Abstract
The aims of this study were to investigate different species of Triticum and Aegilops in terms of the expression pattern of ascorbate peroxidase, superoxide dismutase, and guaiacol peroxidase encoding genes along with the wheat dehydrin gene, wdhn13, which is involved in the induction of tolerance to drought stress. The study also explored the enzyme activity of these three antioxidant enzymes under drought stress. There was a significant difference in the expression level of the four studied genes, Wdhn13, APX, SOD and GPX, between the well-watered and drought stress (FC = 100% and FC = 25%) treatments and among the examined species. The relative expression of Wdhn13 under conditions of drought was higher in T. boeoticum and Ae. crassa compared to the tolerant reference cultivar (Sirvan) and the rest of the wild species. Ae. tauschii showed the highest expression of the APX gene, and the SOD and GPX genes were up-regulated in Ae. tauschii and T. boeoticum at the drought-stressed conditions. The highest APX, GPX and SOD enzyme activities under the drought-stressed conditions were observed in Ae. crassa, T. boeoticum and Ae. neglecta, respectively. The results indicated that there was relevant correspondence between the genetically determined tolerance of wheat species (i.e. the gene transcript levels) and their antioxidant enzyme activities. The species having Ab (T. boeoticum), D (Ae. tauschii) and D (Ae. tauschii and Ae.crassa) genomes had a higher expression of GPX, SOD, and APX genes, respectively.
Introduction
Abiotic stresses are one of the most important causes of damage to agricultural crops. The potential yield of any crop depends on the plant vigor during the first stages of development [Citation1]. The seedling stage in many plants is the most susceptible phase to environmental stresses such as water deficit stress [Citation2]. Water deficit is a burden to plant growth and yield in any area of the world. In order to respond to the environmental fluctuations, plants have developed a complex defense system to adapt to stress by various biochemical and physiological regulations, reflected at the level of gene expression [Citation3]. The stress-induced changes in the expression of some genes reveal the genetic basis for the development of such defense responses [Citation4]. The plant cell responses to dehydration comprise the reposition of osmotically active compounds including hydrophilic proteins such as dehydrins [Citation5]. The dehydrins (DHN) are reported to play a basic function in drought stress tolerance [Citation3, Citation6] and to accumulate in different plant tissues at stressed conditions [Citation5]. All of the late embryogenesis abundant (LEA) proteins are defined by high hydrophilicity, high glycine content, and change in protein secondary structure [Citation7]. There are five subclasses of dehydrins depending on their conserved amino acid sequences [Citation6]. The wheat DHN gene, wdhn13, which is located on the group7 homoeologous chromosomes, encodes the smallest class of DHN protein [Citation8].
Plants have employed different defense systems that allow them to adapt to the stress conditions like drought stress for survival [Citation9]. One of the principle plant defensive mechanisms at cell level during abiotic stresses is an increase in the reactive oxygen species (ROS). Different subcellular organelles such as chloroplasts, mitochondria and peroxisomes are the common sources of ROS generation. The accumulated ROS, including singlet oxygen, hydroxyl radical, superoxide and hydrogen peroxide (H2O2), act as the signal molecules in response to the stress [Citation10]. Excessive production of ROS may lead to cellular damage, enzyme inhibition, protein degradation, DNA and RNA damage, and finally cell death [Citation11]. Hence, the ROS detoxification system in cells is necessary for the defense of the plant against the toxic impact of ROS [Citation12]. The ROS detoxification strategies comprise the enzymatic and non-enzymatic antioxidant elements [Citation13]. The enzymatic antioxidants include catalase (CAT), superoxide dismutase (SOD), guaiacol peroxidase (GPX), ascorbate peroxidase (APX) and peroxiredoxin (PrxR). These enzymes are actually present in all sub-cellular organelles. Generally, an organelle has more than one antioxidant enzyme able to eliminate a single type of ROS [Citation13, Citation14]. Therefore, the over-expression of the antioxidant-enzyme–encoding genes could induce tolerance to abiotic stresses. With the recent progress in molecular biology and biotechnology methods, the regulation of gene expression has been proposed as a robust technology to improve the tolerance of plants to various abiotic stresses [Citation15, Citation16].
Genes encoding antioxidant enzymes have been engineered in several plants to attain improved drought tolerance [Citation17]. Surely, manipulation of genes encoding antioxidant enzymes has provided the new vision into the function of these enzymes in plant cells in deactivating stress-created ROS [Citation18]. Subsequently, ROS are not only considered as the cellular signal of stresses but also secondary messenger involved in the stress-response signaling pathways. The higher production of ROS in the peroxisomes and chloroplasts is correlated with the potent changes in the genes expression [Citation19] that disclose the transportation of O2-derived signals from the plastid to the nucleus [Citation20]. It is well-known that the wild relatives of wheat, due to their longtime adaptation to different environments, considerably suffer from various adverse growth conditions. Hence, breeding to drought tolerance in modern wheat may be accomplished by the allelic gene pool of the wild relatives of wheat [Citation21]. The wild relatives and ancestor species of bread wheat have an extensive pool of basic genes and alleles which could be utilized in wheat breeding programs conferring tolerance to biotic and abiotic stresses [Citation22–24]. The species belonging to the Triticeae genus (Aegilops and Triticum) are known as the main wild relatives of wheat containing a feasible source of gene pool for the stress stability; since they are distributed in semi-arid regions and in this way, they are adapted to their environmental stresses.
