Abstract
Locusta migratoria is one of the global destructive migratory agricultural pests. The species serves as a model insect for the study of morphology, physiology, developmental biology, and neurosciences and so on. In recent years, there has been growing interest in the research of the cell and molecular biology of L. migratoria, and a lot of genes of signalling pathways have been cloned. The Hedgehog (Hh) signalling pathway is essential for cellular proliferation and differentiation during embryonic development. Fused (Fu) is a conserved component of the Hh signalling pathway, and it plays a central role in embryonic development. The Fu gene was cloned from the grasshopper L. migratoria. The gene encodes a 717 amino acid protein. Then, quantitative reverse transcription polymerase chain reaction (qRT-PCR) was used to detect the expression pattern of the Lm-Fu gene at different development stages and tissues. The result showed that the gene was upregulated at the embryonic period and second instar, and the expression level was the highest in the head at the different tissues. Western blotting was used to assess the abundance of the Lm-Fu protein, which was expressed at different development stages. The embryogenesis of L. migratoria was examined by immunohistochemical staining, and the Lm-Fu protein was detected in the head, prothorax and legs. The results showed that the Lm-Fu gene plays an important role during embryonic development.
Introduction
Hedgehog (Hh) signalling plays a highly conserved role in the embryonic development and postnatal life of multicellular organisms ranging from invertebrates to vertebrates. During embryonic development, Hh signalling contributes to cell differentiation, proliferation and survival [Citation1–10], and in postnatal life, in the maintenance of tissue homeostasis [Citation11–13]. In the human body, activation of the Hh pathway results in tumorigenesis, malignancy, and metastasis. This is one of the most widely studied signalling pathways [Citation14,Citation15]. According to research reports, the Hh signalling pathway regulates the formation of many organs during the embryonic stage and determines the direction of cell differentiation; it plays a vital and universal role in cell growth and morphology in the region of Drosophila wing buds [Citation16–20]. Hh is an important signalling pathway regulating the development of wings, legs and primordia; it is also involved in the regulation of other biological processes, such as the migration of germ cells, the development of the lobes, the gonads and the intestines [Citation21–27].
Most of what is known about the Hh signalling cascade comes from studies of Drosophila, where the pathway was originally identified [Citation28]. The Hh signalling pathway is driven by the ligand Hh and transduces signals through binding to its receptor, a 12-transmembrane protein Patched (Ptc). The binding of Hh to Ptc inhibits its repression of Smoothened (Smo), a GPCR-like seven-transmembrane protein [Citation29–36]. Activated Smo functions as the Hh signal transducer to recruit an intracellular protein complex containing the kinesin-like protein Cos2 and the kinase Fused (Fu), leading to Fu phosphorylation and activation [Citation37–45]. In Drosophila, this phosphorylation is sequential and triggered by several kinases that include protein kinase A(PKA;PKA-C1-FlyBase), the serine-threonine kinase Fused (Fu) and G protein-coupled receptor kinase 2 (Gprk2), which promote the conversion of Smo to an open conformation [Citation46–56]. The Ser/Thr kinase Fused (Fu), a known downstream effector of Smo, is part of the Hh signalling pathway, which is one of the most conserved components and may play a central role [Citation57]. The wing imaginal disc model in Drosophila has revealed that phosphorylation at the Hh signalling pathway is induced by the kinase Fused (Fu); in turn, activation of Smo induces Fu to act on its downstream targets [Citation29]. It has been reported that the activity of Fu kinase activates the anterior compartment cells of the wing disc and induces the expression of related genes, thereby affecting the development of the wing. In Drosophila, a biochemical study established that Fu plays a vital role in the embryonic development [Citation58,Citation59].
Fu has been cloned from Drosophila melanogaster (NM_058151.5) and Bombyx mori (XM_004923160.3). However, the structure and function of the Fu protein remains unclear in Locusta. migratoria, which has long served as a model organism for many aspects research, such as insect morphology, behaviour and physiology [Citation60–63]. This insect is also one of the most destructive agricultural pests. In China, outbreaks of L. migratoria have been increasing in recent decades, which are associated with locust’s long-distance migration and resistance to pesticides [Citation64,Citation65]. Studying the physiological characteristics of grasshoppers is important for the control of L. migratoria. In this paper, the cDNA of the Lm-Fu gene was obtained from L.migratoria. The expression levels of Lm-Fu at different developmental stages and adult tissues were investigated. In addition, the protein expression and localization of Fu were analyzed by Western blotting and immunohistochemistry. Our aim was to understand the function of Lm-Fu in L.migratoria.
