Abstract
This study aimed to determine the expression of microRNA (miRNA or miR)-378 in peripheral blood from patients with pregnancy-induced hypertension (PIH), and to try to understand its effect on vascular endothelial injuries induced by PIH. The study included 33 PIH patients. Quantitative real-time polymerase chain reaction was used to determine the expression of miR-378 in peripheral blood and NK cells. NK cells were transfected with miR-378 mimics and its negative control. Bioinformatics was used to predict the potential target gene of miR-378. Flow cytometry and Western blotting were performed to identify changes in the expression of CD226 protein. By co-culturing HUVECs with NK cells, the effects of miR-378 and CD226 on the proliferation, migration and apoptosis of HUVECs were evaluated. The results showed that the expression of miR-378 in peripheral blood was down-regulated with the progression of PIH. The expression of miR-378 was reduced in innate immune NK cells from peripheral blood of PIH patients. miR-378 negatively regulated the expression of CD226 in NK cells, and CD226 is a target gene of miR-378. miR-378 inhibited the expression and secretion of soluble CD226 by NK cells. miR-378 regulated the proliferation, migration and apoptosis of HUVECs by affecting the levels of soluble CD226 expressed by NK cells. The present study demonstrates that miR-378 is down-regulated in peripheral blood and NK cells from patients with PIH, and negatively correlated with the development of PIH. miR-378 protects HUVECs from injuries by soluble CD226 through down-regulating the expression of soluble CD226 in NK cells.
Introduction
Pregnancy-induced hypertension (PIH) is one of the most common complications in pregnancy [Citation1]. Epidemiological statistics show that the incidence of PIH in the world has reached 10%, and it seriously threatens the health of pregnant women and perinatal infants [Citation2,Citation3]. With the increase of age and obesity of mothers in recent years, the incidence of PIH is gradually rising [Citation4]. If PIH is not controlled, it will develop into preeclampsia, or even moderate to severe eclampsia [Citation5]. It is noteworthy that moderate to severe eclampsia is a type of life-threatening multisystem disease, and its complications include eclampsia, HELLP (hemolysis, elevated liver enzymes and low platelet) syndrome, renal dysfunction, pulmonary edema, placental abruption and fetal intrauterine death, etc [Citation5,Citation6]. Therefore, in-depth study of the pathophysiological process of PIH and improvement of diagnosis and treatment strategies are of great significance for the early clinical intervention and improvement of maternal and infant outcomes. At present, the pathogenesis of PIH is still unclear, and further research is needed.
Two important clinicopathological changes of PIH are vasoconstriction and impaired uterine-placental perfusion, both of which are related to vascular dysfunction [Citation7]. Endothelial cells are natural physical barriers to the inner wall of blood vessels, and they play important roles in maintaining the stability of vascular tension and regulating blood coagulation and inflammatory responses [Citation8,Citation9]. Activation and dysfunction of endothelial cells can affect vasoconstriction, leading to elevated blood pressure, thrombosis and interaction between inflammatory endothelial cells and inflammatory cells in vivo [Citation10]. For example, interaction among leukocytes is considered to play an important role in the pathogenesis of PIH [Citation11]. Therefore, studies on the molecular mechanism of dysfunction of vascular endothelial cells in PIH are of great significance in understanding the pathway of vascular injury induced by PIH and screening clinical therapeutic targets.
MicroRNA (miRNA or miR) is a class of non-coding small RNA molecules (18-22 nt) that regulate the translation of proteins by directly binding with the 3’-untranslated region (UTR) of their mRNA [Citation12,Citation13]. It is reported that miRNA molecules are involved in almost all pathophysiological processes, being important biomarkers and therapeutic targets [Citation14]. MiRNAs also play key roles in blood pressure regulation and vascular injury [Citation15]. For example, miR-758 mediates oxLDL-dependent vascular endothelial injury by down-regulating SUCNR1 gene [Citation16]. In diabetes, miR-24 regulates vascular remodeling by targeting and inhibiting PDGF-BB [Citation17]. miR-378, a recently discovered miRNA molecule located on human chromosome 5, has a highly conserved sequence among all vertebrates, with its effect chain being miR-378a-3p [Citation18]. miR-378 is involved in the proliferation, drug resistance and differentiation of various tissues and cells, such as tumour cells, myoblasts and glomerular podocytes [Citation19,Citation20]. These studies suggest that miR-378 has different biological functions among different diseases. However, the expression and function of miR-378 in vascular endothelial cell injury induced by PIH still remain unclear. In the present study, we determine the expression of miR-378 in peripheral blood of patients with PIH and try to understand its functions in the proliferation, migration and apoptosis of vascular endothelial cells.