Understanding the genetic mechanisms underlying the drought tolerance would contribute towards better exploitation of this germplasm in wheat breeding programs. The main objectives of this research were to investigate the effects of water deficit stress on the expression pattern of three antioxidant-enzyme–encoding genes and one wheat dehydrin gene, wdhn13, in the leaves of six wild relatives of wheat along with two drought tolerant and sensitive reference cultivars. The activities of the three antioxidant enzymes, i.e. ascorbate peroxidase, superoxide dismutase and guaiacol peroxidase, and the total protein content were also assayed.
Materials and methods
Plant material and growth conditions
This research was conducted on six species of Aegilops and Triticum grown in a greenhouse along with two reference bread wheat cultivars (T. aestivum cv. Sirvan as tolerant and Darya as sensitive). The information about the genome, ploidy level, and the sampling locations of these species is given in . Ten seeds of each species were planted in pots containing soil-sand mixture (3:1 ratio). All accessions were grown in a greenhouse (25:20 °C day/night and 16:8 h light/dark period). The plants were watered every 2-3 days to maintain 100% field capacity (FC). The field capacity was determined by the method of Souza et al. [Citation25]. The experiment was carried out as a factorial based on a randomized complete block design with four replicates. The water stress treatment started at the three-leaf stage of the seedling growth. At this point, the plants were subjected to two water regimes: Non-stress (100% FC) and drought stress (25% FC). To perform the enzyme assay and mRNA extraction, young leaves were collected 30 days after drought stress treatment and kept in liquid nitrogen immediately.
Table 1. Specifications, genetic formula and sampling area of the wild wheat species belonging to the Triticeae genus, Aegilops and Triticum, in this study.
mRNA extraction and cDNA synthesis
Total RNA of 100 mg of leaf tissue were extracted using RNX-PlusTM Kit (Sinaclon) according to the supplier’s instructions. Genomic DNA contamination was eliminated using RNase-free DNase I (Fermentas, #N0521) and the concentration of total RNA was estimated spectrophotometrically (Nano-Drop Thermo Scientific–2000C, USA). The single-stranded cDNA synthesis was carried out from 1 µg of total RNA using Hyper ScriptTM Reverse Transcriptase Kit (GeneAll, Korea) by the manufacturer’s instructions in a final volume of 20 μL. The housekeeping gene ‘Actin’ as a reference gene was selected to normalize the expression data for the studied Wdhn13, SOD, APX and GPX genes. Special primer pairs were designed for the Wdhn13 gene based on the GenBank database (Accession No. AB863588.1); the primers for SOD, APX and GPX genes were selected from Baek and Skinner [Citation26]. The selected primers were synthesized by the Bioneer Company (South Korea). The primer sequences for the selected genes are listed in .
Table 2. Sequences of primers used for real-time PCR.
Quantitative real-time PCR
Real-time PCR was carried out in a MiniOpticon™ Real-Time PCR System (Bio-Rad, USA) in a volume of 15 μL containing 7.5 μL of 2x RealQ Plus Master-Mix Green (Ampliqon), 1 μL of diluted cDNA, 0.5 μmol/L of forward and reverse primers, and 5.5 μL of RNase-free water. The PCR programme was as follows: first denaturation in 94 °C for 3 min, followed by 45 cycles included 94 °C for 15 s, 58 °C for 20 s, 72 °C for 30 s. The specificity of amplifications was surveyed with a heat-dissociated protocol at 65–95 °C as a final step of the PCR. The reactions were repeated two times (as two technical replications) for each of two biological samples.
Protein extraction and determination of enzyme activity
The protein extraction was carried out according to Kang and Saltveit [Citation27]. The protein extracts were used for the assay of antioxidant enzyme activities: APX, SOD and GPX. The APX activity was measured as a decrease in the absorbance at 290 nm by the method of Asada [Citation28]. The assay mixture contained 0.1 mmol/L ethylenediaminetetraacetic acid (EDTA), 0.05 mmol/L ascorbate, 1.54 mmol/L H2O2, 50 mmol/L phosphate buffer (pH = 7.6), and 60 µL sample extract. The SOD activity was estimated by its ability to inhibit the photochemical reduction of nitro-blue-tetrazolium (NBT) according to Dhindsa et al. [Citation29]. The reaction mixture included 0.1 mmol/L EDTA, 25 µL riboflavin, 50 mmol/L phosphate buffer (pH = 7.8), 75 µmol/L NBT, 13 mmol/L methionine, and 40 µL sample extract. The riboflavin was added last [Citation27], and the tubes were located 30 cm below two fluorescent lamps (15 W) and shaken for 15 min. The absorbance of each sample mixture was read at 560 nm. The GPX activity was assayed using absorbance increase rate at 420 nm for 1 min as described by Upadhyaya et al. [Citation30]. The reaction mixture consisted of 1.25 mmol/L phosphate buffer (pH = 6.1), 0.5 mL H2O2 (1%), 0.5 mL guaiacol (1%), and 20 µL sample extract.
