppressed by myriocin at the MIC, indicating a fungistatic effect. In the treatments with myriocin at 4MIC, the optical density was significantly lower than the value of the MIC treatment after 24 h and the fungistatic effect was 87.42% at 10 h. When the concentration was set at 8MIC, a better fungistatic effect was observed at 6–18 h with myriocin compared to amphotericin B. This indicated that myriocin at 8 MIC was highly effective in inhibiting the growth of Fon.
Figure 8. The minimal inhibitory concentrations of myriocin against Fon (A) and time-kill curves of myriocin with different concentrations against Fon for 24 h (B). Data are expressed as the means ± SD (n = 3).
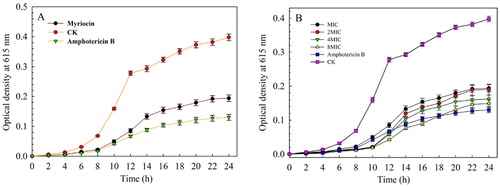
Sphingolipids are major plasma membrane lipid constituents in eukaryotic cells and serine palmitoyl transferase (SPT) is the enzyme that catalyzes the first step of the biosynthesis of all sphingolipids [Citation34]. Myriocin is a SPT inhibitor [Citation35]. Pharmacological inactivation of SPT with myriocin prevented the polarized growth of germinating spores in Aspergillus nidulans [Citation36], myriocin reduced the biofilm biomass and led to the presence of aberrant hyphal structures in Aspergillus fumigatus [Citation37]. In this study, myriocin can inhibit the growth of Fon. However, its mode of action is the subject of ongoing research. Sphingofungins act as new antifungal natural products by inhibiting serine palmitoyl transferase and their structures, especially the structure of sphingofungin E, are strikingly similar to myriocin [Citation38]. Some antimicrobial compounds in Bacillus species played a major role in the biological control of fungal pathogens [Citation39,Citation40] and had synergistic effects [Citation41]. It could be speculated that some antifungal compounds in the CFS, including myriocin, sphingofungin E, sphingofungin F and sphingofungin C, may play a major role in inhibiting the growth of Fon and the synergistic effects among the antifungal compounds was important for this action.
Conclusion
In conclusion, the results clearly demonstrated that the CFS from B. amyloliquefaciens LZN01 in the death phase showed stronger antifungal activity against Fon compared to those from the exponential stage and stationary phase. Antifungal compounds in the CFS could cause damage to the Fon cell membrane and lead to cell death. Myriocin, sphingofungin E, sphingofungin F, 3-methyl-2-oxovaleric acid, gabapentin and sphingofungin C were major differential metabolites. Myriocin content in the CFS was 0.6 μg/mL and its MIC was1.25 μg/mL. The synergistic effects among the antifungal compounds could be important to inhibit Fon. Overall, the results indicated that B. amyloliquefaciens LZN01 is a promising biological agent for the control of Fusarium wilt in watermelon.
Abbreviations | ||
Fon | = | Fusarium oxysporum f. sp. Niveum |
CFS | = | cell-free supernatant |
LB | = | Luria-Bertani medium |
PDA | = | potato-dextrose-agar medium |
SEM | = | scanning electron microscopy |
TEM | = | transmission electron microscopy |
MIC | = | minimum inhibitory concentration |
Supplemental Material
Download PDF (516.1 KB)Supplemental Material
Download PDF (661 KB)Disclosure statement
No potential conflict of interest was reported by the authors.
Additional information
Funding
References
- Fall LA, Clevenger J, McGregor C. Assay development and marker validation for marker assisted selection of Fusarium oxysporum f. sp. niveum race 1 in watermelon. Mol Breed. 2018;38:130.
- Branham SE, Wechter WP, Lambel S, et al. QTL-seq and marker development for resistance to Fusarium oxysporum f. sp. niveum race 1 in cultivated watermelon. Mol Breed. 2018;38:139.
- Mundt CC. Durable resistance: a key to sustainable management of pathogens and pests. Infect Genet Evol. 2014;27:446–455.
- Nawaz MA, Shireen F, Huang Y, et al. Perspectives of vegetable grafting in Pakistan, current status, challenges and opportunities. IJAB. 2017;19:1165–1174.