Materials and methods
Animals and reagents
Eggs of L.migratoria were purchased from Junan, Shandong Province. The L. migratoria eggs were held in a constant temperature incubator at 30 °C and 75% relative humidity with an L12:D12 photoperiod. When the eggs hatched, wheat seedlings were given as food. Nymphal instars were identified by the development of wings. Appropriate amounts of L.migratoria from each instar were taken and rapidly frozen in liquid nitrogen and then stored at –80 °C. All the restriction enzymes, T4 DNA ligases and Taq DNA polymerase were from TaKaRa Biotechnology (Dalian, China).
Cloning of the Fu gene
Total RNA was extracted from adult L.migratoria using RNAiso Plus (TaKaRa, Dalian, China), and then was transcribed into cDNA. Based on the transcriptomic database from our laboratory of L.migratoria, specific primers (Fu-F, Fu-R, ) were synthesized by Genewiz (China). The amplifications were performed with an initial denaturation at 94 °C for 3 min, followed by 35 cycles of denaturation (94 °C, 30 s), annealing (55 °C, 30 s), extension (72 °C, 1 min), and followed by a 10 min of extension at 72 °C. The PCR amplification products were detected using 1.0% agarose gel by electrophoresis. The objective bands were purified by a DNA gel extraction kit (Takara, Dalian, China), and then sequenced (TSINGKE, China).
Table 1. Primers used in this study.
Bioinformatics analysis
The sequence of Lm-Fu was analyzed using BLAST program at the National Center for Biotechnology Information (NCBI, US National Institute of Health) website (http://www.ncbi.nlm.nih.gov/BLAST). SignalP 4.1 program (http://www.cbs.dtu.dk/services/SignalP) and TMHMM2.0 (http://www.cbs.dtu.dk/services/TMHMM/) were used to predict the signal peptide of the Lm-Fu amino acid sequences and transmembrane region. ClustalX2.0 and DNAman were used to carry out multiple alignment analysis. Then, a phylogenetic tree of the insect system was constructed using the neighbour-joining (NJ) method and MEGA6.0 software.
Quantitative real-time polymerase chain reaction (PCR)
RNA samples of L.migratoria were extracted from different development instars of nymphae (the first, second, third, fourth and late fifth instars) and different tissues of adult females and males (prothorax, abdomen, legs, wing, head, midgut). Total RNA was extracted by the Trizol purification method. After that, the extracted mRNA was reverse transcribed into cDNA using a PrimeScript® RT Reagent Kit for Perfect Real Time (Takara, Dalian, China). According to the full-length DNA of Lm-Fu, the qRT-PCR primer pairs (qFu-F, qFu-R, ) were designed in Primer Premier 5.0 (qFu-F, qFu-R, ). The normalization control gene was the β-actin housekeeping gene (β-actinF, β-actinR, ) Real-time qPCR was performed in LightCycler 96 System(Roche, Potsdam, Germany). Each 10 µL PCR reaction mixture contained: 5 µL SYBR Premix Ex Taq (Roche, Potsdam, Germany), 0.4 mL of each primer (10 mmol/L), 1 µL cDNA solutions, and 3.2 mL sterile distilled water. PCR conditions were: an initial denaturation at 95 °Cfor 30 s; followed by 35 cycles of 95 °C for 10 s, 53 °C for 30 s and 72 °C for 15 s. Each experiment was repeated at least three times. Finally, we used the delta-delta Ct method to calculate the relative quantitative expression [Citation66].
Prokaryotic expression
After obtaining the complete sequence of the Lm-Fu gene, primers exFu-F and exFu-R () with restriction enzyme sites (KpnI and EcoRI) were designed. The PCR product and pET-30a were digested with the restriction enzymes. After the two fragments were ligated, the recombinant pET-30a-Fu-plasmid was transferred into E. coli DH5α via the heat shock method. The insertion fragment was sequenced (Genewiz company; Suzhou, China) to ensure that there were no errors in the sequence and then was transferred into E. coli BL21 (DE3). When the optical density (OD) at 600 nm of the transformed cells increased to 0.6–0.8, IPTG was added for induction. Sonication was performed in an ice bath to disintegrate the cells. The fractions were collected and tested by SDS-PAGE on a 12% gel. Finally, the protein was purified and sent to BGI (Shenzhen, China) for antibody preparation.