Statistical analysis
The gene relative expressions were calculated using Pfaffl [Citation31] formula. The normalization of target genes was performed using the Actin gene as reference. The statistical analysis was carried out using two-way analysis of variance (ANOVA) in SAS software ver9.1 (SAS Institute, Cary, NC, USA). The mean comparisons were conducted using Duncan’s Multiple Range Test (DMRT) at p ≤ 0.01.
Results and discussion
Expression of Wdhn13 gene in Triticum and Aegilops species
The ANOVA showed significant (p ≤ 0.01) differences in the expression of the Wdhn13 gene among the stress treatments, species and their interaction (). The Wdhn13 expression was differentially regulated under water deficit (FC = 25%) and its expression increased two times more than in well-watered (FC = 100%) conditions. The relative expression of Wdhn13 in the drought-stressed and well-watered conditions was shown separately for the studied species of Aegilops and Triticum along with the drought-tolerant (Sirvan) and susceptible (Darya) reference cultivars in . The highest expression increase in Wdhn13 was observed in T. aestivum, Ae. crassa and Ae. tauschii. All species, except for the sensitive reference cultivar, Darya, showed higher expression under conditions of drought than that in the well-watered. Furthermore, the Wdhn13 expression in T. boeoticum and Ae. crassa was higher in the drought-stressed condition compared to the tolerant reference cultivar, Sirvan, and the other species. The expression of this gene was also different between the susceptible and tolerant reference cultivars and Sirvan, the drought-tolerant one having a higher expression level ().
Figure 1. Relative expression level of the Wdhn13 gene in the different species belonging to the Triticeae genus along with two tolerant (Sirvan) and sensitive (Darya) reference cultivars under well-watered (FC = 100%) and drought-stressed (FC= 25%) conditions. Values are means with standard error of the means (S.E.M.) and different letters indicate a significant difference at p ≤ 0.01.
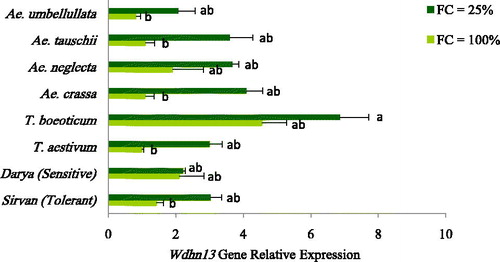
Table 3. Analysis of variance (ANOVA) for the relative expression of Wdhn13, the antioxidant genes, and the enzyme activity in the different species belonging to the Triticeae genus under the well-watered (FC = 100 %) and drought-stressed (FC = 25 %) conditions.
Dehydrins usually cumulate in plants in response to the various environmental stresses including dehydration and osmotic stresses [Citation32]. Increasing the dehydrin gene expression and the accumulation of its proteins will intensify the resistance of the plant to the stress conditions [Citation33]. In another study of some DHN genes in barley cultivars at drought-stressed conditions, the relative expression of the assayed DHN genes was greatly increased in the tolerant cultivars [Citation34]. Moreover, in the study of four DHN genes in two tolerant and susceptible cultivars at the drought-stressed conditions, a higher expression of Wdhn13 gene was reported in the tolerant cultivar. Additionally, Wdhn13 is an abscisic acid (ABA)-responsive gene that is associated with tolerance to drought stress and seems to be involved in the ABA-dependent drought tolerance pathway [Citation35]. According to our results, the expression of Wdhn13 under the drought stress was higher in T. boeoticum and Ae. crassa compared to the tolerant reference cultivar; therefore, they could be considered as more tolerant to drought stress. Dehydrins are important protein chaperons that accumulate in response to drought stress [Citation36]. However, the accumulation rate of compatible solutes (such as protein and proline) alters widely among different plant species [Citation37]. In this research, the total protein in the leaves of Ae. umbellulata increased to a higher extent at the drought stress treatment than that in the other species. This suggests that the increase in total protein during drought stress induction is likely an adaptive mechanism in this species.
Expression of the genes encoding antioxidant enzymes in Triticum and Aegilops species
The analysis of variance showed significant (p ≤ 0.01) differences in the expression of the APX, SOD and GPX genes among the water stress treatments, species, and their interaction (). In drought-stress (FC= 25%), the expression of APX, SOD and GPX genes was increased by 8.3, 13.7, and 11.7-fold compared to the non-stress condition, respectively. The highest upregulation in the drought stress compared to the non-stress conditions was for SOD and the lowest expression was for APX ().
Figure 2. Relative expression level of the antioxidant genes (a), the antioxidant enzyme activity (b) in the well-watered (FC = 100%) and drought-stressed (FC = 25%) conditions in the different species belonging to the Triticeae genus. Values are means with standard error of the means (S.E.M.) and different letters indicate a significant difference at p ≤ 0.01.