- Zhao X, Liu Q, Sanchez MT, et al. Performance of grafted seedless watermelon plants with and without root excision under inoculation with Fusarium oxysporum f. sp. niveum race 2. Hortscience 2018;53:1340–1346.
- Lv HF, Cao HS, Nawaz MA, et al. Wheat intercropping enhances the resistance of watermelon to Fusarium wilt. Front Plant Sci. 2018;9:696.
- Raza W, Faheem M, Yousaf S, et al. Volatile and non-volatile antifungal compounds produced by Trichoderma harzianum SQR-T037suppressed the growth of Fusarium oxysporum f. sp. niveum. Sci Lett. 2013; 1:21–24.
- Faheem M, Raza W, Zhong W, et al. Evaluation of the biocontrol potential of Streptomyces goshikiensis YCXU against Fusarium oxysporum f. sp. niveum. Biol Control. 2015;81:101–110.
- Chenniappan C, Narayanasamy M, Daniel GM, et al. Biocontrol effciency of native plant growth promoting rhizobacteria against rhizome rot disease of turmeric. Biol Control. 2019;129:55–64.
- Raza W, Yuan J, Ling N, et al. Production of volatile organic compounds by an antagonistic strain Paenibacillus polymyxa WR-2 in the presence of root exudates and organic fertilizer and their antifungal activity against Fusarium oxysporum f. sp. niveum. Biol Control. 2015;80:89–95.
- Ben Abdallah D, Tounsi S, Frikha-Gargouri O. Inoculum type affect the efficacy of the endophytic Bacillus amyloliquefaciens subsp. plantarum strain 32a against the plant pathogen Agrobacterium tumefaciens. Appl Soil Ecol. 2019;134:25–30.
- Shcherbakova LA, Odintsova TI, Stakheev AA, et al. Identification of a novel small cysteine-rich protein in the fraction from the biocontrol Fusarium oxysporum strain CS-20 that mitigates Fusarium wilt symptoms and triggers defense responses in tomato. Front Plant Sci. 2016;6:1207.
- Getha K, Vikineswary S. Antagonistic effects of Streptomyces violaceusniger strain G10 on Fusarium oxysporum f. sp. cubense race 4: indirect evidence for the role of antibiosis in the antagonistic process. J Ind Microbiol Biotechnol. 2002;28:303–310.
- Zhao J, Xue QH, Niu GG, et al. Extracellular enzyme production and fungal mycelia degradation of antagonistic Streptomyces induced by fungal mycelia preparation of cucurbit plant pathogens. Ann Microbiol. 2013;63:809–812.
- Nikolić I, Berić T, Dimkić I, et al. Biological control of Pseudomonas syringae pv. aptata on sugar beet with Bacillus pumilus SS-10.7 and Bacillus amyloliquefaciens (SS-12.6 and SS-38.4) strains. J Appl Microbiol. 2019; 126:165–176.
- Sarwar A, Brader G, Corretto E, et al. Qualitative analysis of biosurfactants from Bacillus species exhibiting antifungal activity. PLoS One 2018;13:e0198107. [cited 2019 May 24][15 p.].
- Wu YC, Zhou JY, Li CG, et al. Antifungal and plant growth promotion activity of volatile organic compounds produced by Bacillus amyloliquefaciens. Microbiol Open. 2019;e813. DOI:10.1002/mbo3.813
- Xu WH, Wang ZG, Wu FZ. The effect of D123 wheat as a companion crop on soil enzyme activities, microbial biomass and microbial communities in the rhizosphere of watermelon. Front Microbiol. 2015;6:899.
- Takahashi H, Kai K, Shinbo Y, et al. Metabolomics approach for determining growth-specific metabolites based on Fourier transform ion cyclotron resonance mass spectrometry. Anal Bioanal Chem. 2008;391:2769–2782.
- Vidoudez C, Pohnert G. Comparative metabolomics ofthe diatom Skeletonema marinoi in different growth phases. Metabolomics 2012;8:654–669.
- Lee SB, Rhee YK, Gu EJ, et al. Mass-based metabolomic analysis of Lactobacillus sakei and its growth media at different growth phases. J Microbiol Biotechnol. 2017;27:925–932.
- Wang ZG, Wang CL, You YM, et al. Response of Pseudomonas fluorescens to dimethyl phthalate. Ecotoxicol Environ Saf. 2019;167:36–43.