Western blot
Total proteins from different instars of L.migratoria were extracted and analyzed by 12% sodium dodecyl sulphate polyacrylamide gel electrophoresis (SDS-PAGE.) Then the proteins were transferred to a nitrocellulose (NC) membrane. To block non-specific protein binding, the membrane was incubated with skimmed milk for 2 hours; then the NC membrane was incubated with Rabbit anti-Fu polyclonal antibodies (1:100) at 4 °C overnight. After washing with TBST (TBS/0.1%Tween 20), the NC membrane was incubated with secondary antibody of goat anti-rabbit IgG-HRP (Proteintech, China) (1:10,000) at room temperature for 2 h. Finally, colour development was performed using an ECL chromogenic kit (Sangon, Shanghai, China). β-actin was used as an internal reference.
Immunohistochemistry
Different periods eggs of L.migratoria were collected, soaked with 10% NaClO for 15 minutes, then the embryos were dissected manually in 1× PBS (phosphate buffered saline) by cutting off the anterior pole and squeezing the embryo and yolk out of the egg shell. Subsequently, the eggs were cleaned form yolk and fixed on ice for 30 min in PEM-FA fixative. The embryos were rehydrated stepwise into PBS/0.1% Triton X-100 (PBT), and blocked in PBT/1% BSA for 2 h at room temperature. The treatment embryos were incubated with Rabbit anti-Fu polyclonal antibodies (1:50) at 4 °C overnight. The control embryos were incubated with negative serum. After washing 3 times in PBST, the samples were incubated with secondary antibody of goat anti-rabbit IgG-HRP (1:10000) at room temperature for 1 h, after further washing with PBST. After incubating for 12 minutes using the DAB kit (Sangon, Shanghai, China), the samples were observed.
Data analysis
Data are mean values with standard deviation (±SD) of three independent replicates. Student’s t test and Fish’s LSD multiple comparison test were used for assessment of significant differences among the developmental stages and different tissues.
Results and discussion
Cloning and sequence analysis of the Lm-Fu gene
A 4783-bp cDNA of the Lm-Fu gene was obtained from the grasshopper L. migratoria by RT-PCR, and the gene was named Lm-Fu (GenBank Accession No. MH704853). The sequence contained an open reading frame of 2154 bp, and was predicted to encode 717 amino acids. The theoretical amino acid sequence of Lm-Fu was analyzed and predicted by the CD search program of NCBI. This result is consistent with the report for Drosophila [Citation67] (). The results of the multiple alignments showed a high degree of homology to the Lm-Fu amino acid sequence. Moreover, Standard protein BLAST (blastp) also confirmed that the Lm-Fu amino acid sequence and the amino acid sequence of Neodoprion lecontei shared 50% homology (). The analysis of the phylogenetic tree constructed by the NJ method showed that all sequences from the same group were clustered together. The homology between the Lm-Fu protein of L. migratoria and the Hymenoptera protein situated them more closely, and the confidence level reached 93%. The mammals were the outgroup of the phylogenetic tree ().
Figure 2. Multiple alignment of Lm-Fu amino acid sequences with homologous sequences of other species. Origin species of Fu proteins and their GenBank accession numbers: Neodiprion lecontei, XP_015516766; Camponotus floridanus, XP_011253786; Polistes canadensis, XP_014611904; Leptinotarsa decemlineata, XP_023021946.1; Agrilus planipennis, XP_018334476.1; Pogonomyrmex barbatus, XP_011631863; Bicyclus anynana, XP_023948396.1; Onthophagus taurus, XP_022902513; Aedes albopictus, XP_019535697; Aedes aegypti, XP_001658684.2; Helicoverpa armigera, XP_021189625; Papilio polytes, XP_013146380.1; Locusta migratoria, MH704853; Consensus: Shared sequence. The common amino acids are indicated with black highlight.
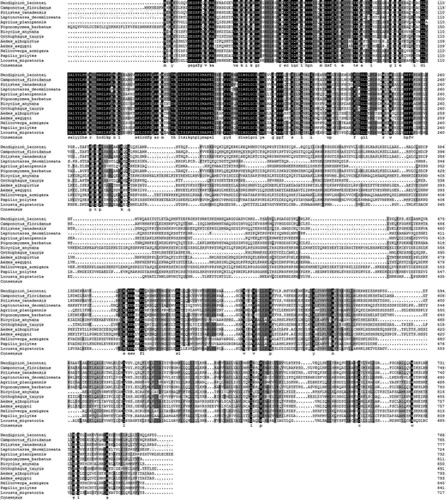
Expression analysis of Lm-Fu at different stages and different tissues
qRT-PCR was used to analyze the expression levels of the Lm-Fu gene at different developmental stages and in different tissues. The results showed that the Lm-Fu gene was expressed in the different tissues and developmental stages. The expression levels of the Lm-Fu gene in the second instar were higher than those in other instars. At the second instar, the wing primordium of the L. migratoria begins to develop. Fu protein plays a role in the formation of wing imaginal discs. Fused kinase activity is required at the A/P boundary in the anterior compartment of the wing disc [Citation58]. The fu mutant wings present alterations in the region of the wing blade between veins 3 and 4 and thus are affected in anterior and posterior compartments on both sides of the A/P boundary in D. melanogaster [Citation58]. In the fly wing imaginal disc, the protein kinase Fused (FU) plays a key role in the responses to high levels of Hh [Citation58]. In our study, the transcripts of the Lm-Fu gene were at lower levels in the third and fourth instar. Among them, the expression of Lm-Fu reached the lowest levels in the fourth instar ().