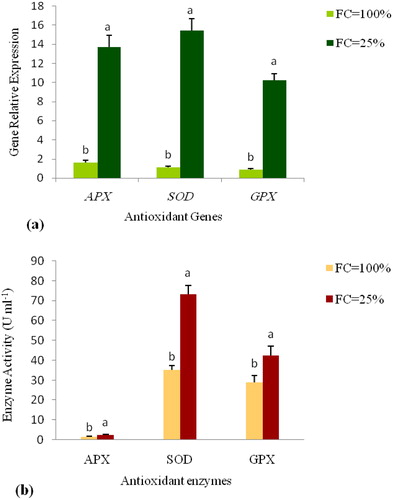
The mean comparison of the SOD gene expression among species () showed that the species Ae. tauschii, Ae. crassa and T. boeoticum had the highest expression increase rate from the well-watered to the drought-stressed condition, respectively. However, the expression levels of this gene showed that the SOD expression was increased in all species under the drought stress treatment compared to the well-watered conditions, and the increased rates in all studied species were significantly higher than that in the resistant reference cultivar, Sirvan. Furthermore, the expression level of this gene in the drought-tolerant reference cultivar was higher than that in the sensitive reference cultivar (Darya) ().
Figure 3. Relative expression of SOD (a), APX (b), GPX (c) gene in the different species belonging to the Triticeae genus along with two tolerant (Sirvan) and sensitive (Darya) reference cultivars under the well-watered (FC = 100%) and drought-stressed (FC = 25%) conditions. Values are means with standard error of the means (S.E.M.) and different letters indicate a significant difference at p ≤ 0.01.
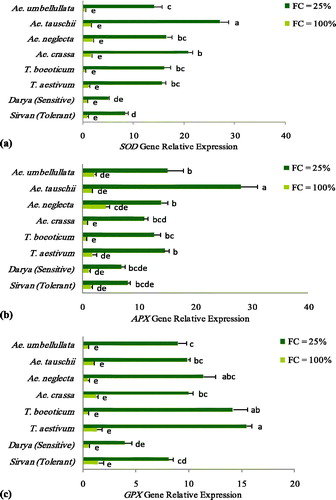
Under the non-stress and drought stress conditions, the expression of APX gene was significantly variable among species. The average expression level of the APX gene in the studied wheat species () showed that Ae. tauschii and Ae. umbellulata had the highest up-regulation at the drought stress condition. Moreover, the lowest increased rate of the gene expression was related to Ae. neglecta, whereas, at the well-watered condition, the highest APX expression was observed in this species. All species had a higher APX gene expression compared to the drought-tolerant reference cultivar under the drought stress conditions ().
The expression level of GPX among the species under drought stress ranked as follows: T. aestivum > T. boeoticum > Ae. Neglecta > Ae. Tauschii > Ae. Crassa > Ae. Umbellulata > tolerant reference cultivar; Sirvan > susceptible reference cultivar; Darya ().
The intervention of the antioxidant defense in raising the drought tolerance of plant species may also be reflected in the level of gene transcription. Transcriptome studies in plants have shown the mediation of stress responses to salinity and drought by changed gene expression [Citation38]. APX, as the main component in the ascorbate- glutathione cycle, has a crucial role in the balancing of the intercellular ROS accumulation. As a general rule, up-regulation of this gene improves the stress tolerance of plants [Citation39]. In this study, Ae. tauschii had the highest expression of the APX gene at the drought stress condition; hence, this species was considered as the best candidate for higher expression of the APX gene under drought and oxidative stresses. SOD genes act as the first defensive line against oxidative stress in plants [Citation40]. Furthermore, the higher expression of SOD genes has been reported to result in improved oxidative stress tolerance in plants [Citation39]. In the present study, the up-regulation of the SOD gene under the drought stress was related to the species containing D genome, i.e. Ae. tauschii (DD) and Ae. crassa (MMDD). Similar to other antioxidant genes, GPX can act as the effective defense line in scavenging H2O2 under the stressed conditions [Citation41]. In the present study, under the drought stress conditions all species exhibited a higher expression of GPX gene compared to the drought-tolerant reference cultivar, Sirvan. The higher expression observed for this gene in all studied species suggests a considerable ability of several alien genomes for the active expression of GPX in the response to drought stress.
In this study, three genes encoding antioxidant enzymes exhibited different expression patterns among the studied species of Aegilops and Triticum in response to drought. Unlike the conditions of drought, fewer species differences were detected in the expression levels of APX, GPX and SOD under well-watered condition. Their expression, however, was significantly inhibited by the drought stress in both reference cultivars, and the transcript levels of the antioxidant genes were significantly higher in Sirvan than those in Darya. These data suggest that the better drought tolerance of species is likely associated with increased expression of specific genes encoding antioxidant enzymes. In agreement, an Arabidopsis thaliana mutant with a higher expression of APX was more resistant to drought than the wild-type plants and showed a better water use efficiency [Citation42]. Additionally, the over-expression of Cu/ZnSOD derived from rice in Nicotiana tabacum strengthened the antioxidant system of chloroplasts and showed more tolerance to drought and osmotic stress than the wild-type [Citation43]. The transcript abundance of APX and SOD genes largely increased in some species such as Ae. tauschii at the drought stress condition, but relatively small increases were observed in SOD and APX enzyme activity. Furthermore, the expression pattern of the APX gene in T. aestivum and Ae. neglecta did not change with the enzyme activity alterations. In accordance, Mittler and Zilinskas [Citation44], Luna et al. [Citation45] and Reddy et al. [Citation46] reported inverse relationships between the transcript levels and related enzyme products. The discrepancy between the gene expression and enzymatic activity in some species during drought stress condition demonstrates that the enzyme changes were not caused at the mRNA levels but were regulated at the post-transcriptional level, which may be due to the enzyme activation/inactivation or synthesis/degradation activities induced by drought.