Subjects and methods
Patients
A total of 33 pregnant women with PIH who underwent uterine-incision delivery at our hospital between January 2017 and January 2018 were included in the present study. Among the 33 patients, 17 cases had PIH (increased blood pressure and no proteinuria after 20 weeks of gestation), 10 cases had mild preeclampsia (mPE; systolic pressure ≥ 140 mmHg or diastolic pressure ≥ 90 mmHg; 24 h proteinuria ≥ 300 mg), and 6 cases had severe preeclampsia (sPE; systolic pressure ≥ 160 mmHg or diastolic pressure ≥ 100 mmHg; 24 h proteinuria ≥ 2 g). In the group of patients, the age range was 23–38 years; the mean age was 35.4 years and the median age was 34 years. In addition, 30 women with normal labour were included in the control group. In the control group, the age range was 22–36 years, the mean age was 28.5 years and the median age was 27 years. Both groups of pregnant women were with single pregnancies. None of the pregnant women had heart, liver, kidney or blood system diseases, diabetes, rheumatism, autoimmune diseases, infection or medication history within 4 weeks before operation. Peripheral blood (5 mL) was collected from all patients and control subjects. Natural killer (NK) cells were isolated from the group of women with normal labour.
Ethics statement
All procedures performed in this study were approved by the Ethics Committee of The First People’s Hospital of Lanzhou City. Written informed consent was obtained from all patients or their families.
Cells
Human umbilical vein endothelial cells (HUVECs; Cell Bank, Chinese Academy of Sciences, Shanghai, China) were cultured in DMEM medium supplemented with 10% fetal bovine serum (FBS) under 37 °C and 5% CO2. When reaching 70–90% confluency, the cells were trypsinised and passaged. HUVECs at 3–5 generations were used for experiments.
Peripheral blood mononuclear cells (PBMCs) were isolated from 2 mL anticoagulant venous blood using Ficoll lymphocyte separation solution. Then, PBMCs were mixed with 5 volumes of sterile phosphate-buffered saline (PBS) and centrifuged at 1200 g (Allegra 25 R; Beckman Coulter, Brea, CA, USA) for 6 min before removing the supernatant. Then, the cell pellets were resuspended using sterile PBS.
Quantitative real-time polymerase chain reaction
Peripheral blood (150 μL) was lysed with 750 μL TRIzol reagent, and cells (1 × 106) were lysed with 1 mL TRIzol reagent following the manufacturer’s manual (Thermo Fisher Scientific, Waltham, MA, USA) for total RNA extraction. Then, cDNA was obtained by reverse transcription from 1 μg RNA and stored at −80 °C. Reverse transcription of miRNA was carried out using miScript II RT Kit (Qiagen, Hilden, Germany) according to the manufacturer’s manual.
The expression of miR-378 was determined by miScript SYBR® Green PCR Kit (Qiagen, Hilden, Germany), using U6 as internal reference. The sequence of the miR-378 forward primer was 5'- ACTGGACTTGGAGTCAGAAGGC -3' (forward), and the reverse primer of miR-378 was a common primer (provided by the kit). The sequences of the U6 primers were 5'- CTCGCTTCGGCAGCACA -3' (forward) and 5'- AACGCTTCACGAATTTGCGT -3' (reverse). The reaction system (30 μL) was composed of cDNA (5 μL), Mix (10 μL), upstream primer (1 μL), downstream primer (1 μL) and ddH2O (13 μL). The reaction protocol was: initial denaturation at 95 °C for 5 min; denaturation at 95 °C for 30 s and annealing at 60 °C for 30 s (40 cycles) (iQ5; Bio-Rad, Hercules, CA, USA). The 2−ΔΔCt method was used to calculate the relative expression of miR-378 against U6. Each sample was tested in triplicate wells and the results were averaged.