Figure 4. Expression analysis of the Lm-Fu gene at different developmental stages of L. migratoria. 1: embryonic period; 2: first instar; 3: second instar; 4: third instar; 5: forth instar; 6: fifth instar. Data are means with standard deviation (± SD) of triplicate experiments. Asterisks indicate signicant differences compared to the control: *p < .05; **p < .01.
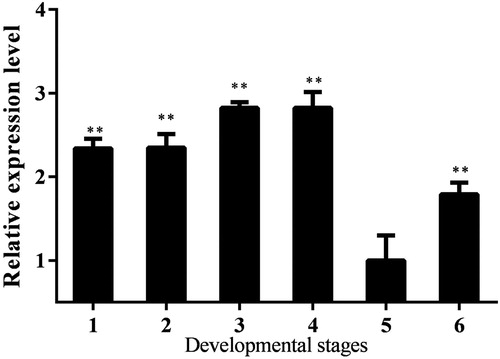
In order to better study the function of Lm-Fu in embryonic development, we observed and analyzed the expression levels of Lm-Fu in different periods of embryogenesis. The relative expression level was higher during the embryonic period, which is in agreement with the important role that the Ser/Thr kinase Fused (Fu) has in the Hh signalling pathway during the embryonic development [Citation68,Citation69]. The expression level was the highest on the 8th day of the embryonic period. The reason could be that the Lm-Fu gene promotes development in the nervous system. The nervous system development begins on the 8th day of the embryonic period. In the developing Drosophila eye, Hh controls the initiation and propagation of the morphogenetic furrow, leading to photoreceptor differentiation. Hh signalling is important during Drosophila nervous system development [Citation70]. Fused is involved in the development of various organs; for example, it is expressed in the central nervous system during development [Citation71,Citation72]. In our study, the expression level of the Lm-Fu gene reached the lowest on the 14th day of the embryonic period ().
Figure 5. Expression analysis of the Lm-Fu gene at different developmental stages in the embryonic period of L. migratoria. Data are means ± SD of triplicate experiments. Asterisks indicate signicant differences compared to the control: *p < .05; ** p < .01.
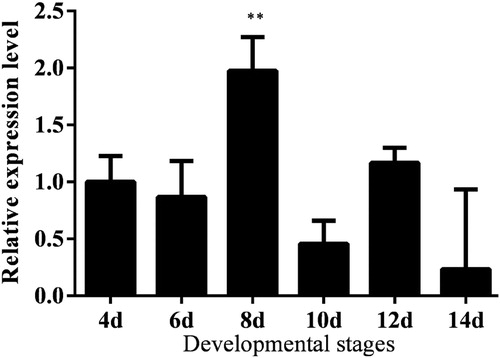
As shown in , the Lm-Fu gene was expressed at different degrees in different tissues of male and female adults. In all tissues, the expression was higher in males than in females. The reason could be the effect of estrogen on the Lm-Fu gene [Citation73]; however, the mechanism is not yet clear. The expression level of the Lm-Fu gene in different tissues of the female was low. The Lm-Fu gene had the lowest expression level in the male and female midgut, and it reached higher levels in the male and female head.
SDS-PAGE analysis of Lm-Fu and Western blot
The partial sequences were selected for prokaryotic expression. The recombinant cells were disintegrated by sonication. The results of SDS-PAGE showed that the target protein was expressed in inclusion bodies, and a protein band of 48 kD was obtained. After purification and concentration, the target protein of relative purity was obtained ().
Figure 7. SDS-PAGE (A) and Western blot (B) analysis of Lm-Fu. Note: (A) SDS-PAGE analysis: M, protein Marker (TransGen Biotech, Beijing); Lane 1, recombinant product of pET-30a-Lm-Fu without inducer; Lane 2, recombinant product with inducer; Lane 3, supernatant of cell lysate; Lane 4, precipitate fraction; Lane 5, purified recombinant protein. (B) Protein expression analysis of Lm-Fu at different stages.