Antioxidant enzymes activity in response to drought in Triticum and Aegilops species
In the present study, Ae. umbellulata showed the highest total protein content increase (28.7%) from the well-watered to the drought-stressed condition followed by the tolerant reference cultivar, Sirvan, (17.5%). Also, the highest protein content decrease (37%) was related to Ae. neglecta. Furthermore, in Ae. carssa, Ae. tauschii, T. boeoticum and T. aestivum, it decreased slightly (7.2, 2.1, 9.1, 4.5% respectively) under the drought stress conditions (). The significant differences observed in the activity of three studied antioxidant enzymes among the drought stress treatments, species and their interaction (). The drought stress significantly increased the antioxidant activities of APX, SOD and GPX by 50, 107 and 47% on average, respectively (). The increasing trend of APX activity under drought stress was led by Ae. crassa, followed by the tolerant reference cultivar (Sirvan), then Ae. umbellulata, T. boeoticum, susceptible reference cultivar (Darya) and Ae. tauschii (). The APX activity under drought stress was lower than that under the well-watered conditions in Ae. neglecta and T. aestivum. In the tolerant reference cultivar, Sirvan, it increased significantly at drought-stressed treatment in comparison to the well-watered conditions.
Figure 4. Changes in the leaf total protein content in the different species belonging to the Triticeae genus along with two tolerant (Sirvan) and sensitive (Darya) reference cultivars under well-watered (FC = 100%) and drought-stressed (FC = 25%) conditions.
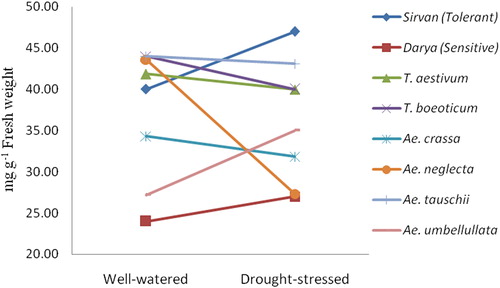
Figure 5. Changes in APX (a), SOD (b) and GPX (c) enzyme in the different species belonging to the Triticeae genus along with two tolerant (Sirvan) and sensitive (Darya) reference cultivars under well-watered (FC = 100%) and drought-stressed (FC = 25%) conditions. Values are means with standard error of the means (S.E.M.) and different letters indicate a significant difference at p ≤ 0.01.
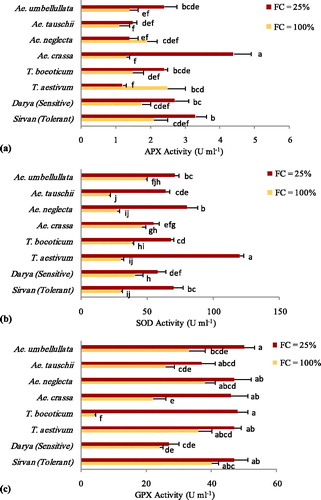
The highest activity of SOD at well-watered conditions was observed in Ae. crasa and Ae. umbullata (). Drought strongly affected the SOD activity, and maximum SOD activity at conditions of drought was observed in T. aestivum and Ae. neglecta. In the tolerant reference cultivar, Sirvan, the SOD activity under drought stress was much higher (233%) than that in the well-watered conditions, but in the susceptible reference cultivar, Darya, it increased slightly (41%) in comparison to the well-watered conditions. The enhancement of the SOD activity at drought stress compared to that at well-watered conditions in two species, T. aestivum (400%) and Ae. neglecta (296%), was higher than that in the tolerant reference cultivar (). The maximum GPX activity was observed in Ae. umbellullata, Ae. neglecta, T. boeioticum and the tolerant reference cultivar, Sirvan, at the drought stress conditions (). Furthermore, the highest enhancement of the GPX activity from the well-watered to the drought-stressed conditions was observed in T. boeioticum ().
The antioxidant enzyme activities, such as those of APX, CAT, GPX and SOD, increase under various abiotic stresses [Citation47, Citation48] and a comparatively higher activity has also been reported in the tolerant cultivars [Citation49–51], suggesting that a higher antioxidant enzyme activity had a key role in giving resilience to these cultivars against the abiotic stress. Esfandiari et al. [Citation52] reported that the activation of the antioxidant enzymes was necessary for the tolerance to the drought stress in wheat. Studies indicated that species tolerant to abiotic stress can reciprocate the stress through the antioxidant defense systems. Therefore, there is a correlation between the antioxidant defense system and the tolerance to stress [Citation53]. The diversity in the changes in the antioxidant enzymes that occur in the drought tolerance response may be due to differences in the levels of tolerance among plant species. In our experiment, the results indicated that the various wheat species clearly responded to the water-deficit stress differently in terms of APX, GPX and SOD activities to scavenge the reactive oxygen species. It is obvious that increasing the activity of antioxidant enzymes is one strategy of the drought tolerance mechanisms in plants.