Separation, purification and culture of NK cells
MagniSort™ Mouse NK cell Enrichment Kit (Thermo Fisher Scientific, Waltham, MA, USA) was used to separate NK cells in peripheral blood from normal pregnant women. Briefly, the separated PBMCs were mixed with PBS to reach 10 mL, and centrifuged at 300 g and room temperature for 10 min before removing the supernatant. Then, 5 mL PBS was added to resuspend the cells, which were then centrifuged at 250 g for 10 min before removing the supernatant. The cells were resuspended in 1 mL 1x BD IMag buffer before use. From the suspended solution, 10 μL was aspirated and mixed with 190 μL PBS. Then, 10 μL biotinylated human NK cell concentrate was added and incubated in dark at room temperature for 30 min. Then, 1.8 mL 1X BD buffer was added to wash off biotin, followed by centrifugation at 300 g for 10 min. Finally, the cells were resuspended in 500 μL 1X BD buffer, followed by addition of an equal volume of beads solution. After incubation in dark for 30 min, the mixture was mixed gently and placed on magnet for 15 min. The supernatant was then transferred to a new Eppendorf tube, and NK cells were obtained. NK cells were cultured in RPMI-1640 medium supplemented with 10% FBS and 100 IU IL-2 at 37 °C and 5% CO2 for 48 h before use.
Bioinformatics analysis
On the TargetScan website (http://www.targetscan.org/vert_72/), the name “miR-378a-3p” was searched, and its potential target genes were obtained.
Dual luciferase reporter assay
According to the bioinformatics analysis, we synthesised wild-type (WT) and mutant seed regions of miR-378a-3p in the 3’-UTR of the CD226 gene in vitro. Then, their two ends were attached with Spe-1 and HindIII restriction sites, and then cloned into pMIR-REPORT luciferase reporter plasmids. Plasmids (0.8 μg) with WT or mutant 3’-UTR sequences were co-transfected with agomiR-378a-3p (100 nmol/L; Sangon Biotech, Shanghai, China) into 293 T cells. For control, 293 T cells were transfected with agomiR-negative control (NC). After cultivation for 24 h, the cells were lysed using dual luciferase reporter assay kit (Promega, Fitchburg, WI, USA) according to the manufacturer’s manual, and luminescence intensity was measured using GloMax 20/20 luminometer (Promega, Fitchburg, WI, USA). Renilla luminescence activity served as the internal reference to measure the luminescence values of each group of cells.
Transfection of NK cells
Sorted peripheral blood NK cells (1 × 105) were seeded onto 24-well plates and cultured in biotin-free RPMI-1640 medium supplemented with 10% FBS and 100 U IL-2. When reaching 70% confluency, the cells were used for transfection. In the first vial, 1.5 µL miR-378 mimics (20 pmol/µL; mimics group) (Hanbio Biotechnology Co., Ltd., Shanghai, China) was mixed with 50 µL Opti Mem medium (Thermo Fisher Scientific, Waltham, MA, USA). In the second vial, 1 µL Lipofectamine 3000 (Thermo Fisher Scientific, Waltham, MA, USA) was mixed with 50 µL Opti Mem medium. After standing still for 5 min, the two vials were combined for longer incubation at room temperature for 20 min. Then, the mixtures were added to the cells in the respective groups. Six hours later, the medium was replaced with RPMI-1640 medium containing 10% FBS and 100 U IL-2. After cultivation for 48 h, the cells were collected for further assays.
Cell-counting kit 8 (CCK-8) assay
HUVECs were trypsinised and seeded into 96-well plates at a density of 2 × 103/well. At 24, 48 and 72 h, the medium was discarded, and the cells were washed with PBS twice, followed by addition of DMEM medium and 10 μL CCK-8 reaction reagent (Beyotime, Shanghai, China). After incubation at 37 °C and 5% CO2 for 30 min, the absorbance of each well was measured at 490 nm (DNM-9606; Perlong Medical, Beijing, China) to plot the cell proliferation curves.
Flow cytometry
After being co-cultured for 7 days or at 48 h after transfection with miR-378 mimics, cells (1 × 106) in each group were washed with pre-cooled PBS twice and subjected to flow cytometry (FACSCalibur, BD Biosciences, Franklin Lakes, NJ, USA) using ANXN V FITC APOPTOSIS DTEC KIT I (BD Biosciences, Franklin Lakes, NJ, USA) following the manufacturer’s manual to detect cell apoptosis. Cells with ANNEXIN V-positive values were early apoptotic cells; those with PI-positive values were necrotic cells, and those with double positive values were late apoptotic cells.