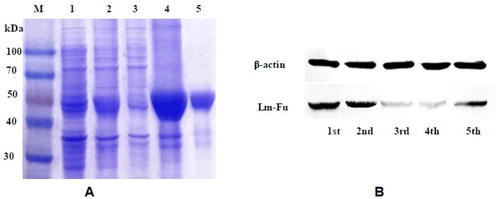
We detected the expression of Lm-Fu by western blot. Experiments were carried out by selecting different days of L. migratoria from each instar nymphs. As shown in , Lm-Fu had the highest expression in the second instar, and relatively low expression in the third and fourth instars, reaching the lowest level at the fourth instar. The western blot results were consistent with qRT-PCR.
Immunohistochemistry analysis of Lm-Fu prorein
Chin et al. [Citation74] divided the embryonic development of L. migratoria into 18 stages. In different stages, embryos express different forms. Immunohistochemistry was used to detect the location of Lm-Fu protein of L. migratoria in different developmental stages of the embryo (). During the E-6 period, compared to the control group (1), Lm-Fu was expressed in the head, but the expression was relatively small (2). At E-8 period, there was a groove in the middle of the hind leg bud to divide the hind leg into the femur and tibia. It could be observed that Lm-Fu was expressed in the upper mandible and the antennae, but the effect was not obvious (2). At E-10 period, Lm-Fu was expressed in the head, as well as in the fore leg and middle leg, and the effect was obvious (, E10A2). At E-12 period, a red-brown cresent-shaped compound eye began to appear. There was transposition in the fore leg, middle leg and hind leg., and Lm-Fu was detected in the head and legs (, E12A2). At E-14 period, the segmentation of the legs was clearly visible and the expression of Lm-Fu was increased (, E14A2). At E-16 period, the herringbone pattern on the femur leg was clearly visible, the black cresent-shaped femur appeared at the tarsus, and Lm-Fu was distributed in the head, prothorax and legs (, E16A2). The immunohistochemistry results showed that Lm-Fu prorein was located in the head appendages and legs segments in the embryo development, which is consistent with the previous report that Fu prorein exhibits constitutive, non-specific expression [Citation70]. There are research reports that the Hh signalling pathway plays a critical role in determining proper embryonic patterning and cell fate determination in the central nervous system [Citation71]. Since the Ser/Thr kinase Fused (Fu) is a positive regulator of the Hh signalling pathway during the embryonic development [Citation68,Citation69], we hypothesize that Lm-Fu prorein plays an important role in the development of the embryo, especially in the early development of the embryonic head appendages and legs segments.
Figure 8. Immunohistochemistry of L. migratoria in different developmental stages. A1, control group; A2, experimental group. E6, sixth stage of embryonic development; E8, eighth stage of embryonic development; E10, tenth stage of embryonic development; E12, twelfth stage of embryonic development; E14, fourteenth stage of embryonic development; E16, sixteenth stage of embryonic development.
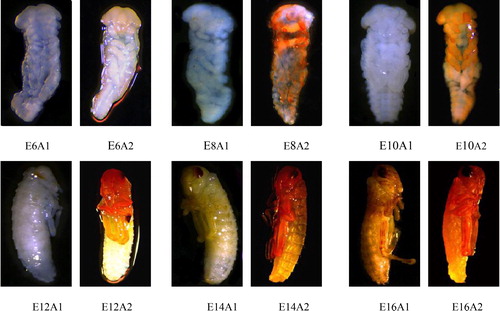
Conclusions
In this study, the sequence of Lm-Fu from L. migratoria was cloned, and sequence alignment was performed. The analysis of the expression patterns of the Lm-Fu gene by qRT-PCR showed that the Lm-Fu gene was expressed in different developmental stages and different tissues at different levels. The expression of the Lm-Fu gene was the highest in the second instar. The expression of the Lm-Fu gene in males was higher than that in females. The results of qRT-PCR and immunohistochemistry showed that Lm-Fu played an important role in embryonic development. The research on the Lm-Fu gene of L. migratoria established a theoretical foundation for exploring new approaches to the control of locust plagues.
Disclosure statement
No potential conflict of interest was reported by the authors.
Additional information
Funding
References
- Jiang YJ, Zhang SX, Mao CQ. Defining a critical period in calvarial development for Hedgehog pathway antagonist-induced frontal bone dysplasia in mice. Int J Oral Sci. 2019;11:3.