The APX enzyme, available in the chloroplasts and the cytosol, can eliminate H2O2 efficiently, primarily in the chloroplast where catalase is absent. In our study, a maximum APX activity increase (338%) occurred in Ae. crassa followed by Ae. umbellulata, T. boeoticum and Ae. tauschii at the drought-stressed conditions. Suneja et al. [Citation54] reported an increase in the APX activity in Ae. tauschii in response to drought stress. In this study, drought caused a reduction in the APX activities in T. aestivum and Ae. neglecta. The reduction in the APX activity during drought stress may lead to an increase in lipid peroxidation in both species. The SOD is a potent antioxidant enzyme because it dismutates superoxide free radicals with an incredible effectiveness, resulting in the generation of H2O2 and O2. Other studies have reported the key role of SOD in the anti-oxidative defense [Citation55, Citation56], assessing the water resistance status of plants [Citation54] and as a proper selection index for screening the drought-tolerant wheat [Citation57]. In our study, the examined species varied in their SOD activity in response to water stress. This indicates that different species of wheat showed discrete drought stress thresholds, but maximum SOD activity was observed in T. aestivum and Ae. neglecta under drought stress. Since the detected enzyme activity is a consequence of both synthesis and decomposition, any reduction in net SOD under stress could be attributed to either the enhanced degradation or reduced synthesis of the enzyme [Citation58]. GPX is another important enzyme that plays a key role in protecting against stresses through the removal of H2O2 in chloroplasts and biosynthesizing lignin [Citation59, Citation60]. In this research, the maximum GPX activity occurred in Ae. umbellullata and Ae. neglecta, both having a U genome.
Conclusions
The obtained results showed the relevant agreement between the genetically determined drought tolerance of wheat species using gene transcript levels and their corresponding antioxidant enzyme activities. The relative expression of the Wdhn13 gene at drought stress condition was higher in T. boeoticum and Ae. crassa compared to the tolerant reference cultivar and the other species. In the functional genomics analyses of the selected species, the species possessing Ab (T. boeoticum), D (Ae. tauschii) and D (Ae. tauschii and Ae.crassa) genomes had a higher expression of GPX, SOD and APX genes, respectively.
Acknowledgements
The authors are grateful to the genomics laboratory manager of Imam Khomeini International University, who provided insight and expertise that greatly assisted this research.
Disclosure statement
No potential conflict of interest was reported by the authors.
Additional information
Funding
References
- Tian Y, Guan B, Zhou D. Responses of seed germination, seedling growth, and seed yield traits to seed pretreatment in maize (Zea mays L.). Sci World J. 2014;1–8.
- Patade VY, Maya K, Zakwan A. Seed priming mediated germination improvement and tolerance to subsequent exposure to cold and salt stress in capsicum. Res J Seed Sci. 2011;4:125–136.
- Choi DW, Zhu B, Close TJ. The barley (Hordeum vulgare L.) dehydrin multigene family: sequences, allele types, chromosome assignments, and expression characteristics of 11 Dhngenes of cv. Dicktoo. Theor Appl Genet. 1999;98:1234–1247.
- Allagulova CR, Gimalov FR, Shakirova FM. The plant dehydrins: structure and putative functions. Biochemistry. 2003;68:945–951.
- Kosova K, Holkova L, Prasil IT, et al. Expression of dehydrin 5 during the development of frost tolerance in barley (Hordeum vulgare). J Plant Physiol. 2008;165:1142–1151.
- Drira M, Saibi W, Brini F, et al. The K-segments of the wheat dehydrin DHN-5 are essential for the protection of lactate dehydrogenase and b-glucosidase activities in vitro. Mol Biotechnol. 2013;54:643–650.
- Rorat T. Plant dehydrins, tissue location, structure and function. Cell Mol Biol Lett. 2006;11:536–556.
- Ohno R, Takumi S, Nakamura C. Kinetics of transcript and protein accumulation of a low-molecular weight wheat LEA D-11 dehydrin in response to low temperature. Plant Physiol. 2003;169:193–200.
- Ramezani A, Niazi A, Abolimoghadam AA, et al. Quantitative expression analysis of TaSOS1 and TaSOS4 genes in cultivated and wild wheat plants under salt stress. Mol Biotechnol. 2013;3:189–197.
- Zhang Y, Li Y, Peng Y, et al. Clones of FeSOD, MDHAR, DHAR genes from white clover and gene expression analysis of ROS-scavenging enzymes during abiotic stress and hormone treatments. Molecules. 2015;20:20939–20954.
- Hossain MS, Dietz KJ. Tuning of redox regulatory mechanisms, reactive oxygen species and redox homeostasis under salinity stress. Front Plant Sci. 2016; [cited 2019 Jun 12]7:548.
- Sofo A, Scopa A, Nuzzaci M, et al. Ascorbate peroxidase and catalase activities and their genetic regulation in plants subjected to drought and salinity stresses. IJMS. 2015;16:13561–13578. doi: http://dx.doi.org/10.3390/ijms160613561.
- Scandalios JG. Oxidative stress: molecular perception and transduction of signals triggering antioxidant gene defenses. Braz J Med Biol Res. 2005;38:995–1014.