Transwell assay
HUVECs (1 × 105) from each group were seeded into the upper chamber containing 200 μL serum-free RPMI-1640 medium. In addition, 500 μL RPMI-1640 medium supplemented with 10% fetal bovine serum was added into the lower chamber. After 24 h, the chamber was removed and the cells in the upper chamber were wiped off. After being fixed with 4% formaldehyde for 10 min, the membrane was stained using the Giemsa method for microscopic observation of 5 random fields (200×). The number of transwell cells was calculated for the evaluation of cell invasion and migration ability. All procedures were carried out on ice with pipetting tips being cooled at 4 °C.
Western blotting
NK cells (1 × 106) were trypsinised, collected and lysed with precooled Radio-Immunoprecipitation Assay (RIPA) lysis buffer (600 μL; 50 mmol/L Tris-base, 1 mmol/EDTA, 150 mmol/NaCl, 0.1% sodium dodecyl sulphate, 1% TritonX-100, 1% sodium deoxycholate; Beyotime Institute of Biotechnology, Shanghai, China) for 5 min on ice. The mixture was centrifuged at 12,000 g (Allegra 25 R; Beckman Coulter, Brea, CA, USA) and 4 °C for 10 min. The supernatant was then mixed with 5× sodium dodecyl sulphate loading buffer before denaturation in boiling water bath for 10 min. Afterwards, the samples (5 µL) were subjected to 10% sodium dodecyl sulphate-polyacrylamide gel electrophoresis at 100 V. The resolved proteins were transferred to polyvinylidene difluoride membranes on ice (250 mA, 1 h) and blocked with 50 g/L skimmed milk at room temperature for 1 h. Then, the membranes were incubated with rabbit anti-human CD226 (1:1000; BD Biosciences, Franklin Lakes, NJ, USA) or GAPDH (1:4000; BD Biosciences, Franklin Lakes, NJ, USA) primary antibodies at 4 °C overnight. After 3 times of extensive washing with phosphate-buffered saline with Tween 20 for 15 min, the membranes were incubated with goat anti-rabbit horseradish peroxidase-conjugated secondary antibody (1:4,000; BD Biosciences, Franklin Lakes, NJ, USA) for 1 h at room temperature before washing 3 times with phosphate-buffered saline with Tween 20 for 15 min. Then, the membrane was developed with enhanced chemiluminescence detection kit (Sigma-Aldrich, St. Louis, MO, USA) for imaging. Image lab v3.0 software (Bio-Rad, Hercules, CA, USA) was used to acquire and analyse the imaging signals. The relative content of the target proteins was expressed against GAPDH.
Statistical analysis
The results were analysed using SPSS 20.0 statistical software (IBM, Armonk, NY, USA). The data were expressed as means with standard deviations (±SD). Data were tested for normality. Multigroup measurement data were analysed using one-way analysis of variance (ANOVA). In case of homogeneity of variance, Least Significant Difference and Student-Newman-Keuls methods were used; in case of heterogeneity of variance, Tamhane’s T2 or Dunnett’s T3 method was used. Comparison between two groups was carried out using Student’s t-test. P < 0.05 indicated statistically significant differences.
Results and discussion
Expression of miR-378 in peripheral blood is down-regulated as the progression of PIH
PIH is the most common obstetric disease in clinic, being peculiar to pregnant women during pregnancy [Citation21]. After 20 weeks of gestation, patients usually show clinical manifestations such as hypertension and proteinuria. If untreated, it will develop into eclampsia, which is a great threat to the lives of mothers and fetuses, and can lead to deaths in serious cases [Citation22]. One of the basic pathological changes of PIH is vascular endothelial injury, which is related to persistent hypertension and immune abnormality [Citation23,Citation24]. As an important regulator at posttranscriptional level, miRNAs are also involved in the occurrence and development of PIH [Citation25]. For example, the expression of miR-210 is up-regulated in the peripheral blood of patients with PIH [Citation26]. Another study reported that the expression of miR-204-5p is up-regulated in the peripheral blood of PIH patients and is positively correlated with the clinical development of PIH [Citation27]. In addition, multiple miRNA molecules are abnormally expressed in the peripheral blood of patients with PIH, such as let-7 and miR-519d-3p [Citation28,Citation29]. To measure the expression of miR-378 in peripheral blood, we used qRT-PCR. The data showed that miR-378 expression in peripheral blood from patients with PIH was significantly lower than that in normal subjects (P < 0.05) (). In addition, miR-378 levels in peripheral blood were significantly different among patients with PIH, mPE and sPE (P < 0.05) (). These results suggest that the expression of miR-378 in peripheral blood is down-regulated in the course of PIH progression.