- Byrne EF, Luchetti G, Rohat Gi R. Multiple ligand binding sites regulate the Hedgehog signal transducer Smoothened in vertebrates. Curr Opin Cell Biol. 2018;51:81–88.
- Briscoe J, Thérond, Pascal P. The mechanisms of Hedgehog signalling and its roles in development and disease. Nat Rev Mol Cell Biol. 2013;14:418–431.
- Ingham PW, Nakano Y, Seger C. Mechanisms and functions of Hedgehog signalling across the metazoa. Nat Rev Genet. 2011;12:393–406.
- Varjosalo M, Taipale J. Hedgehog: functions and mechanisms. Genes Dev. 2008;22:2454–2472.
- Giordano C, Ruel L, Poux C, et al. Protein association changes in the Hedgehog signaling complex mediate differential signaling strength. Development. 2018;145.
- Liu C, Zhou Z, Yao X, et al. Hedgehog signaling downregulates Suppressor of Fused through the HIB/SPOP-Crn axis in Drosophila. Cell Res. 2014;24:595–609.
- Huang DL, Wang YT, Tang JB, et al. Molecular mechanisms of suppressor of fused in regulating the hedgehog signalling pathway. Oncol Lett. 2018;15:6077–6086.
- Lum L, Beachy PA. The Hedgehog response network: Sensors, switches, and routers. Science. 2004;304:1755–1759.
- Zhang YH, Zhang YJ. Forkhead box C2 promotes the invasion ability of human trophoblast cells through Hedgehog (Hh) signaling pathway. Cell Biol Int. 2018;42:859–866.
- McMahon AP, Ingham PW, Tabin CJ. Developmental roles and clinical significance of Hedgehog signaling. Curr Top Dev Biol. 2003;53:1–114.
- Huangfu D, Anderson KV. Signaling from Smo to Ci/Gli: conservation and divergence of Hedgehog pathways from Drosophila to vertebrates. Development. 2006;133:3–14.
- Hooper JE, Scott MP. Communicating with Hedgehogs. Nat Rev Mol Cell Biol. 2005;6:306–317.
- Rubin LL, De Sauvage FJ. Targeting the Hedgehog pathway in cancer. Nat Rev Drug Discov. 2006;5:1026–1033.
- Jitender B, Virender K, Dong YX, et al. Design of Hedgehog pathway inhibitors for cancer treatment. Med Res Rev. 2019;39:1137–1204.
- Fuccillo M, Joyner AL, Fishell GM. orphogen to mitogen: the multiple roles of hedgehog signaling in vertebraten neural development. Nat Rev Neurosci. 2006;7:772–783.
- Allen EB, Kuan-Ping Y. The hedgehog pathway and basal cell carcinomas. Hum Mol Genet. 2001;10:757–762.
- George H, Christian von M, Konrad B. The Hedgehog signaling pathway: where did it come from. Plos Biol. 2009;7:1–6.
- Casso DJ, Biehs B, Kornberg TB. A novel interaction between Hedgehog and Notch promotes proliferation at the anterior-posterior organizer of the Drosophila wing. Genetics. 2011;187:485–499.
- Christiansen AE, Tian D, Bergmann A. Ligand-independent activation of the Hedgehog pathway displays non-cell autonomous proliferation during eye development in Drosophila. Mech Develop. 2012;129:98–108.
- Tabata T, Kornberg TB. Hedgehog is a signaling protein with a key role in patterning Drosophila imaginal discs. Cell. 1994;76:89–102.
- M Ohler J. Requirements for hedgehog, a segmental polarity gene, in patterning larval and adult cuticle of Drosophila. Genetics. 1988;120:1061–1072.
- Easier K, Struhl G. Compartment boundaries and the control of Drosophila limb pattern by hedgehog protein. Nature. 1994;368:208–208.
- Diaz-Benjumea FJ, Cohen B, Cohen S. Cell interaction between compartments establishes the proximal-distal axis of Drosophila legs. Nature (London). 1994;372:175–179.
- Dominguez M. Dual role for Hedgehog in the regulation of the proneural gene atonal during ommatidia development. Development. 1999;126:2345–2353.
- Zhang Y, Kalderon D. Regulation of cell proliferation and p atterning in Drosophila oogenesis by Hedgehog signaling. Development. 2000;127:2165–2176.
- Duman-Scheel M, Weng L, Xin S, et al. Hedgehog regulates cell growth and proliferation by inducing Cyclin D and Cyclin E. Nature. 2002;417:299–304.
- Nusslein VC. M utation affecting segment number and polarity in Drosophila. Nature. 1980;287:795–801.