- Xu WH, Liu D, Wu FZ, et al. Root exudates of wheat are involved in suppression of Fusarium wilt in watermelon in watermelon-wheat companion cropping. Eur J Plant Pathol. 2015;141:209–216.
- Xu WH, Wang ZG, Wu FZ. Companion cropping with wheat increases resistance to Fusarium wilt in watermelon and the roles of root exudates in watermelon root growth. Physiol Mol Plant Pathol. 2015; 90:12–20.
- Sun Y, Dong WB, Sun L, et al. Insights into the membrane interaction mechanism and antibacterial properties of chensinin-1b. Biomaterials 2015;37:299–311.
- Wadsworth JM, Clarke DJ, McMahon ST, et al. The chemical basis of serine palmitoyltransferase inhibition by myriocin. J Am Chem Soc. 2013;135:14276–14285.
- Tudela JLR, Barchiesi F, Bille J, et al. Method for the determination of minimum inhibitory concentration (MIC) by broth dilution of fermentative yeasts. Clin Microbiol Infect. 2003;9:1–8.
- Li LR, Shi YH, Cheng XR, et al. A cell-penetrating peptide analogue, P7, exerts antimicrobial activity against Escherichia coli ATCC25922 via penetrating cell membrane and targeting intracellular DNA. Food Chem. 2015;166:231–239.
- Gumbo JR, Cloete TE, van Zyl GJJ, et al. The viability assessment of Microcystis aeruginosa cells after co-culturing with Bacillus mycoides B16 using flow cytometry. Phys Chem Earth 2014;72–75:24–33.
- Kim SG, Zakaullah K, Jeon YH, et al. Inhibitory effect of Paenibacillus polymyxa GBR-462 on Phytophthora capsici causing phytophthora blight in chili pepper. J Phytopathol. 2009;157:329–337.
- Demirbaş F, İspirli H, Kurnaz AA, et al. Antimicrobial and functional properties of lactic acid bacteria isolated from sourdoughs. LWT Food Sci. Technol. 2017;79:361–366.
- Kluepfel D, Bagli J, Baker H, et al. Myriocin, a new antifungal antibiotic from Myriococcum albomyces. J Antibiot. 1972;25:109–115.
- Pereira CB, de Oliveira DM, Hughes AFS, et al. Endophytic fungal compounds active against Cryptococcus neoformans and C. gattii. J Antibiot. 2015;68:436–444.
- Yamaji-Hasegawa A, Takahashi A, Tetsuka Y, et al. Fungal metabolite sulfamisterin suppresses sphingolipid synthesis through inhibition of serine palmitoyl transferase. Biochemistry 2005;44:268–277.
- Castro EV, Yoneyama KG, Haapalainen EF, et al. Myriocin, a serine palmitoyl transferase inhibitor, blocks cytokinesis in Leishmania (Viannia) braziliensis promastigotes. J Eukaryot Microbiol. 2013;60:377–387.
- Cheng J, Park TS, Fischl AS, et al. Cell cycle progression and cell polarity require sphingolipid biosynthesis in Aspergillus nidulans. Mol Cell Biol. 2001;21:6198–6209.
- Perdoni F, Signorelli P, Cirasola D, et al. Antifungal activity of myriocin on clinically relevant Aspergillus fumigatus strains producing biofilm. BMC Microbiol. 2015;15:248.
- Nakamura T, Shiozaki M. Total synthesis of sphingofungin E from D-glucose derivative. Tetrahedron 2002; 58:8779–8791.
- Hu LB, Shi ZQ, Zhang T, et al. Fengycin antibiotics isolated from B-FS01 culture inhibit the growth of Fusarium moniliforme Sheldon ATCC 38932. FEMS Microbiol Lett. 2007;272:91–98.
- Álvarez F, Castro M, Principe A, et al. The plant-associated Bacillus amyloliquefaciens strains MEP218 and ARP23 capable of producing the cyclic lipopeptides iturin or surfactin and fengycin are effective in biocontrol of sclerotinia stem rot disease. J Appl Microbiol. 2012;112:159–174.
- Tao Y, Bie XM, Lv FX, et al. Antifungal activity and mechanism of fengycin in the presence and absence of commercial surfactin against Rhizopus stolonifer. J Microbiol. 2011;49:146–150.