Figure 1. Expression of miR-378 in peripheral blood and its clinical relevance. (A) Relative expression of miR-378 in normal subjects and PIH patients. *, P < 0.05 compared with normal subjects. (B) Relative expression of miR-378 in peripheral blood of normal subjects and patients with PIH, mild preeclampsia (mPE) or severe preeclampsia (sPE). *, P < 0.05.
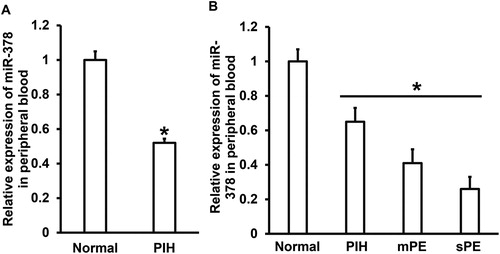
Expression of miR-378 is reduced in innate immune NK cells from peripheral blood of PIH patients
It is reported that pregnancy is closely related to the maternal autoimmune system [Citation30]. The mother’s body produces protective immune responses for the fetus until the fetus is delivered [Citation31]. Abnormal maternal immunity can lead to abnormal fetal development, injury or even abortion. For example, abnormal increase of NK cell activity in decidua is closely related to abortion [Citation32]. To determine the expression of miR-378 in PBMCs and NK cells from peripheral blood, we isolated NK cells from PBMCs and carried out qRT-PCR. The data showed that NK cells were successfully separated from PBMCs as a subgroup (). qRT-PCR showed that the expression of miR-378 in PBMCs from PIH patients was significantly lower than that from normal subjects (P < 0.05) (). Similarly, the expression of miR-378 in NK cells from PIH patients was significantly reduced compared to that from normal subjects (P < 0.05) (). The results indicate that the expression of miR-378 is reduced in innate immune NK cells from peripheral blood of PIH patients.
Figure 2. Expression of miR-378 in NK cells from peripheral blood. (A) Flow cytometric confirmation of the separation of NK cells from PBMCs. (B) Relative expression of miR-378 in PBMCs from normal subjects and PIH patients. *, P < 0.05 compared with normal subjects. (C) Relative expression of miR-378 in NK cells from normal subjects and PIH patients. *, P < 0.05 compared with normal subjects.
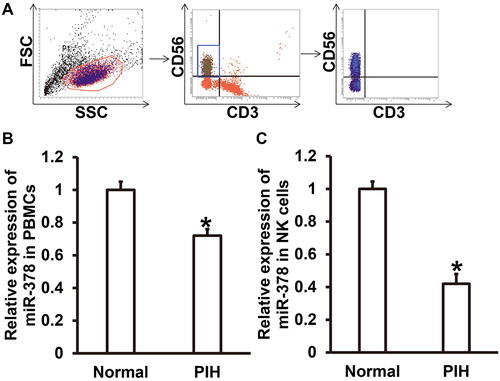
miR-378 negatively regulates the expression of CD226 in NK cells and CD226 is a target gene of miR-378
NK cells are the body's first line of defense against infections and tumours, and they are also the main effector cells of innate immunity [Citation33]. Abnormal immunity of NK cells is closely related to abortion in pregnant women [Citation34], suggesting that NK cells participate in the regulation of pregnancy. Bioinformatics prediction showed that activated receptor CD226 on the surface of NK cells was a potential target of miR-378 (). Then, NK cells were transfected with agomiR-378 or antagomiR-378. qRT-PCR showed that the expression of miR-378 in NK cells of the agomiR-378 group was significantly higher than that of the NC group (P < 0.05) (), whereas that of the antagomiR-378 group was significantly lower than that of the NC group (P < 0.05) (). Western blotting showed that the expression of CD226 protein in NK cells of the agomiR-378 group was significantly lower than that in the NC group (P < 0.05) (), whereas that of the antagomiR-378 group was significantly higher than that of the NC group (P < 0.05) (). Dual luciferase reporter assay showed that miR-378 directly bound with the 3’-UTR of CD226 mRNA (). These results suggest that miR-378 negatively regulates the expression of CD226 in NK cells and CD226 is a target gene of miR-378.