- Sanial M, Bécam I, Hofmann L, et al. Dose dependent transduction of Hedgehog relies on phosphorylation-based feedback between the GPCR Smoothened and the kinase Fused. Development. 2017;144:1841–1850.
- Zhang J, Liu Y, Jiang K, et al. SUM O regulates the activity of Smoothened and Costal-2 in Drosophila Hedgehog signaling. Sci Rep. 2017;7:42749. [cited 2019 Apr 01]
- Casali A, Struhl G. Reading the Hedgehog morphogen gradient by measuring the ratio of bound to unbound Patched protein. Nature. 2004;431:76–80.
- Marigo V, Davey RA, Zuo Y, et al. Biochemical evidence that Patched is the Hedgehog receptor. Nature (London). 1996;384:176–179.
- Stone DM, Hynes M, Armanini M, et al. The tumour-suppressor gene patched encodes a candidate receptor for Sonic hedgehog. Nature. 1996;384:129–134.
- Zhao Y, Tong C, Jiang J. Hedgehog regulates smoothened activity by inducing a conformational switch. Nature. 2007;450:252–258.
- Corbit KC, Aanstad P, Singla V, et al. Vertebrate Smoothened functions at the primary cilium. Nature (London). 2005;437:1018–1021.
- Rohatgi R, Milenkovic L, Scott MP. Patched1 regulates hedgehog signaling at the primary cilium. Science. 2007;317:372–376.
- Kuzhandaivel A, Schultz S, Alkhori L, et al. Cilia-mediated hedgehog signaling in Drosophila . Cell Rep. 2014;7:672–680.
- Marcel VDH, Ingham PW. Smoothened encodes a receptor-like serpentine protein required for hedgehog signalling. Nature (London). 1996;382:547–551.
- Denef N, Dagmar N, Perez L, et al. Hedgehog induces opposite changes in turnover and subcellular localization of patched and smoothened. Cell. 2000;102:521–531.
- Alcedo J, Ayzenzon M, Ohlen TV, et al. The Drosophila smoothened gene encodes a seven-pass membrane protein, a putative receptor for the hedgehog signal. Cell. 1996;86:221–232.
- Lum L, Zhang C, Oh S, et al. Hedgehog signal transduction via smoothened association with a cytoplasmic complex scaffolded by the atypical Kinesin, Costal-2. Mol Cell. 2003;12:1261–1274.
- Jia J. Smoothened transduces Hedgehog signal by physically interacting with Costal2/Fused complex through its C-terminal tail. Gene Dev. 2003;17:2709–2720.
- Zhang Y, Mao F, Lu Y, et al. Transduction of the Hedgehog signal through the dimerization of Fused and the nuclear translocation of Cubitus interruptus. Cell Res. 2011;21:1436–1451.
- Zhou Q, Kalderon D. Hedgehog activates fused through phosphorylation to elicit a full spectrum of pathway responses. Dev Cell. 2011;20:802–814.
- Ruel L, Rodriguez R, Gallet A, et al. Stability and association of smoothened, Costal2 and fused with Cubitus interruptus are regulated by Hedgehog. Nat Cell Biol. 2003;5:907–913.
- Zhang C, Williams EH, Guo Y, et al. Extensive phosphorylation of Smoothened in Hedgehog pathway activation. Proc Natl Acad Sci USA. 2004;101:17900–17907.
- Jia J, Tong C, Wang B, et al. Hedgehog signalling activity of Smoothened requires phosphorylation by protein kinase A and casein kinase I. Nature. 2004;432:1045–1050.
- Apionishev S, Katanayeva NM, Marks SA, et al. Drosophila Smoothened phosphorylation sites essential for Hedgehog signal transduction. Nat Cell Biol. 2005;7:86–92.
- Zhou Q, Apionishev S, Kalderon D. The contributions of protein Kinase A and smoothened phosphorylation to Hedgehog signal transduction in Drosophila melanogaster. Genetics. 2006;173:2049–2062.
- Jia H, Liu Y, Xia R, et al. Casein kinase 2 promotes Hedgehog signaling by regulating both smoothened and Cubitus interruptus. J Biol Chem. 2010;285:37218–37226.
- Chen Y, Li S, Tong C, et al. G protein-coupled receptor kinase 2 promotes high-level Hedgehog signaling by regulating the active state of Smo through kinase-dependent and kinase- independent mechanisms in Drosophila. Gene Dev. 2010;24:2054–2067.
- Fan J, Liu Y, Jia J. Hh-induced Smoothened conformational switch is mediated by differential phosphorylation at its C-terminal tail in a dose- and position-dependent manner. Dev Biol. 2012;366:172–184.