- Mittler R, Vanderauwera S, Gollery M, et al. Reactive oxygen gene network of plants. Trends Plant Sci. 2004;9:490–498.
- Yousfi S, Márquez AJ, Betti M, et al. Gene expression and physiological responses to salinity and water stress of contrasting durum wheat genotypes. J Integr Plant Biol. 2016;58:48–66.
- Blum A. Drought resistance-is it really a complex trait? Functional Plant Biol. 2011;38:753–757.
- Ashraf M. Inducing drought tolerance in plants: recent advances. Biotechnol Adv. 2010;28:169–183.
- Polle A. Dissecting the superoxide dismutase-ascorbate-glutathione-pathway in chloroplasts by metabolic modeling. Computer simulations as a step towards flux analysis. Plant Physiol. 2001;126:445–462.
- Gadjev I, Vanderauwera S, Gechev TS, et al. Transcriptomic footprints disclose specificity ofreactive oxygen species signaling in Arabidopsis. Plant Physiol. 2006;141:436–445.
- Laloi C, Apel K, Danon A. Reactive oxygen signalling: the latest news. Curr Opin Plant Biol. 2004;7:323–328.
- Rampino P, Pataleo S, Gerardi C, et al. Drought stress response in wheat: physiological and molecular analysis of resistant and sensitive genotypes. Plant Cell Environ. 2006;29:2143–2152.
- Zamani-Bangohari M, Niazi A, Moghaddam AA, et al. Genome-wide analysis of key salinity-tolerance transporter (HKT;5) in wheat and wild wheat relatives (A and D genomes). In Vitro Cell Dev Biol Plant. 2013;49:97–106.
- Kiani R, Arzani A, Habibi F. Physiology of salinity tolerance in Aegilop scylindrica. Acta Physiol Plant. 2015;37:135–145.
- Masoomi-Aladizgeh F, Aalami A, Esfahani M, et al. Identification of CBF14 and NAC2 genes in Aegilops tauschii associated with resistance to freezing stress. Appl Biochem Biotechnol. 2015;176:1059–1070.
- Souza CC, Oliveira FA, Silva IF, et al. Evaluation of methods of available water determination and irrigation management in terra roxa under cotton crop. Rev Bras Eng Agríc Ambient. 2000;4:338–342.
- Baek KH, Skinner DZ. Alteration of antioxidant enzyme gene expression during cold acclimation of near-isogenic wheat lines. Plant Sci. 2003;165:1221–1227.
- Kang HM, Saltveit ME. Antioxidant enzymes and DPPH radical scavenging activity in chilled and heat shocked rice (Oryza sativa L.) seedling radicles. J Agric Food Chem. 2002;50:513–518. https://doi.org/10.1021/jf011124d
- Asada K. Ascorbate peroxidase A hydrogen peroxide-scavenging enzyme in plants. Physiol Plant. 1992;85:235–241.
- Dhindsa RS, Plumb-Dhindsa P, Thorpe TA. Leaf senescence: correlated with increased levels of membrane permeability and lipid peroxidation, and decreased levels of superoxide dismutase and catalase. J Exp Bot. 1981;32:93–101. https://doi.org/10.1093/jxb/32.1.93
- Upadhyaya A, Sankhla D, Davis TD, et al. Effect of paclobutrazol on the activities of some enzymes of activated oxygen metabolism and lipid peroxidation insenescing soybean leaves. J Plant Physiol. 1985;121:453–461. https://doi.org/10.1016/S0176-1617(85)80081–X
- Pfaffl MW. A new mathematical model for relative quantification in real-time RT-PCR. Nucleic Acids Res.. 2001;29:e45. [cited 2019 Jun 12].
- Close TJ. Dehydrins: A commonality in the response of plants todehydration and low temperatures. Physiol Plant. 1997;100:291–296.
- Porcel R, Azcon R, Ruiz-Lozano JM. Evaluation of the role of genes encoding for dehydrin proteins (LEA D-11) during drought stress in arbuscular mycorrhizal Glycine max and Lactuca sativa plants. J Exp Bot. 2005;56:1933–1942.
- Guo P, Baum M, Grando S, et al. Differentially expressed genes between drought-tolerant and drought-sensitive barley genotypes in response to drought stress during the reproductive stage. J Exp Bot. 2009;60:3531–3544.
- Kaur M, Gupta AK, Zhawar VK. Antioxidant response and Lea gene expression under exogenous ABA and water deficit stress in wheat cultivars contrasting in drought tolerance. J Plant Biochem Biotechnol. 2014;23:18–30.
- Ennajeh M, Vadel AM, Khemira H. Osmoregulation and osmoprotection in the leaf cells of two olive cultivars subjected to severe water deficit. Acta Physiol Plant. 2009;31:711–721. https://doi.org/10.1007/s11738-009-0283-6
- Kadkhodaie A, Zahedi M, Razmjoo J, et al. Changes in some anti-oxidative enzymes and physiological indices among sesame genotypes (Sesamum indicum L.) in response to soil water deficits under field conditions. Acta Physiol Plant. 2014;36:641–650. https://doi.org/10.1007/s11738-013-1442-3
- Burklew CE, Ashlock J, Winfrey WB, et al. Effects of aluminum oxide nanoparticles on the growth, development, and microRNA expression of tobacco (Nicotiana tabacum). PLoS One. 2012;7:e34783. [cited 2019 Jun 12]; https://doi.org/10.1371/journal.pone.0034783
- Wang Y, Wisniewski M, Meilan R, et al. Overexpression of cytosolic ascorbate peroxidase in tomato confers tolerance to chilling and salt stress. J Am Soc Hortic Sci. 2005;130:167–173.