Figure 3. Connection between CD226 and miR-378. (A) Bioinformatics prediction of the wild-type and mutant binding sites of miR-378 in CD226 mRNA. (B-C) Relative expression of miR-378 in NK cells transfected with (B) agomiR-378 or (C) antagomiR-378. qRT-PCR was used to determine the expression of miR-378. *, P < 0.05 compared with negative control (NC). (D-E) Relative expression of CD226 protein in NK cells transfected with (D) agomiR-378 or (E) antagomiR-378. Western blotting was used to determine the expression of CD226 protein. *, P < 0.05 compared with NC. (F) Direct binding of miR-378 with its target gene CD226 identified by dual luciferase reporter assay. *, P < 0.05 compared with NC.
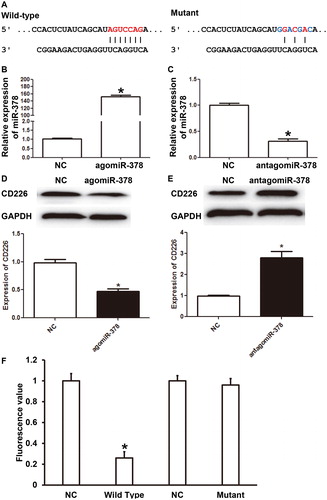
miR-378 inhibits the expression and secretion of soluble CD226 by NK cells
CD226 protein has two subtypes: one subtype is a transmembrane protein, an activated receptor on the surface of NK cells; the other subtype is soluble CD226 protein, which is involved in the regulation of proliferation and metastasis of tumor cells [Citation35,Citation36]. To measure the levels of soluble CD226 expressed and secreted by NK cells, flow cytometry and Western blotting were employed. Flow cytometry showed that the number of intracellular CD226+ NK cells in the agomiR-378 group was significantly lower than that in the NC group (P < 0.05) (), whereas that in the antagomiR-378 group was significantly higher than that in the NC group (P < 0.05) (). Western blotting showed that cytoplasmic CD226 level of NK cells in agomiR-378 group was significantly lower than that in NC group (P < 0.05) (), and that in antagomiR-378 group was significantly higher than that in NC group (P < 0.05) (). The results indicate that miR-378 inhibits the expression and secretion of soluble CD226 by NK cells.
Figure 4. Expression of soluble CD226 in NK cells after regulation by miR-378. (A-B) Flow cytometric analysis of the expression of soluble CD226 in NK cells transfected with (A) agomiR-378 or (B) antagomiR-378. (C-D) Expression of soluble CD226 in NK cells transfected with (C) agomiR-378 or (D) antagomiR-378 determined by Western blotting. *, P < 0.05 compared with negative control (NC) group.
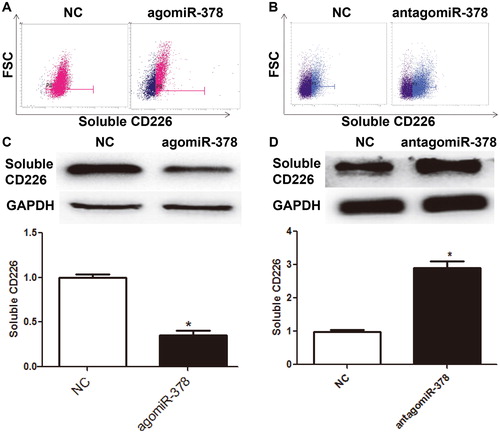
miR-378 regulates the proliferation, migration and apoptosis of HUVECs by affecting the levels of soluble CD226 expressed by NK cells
Persistent elevation of blood pressure in patients with PIH can lead to vascular injury, and injuries of vascular endothelial cells are the most common basic pathological changes in PIH [Citation37]. Vascular endothelial cells not only play a role of physical barrier in the maintenance of vascular function, but also have a strong secretory function, which plays an important role in regulating vascular pressure, thrombosis and chronic inflammation [Citation38,Citation39]. Proliferation and migration of vascular endothelial cells ensure structural and functional integrity of the cells, and they are regulated by gene expression of endothelial cells themselves and are also related to signalling molecules in the blood [Citation40]. To test whether soluble CD226 secreted by NK cells affects the biological functions of HUVECs, we co-cultured HUVECs with NK cells transfected by agomiR-378 or antagomiR-378. CCK-8 assay showed that the absorbance of HUVECs co-cultured with NK cells of the agomiR-378 group was significantly higher than that of the NC group at 48 h and 72 h (P < 0.05) (). By