- Jiang K, Liu Y, Fan J, et al. Hedgehog-regulated atypical PKC promotes phosphorylation and activation of Smoothened and Cubitus interruptus in Drosophila. Proc Natl Acad Sci USA. 2014;111:4842–4850.
- Maier D, Cheng S, Faubert D, et al. A broadly conserved g-protein-coupled receptor kinase phosphorylation mechanism controls Drosophila smoothened activity. PLoS Genet. 2014;10:e1004399. [cited Apr 01]
- Ma G, Li S, Han Y, et al. Regulation of Smoothened trafficking and Hedgehog signaling by the SUMO Pathway. Dev Cell. 2016;39:438–451.
- Ballesterostajadura R, Guerras Colón FI, Velarde Suárez S, et al. Numerical model for the unsteady flow features of a Squirrel Cage Fan. In: ASME 2009 Fluids Engineering Division Summer Meeting; Vail, Colorado, USA, August 2–6, 2009. Proceedings, Volume 2: Fora. American Society of Mechanical Engineers; 2009. p. 173–183.
- Hooper JE, Scott MP. The Drosophila patched gene encodes a putative membrane protein required for segmental patterning. Cell. 1989;59:751–765.
- Alves G, Limbourg BB, Tricoire H, et al. M odulation of Hedgehog target gene expression by the Fused serine-threonine kinase in wing imaginal discs. Mech Dev. 1998;78:17–31.
- Préat T, Thérond P, Lamour-Isnard C, et al. A putative serine/threonine protein kinase encoded by the segment-polarity fused gene of Drosophila. Nature. 1990;347:87–89.
- Wang XH, Fang XD, Yang PC, et al. The locust genome provides insight into swarm formation and long-distance flight. Nat Commun. 2014;5:2957.
- Liu XJ, Sun YW, Li DQ, et al. Identification of LmUAP1 as a 20-hydroxyecdysone response gene in the chitin biosynthesis pathway from the migratory locust, Locusta migratoria. Insect Sci. 2018;25:211–221.
- Wang HS, Wang XH, Zhou CS, et al. cDNA cloning of heat shock proteins and their expression in the two phases of the migratory locust. Insect Mol Biol. 2007;16:207–219.
- Wei YY, Chen S, Yang PC, et al. Characterization and comparative profling of the small RNA transcriptomes in two phases of locust. Genome Biol. 2009;10:R6.
- Ayali A, Yerushalmi Y. Locust research in the age of model organisms: introduction to the special issue in honor of MP Pener’s 80th birthday. J Insect Physiol. 2010;56:831–833.
- Zhao XM, Gou X, Qin ZY, et al. Identification and expression of cuticular protein genes based on Locusta migratoria transcriptome. Sci Rep. 2017;7.
- Therond PP, Knight JD, Kornberg TB, et al. Phosphorylation of the fused protein kinase in response to signaling from hedgehog. Proc Natl Acad Sci USA. 1996;93:4224–4228.
- Livak KJ, Schmittgen TD. Analysis of relative gene expression data using real-time quantitative PCR and the 2(-Delta Delta C(T)) Method. Methods. 2001;25:402–408.
- Forbes AJ, Nakano Y, Taylor AM, et al. Genetic analysis of hedgehog signalling in the Drosophila embryo. Dev Suppl. 1993;11:115–124.
- Skoda AM, Simovic D, Karin V, et al. The role of the Hedgehog signaling pathway in cancer: A comprehensive review. Bosn J of Basic Med Sci. 2018;18:8–20.
- Rangarajan R, Courvoisier H, Gaul U. Dpp and Hedgehog mediate neuron-glia interactions in Drosophila eye development by promoting the proliferation and motility of subretinal glia. Mech Dev. 2001;108:93–103.
- Gulino A, Marcotullio LD, Ferretti E, et al. Hedgehog signaling pathway in neural development and disease. Psychoneuroendocrinology. 2007;32:S52–S56.
- Yesser B, Andrew H, Sangwoo S, et al. The M any Hats of Sonic Hedgehog signaling in nervous system development and disease. J Dev Biol. 2016;4:35–52.
- Fausto SA. On the timing and place of action during embryogenesis of the female-sterile mutants fused and rudimentary Drosophila melanogaster. Dev Biol. 1971;26:452–463.
- Chin CT, Chai CH, Sha CY. Studies on the locut egg I: morphological changes of the embryo during egg incubation, with special reference to the development stages of eggs collected from the field (Acrididae: Orthoptera.). Acta Entomol Sin. 1954;4:383–398.