- Verma KK, Singh M, Gupta RK, et al. Photosynthetic gas exchange, chlorophyll fluorescence, antioxidant enzymes, and growth responses of Jatroph acurcas during soil flooding. Turk J Bot. 2014;38:130–140.
- Vangronsveld J, Clijsters H. Toxic effects of metals. In: Farago ME, editor. Plants and the chemical elements: biochemistry, uptake, tolerance and toxicity. Weinheim, Germany: VCH Publishers; 1994. p. 50–177.
- Rossel JB, Walter PB, Hendrickson L, et al. A mutation affecting ascorbate peroxidase 2 gene expression reveals a link between responses to high light and drought tolerance. Plant Cell Environ. 2006;29:269–281.
- Badawi GH, Yasuo Y, Emi S, et al. Enhanced tolerance to salt stress and water deficit by overexpressing superoxide dismutase in tobacco (Nicotiana tabacum) chloroplasts. Plant Sci. 2004;166:919–928.
- Mittler R, Zilinskas BA. Regulation of pea cytosolic ascorbate peroxidase and other antioxidant enzymes during the progression of drought stress and following recovery from drought. Plant J. 1994;5:397–405.
- Luna CM, Pastori GM, Driscoll S, et al. Drought controls on H2O2 accumulation, catalase (CAT) activity and CAT gene expression in wheat. J Exp Bot. 2005;56:417–423.
- Reddy PCO, Sairanganayakulu G, Thippeswamy M, et al. Identification of stress-induced genes from the drought tolerant semi-arid legume crop horsegram (Macrotyloma uniflorum) through analysis of subtracted expressed sequence tags. Plant Sci. 2008;175:372–384.
- Gueta-Dahan Y, Yaniv Z, Zilinskas BA, et al. Salt and oxidative stress: similar and specific responses and their relation to salt tolerance in citrus. Planta. 1997;203:460–469.
- Yu Z, Rengel Q. Drought and salinity differentially influence activities of superoxide dismutase in narrow leafed lupines. Plant Sci. 1999;141:1–11.
- Sairam RK, Deshmukh PS, Shukla DS. Tolerance of drought and temperature stress in relation to increased antioxidant enzyme activity in wheat. J Agron Crop Sci. 1997;178:171–177.
- Hernandez JA, Jimenez A, Mullineaux P, et al. Tolerance of (Pisum sativum) to long term salt stress is associated with induction of antioxidant defences. Plant Cell Biol. 2000;23:853–862.
- Farooq M, Hussain M, Nawaz A, et al. Seed priming improves chilling tolerance in chickpea by modulating germination metabolism, trehalose accumulation and carbon assimilation. Plant Physiol Biochem. 2017;111:274–283. https://doi.org/10.1016/j.plaphy.2016.12.012
- Esfandiari E, Shakiba MR, Mahboob SA, et al. Effect of water stress on antioxidant enzymes activities and lipid peroxidation of wheat seedlings. Agri Sci. 2007;19:130–138.
- Demiral T, Turkan I. Does exogenous glycine betaine affect antioxidative system of rice seedlings under NaCl treatment? J Plant Physio. 2004;61:1089–1100.
- Suneja Y, Gupta AK, Bains NS. Bread wheat progenitors: Aegilops tauschii (DD genome) and Triticum dicoccoides (AABB genome) reveal differential antioxidative response under water stress. Physiol Mol Biol Plants. 2017;23:99–114. https://doi.org/10.1007/s12298-016-0409-4
- Huseynova IM, Aliyeva DR, Aliyev JA. Subcellular localization and responses of superoxide dismutase isoforms in local wheat varieties subjected to continuous soil drought. Plant Physiol Biochem. 2014;81:54–60.
- Alscher RG, Erturk N, Heath LS. Role of superoxide dismutases (SODs) in controlling oxidative stress in plants. Exp Bot. 2002;53:1331–1341.
- Ren J, Sun LN, Zhang QY, et al. Drought tolerance is correlated with the activity of antioxidant enzymes in Cerasus humilis seedlings. Biomed Res Int. 2016;1–9.
- Zhang J, Kirkham MV. Drought stress induced changes in activities of superoxide dismutase, catalase, and peroxidase in wheat species. Plant Cell Physiol. 1994;35:785–791.
- Vieira EA, Silva MG, Moro CF, et al. Physiological and biochemical changes attenuate the effects of drought on the Cerrado species Vatairea macrocarpa (Benth.) Ducke. Plant Physiol Biochem. 2017;115:472–483. https://doi.org/10.1016/j.plaphy.2017.04.022
- Aydın S, Buyuk I, Aras ES. Expression of SOD gene andevaluating its role in stress tolerance in NaCl and PEG stressed Lycopersicum esculentum. Turk J Bot. 2014;38:89–98.