contrast, the absorbance of HUVECs co-cultured with NK cells of the antagomiR-378 group was significantly lower than that of the NC group at 48 h and 72 h (P < 0.05) (). Transwell assay showed that the migration ability of HUVECs co-cultured with NK cells of the agomiR-378 group was significantly increased than that of the NC group (P < 0.05) (), whereas the migration ability of HUVECs co-cultured with NK cells of the antagomiR-378 group was significantly decreased compared to that of the NC group (P < 0.05) (). Flow cytometry showed that the apoptotic rate of HUVECs co-cultured with NK cells of the agomiR-378 group was significantly reduced compared to that of the NC group (P < 0.05) (), whereas the apoptotic rate of HUVECs co-cultured with NK cells of the antagomiR-378 group was significantly higher than that of the NC group (P < 0.05) (). To verify that miR-378 exerted its effect via CD226, we added recombinant CD226 protein in the medium of the agomiR-378 group, and CD226 antibody in the medium of the antagomiR-378 group, as rescue groups. The data showed that recombinant CD226 protein and CD226 antibody reversed the effects of NK cells of the agomiR-378 and antagomiR-378 groups on the biological functions of HUVECs, respectively (). These results suggest that miR-378 regulates the proliferation, migration and apoptosis of HUVECs by affecting the levels of soluble CD226 expressed by NK cells.
Figure 5. Biological functions of HUVECs co-cultured with NK cells with up- or down-regulation of miR-378, and the effect of CD226. (A) Proliferation of HUVECs transfected with miR-NC or agomiR-378, or HUVECs transfected with agomiR-378 and cultured with recombinant CD226 protein (rescue group). *P < 0.05 compared with NC group. (B) Proliferation of HUVECs transfected with miR-NC or antagomiR-378, or HUVECs transfected with antagomiR-378 and cultured with CD226 antibody (rescue group). *P < 0.05 compared with NC group. (C) Absorbance of HUVECs transfected with miR-NC or agomiR-378, or HUVECs transfected with agomiR-378 and cultured with recombinant CD226 protein (rescue group). Transwell assay was used to evaluate migration ability. *P < 0.05 compared with NC group. (D) Absorbance of HUVECs transfected with miR-NC or antagomiR-378, or HUVECs transfected with antagomiR-378 and cultured with CD226 antibody (rescue group). Transwell assay was used to evaluate migration ability. *P < 0.05 compared with NC group. (E) Apoptotic rate of HUVECs transfected with miR-NC or agomiR-378, or HUVECs transfected with agomiR-378 and cultured with recombinant CD226 protein (rescue group). Flow cytometry was used to detect apoptosis. *P < 0.05 compared with NC group. (F) Apoptotic rate of HUVECs transfected with miR-NC or antagomiR-378, or HUVECs transfected with antagomiR-378 and cultured with CD226 antibody (rescue group). Flow cytometry was used to detect apoptosis. *P < 0.05 compared with NC group.
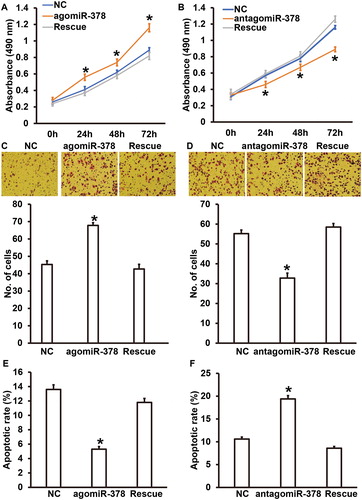
Conclusions
The present study demonstrated that the expression of miR-378 in peripheral blood and NK cells from patients with PIH was reduced as compared to normal subjects. miR-378 down-regulated the expression and release of soluble CD226 by NK cells and inhibited the injuries of vascular endothelial cells caused by CD226.
Ethical approval and consent to participate
All procedures performed in this study were approved by the Ethics Committee of The First People’s Hospital of Lanzhou City. Written informed consent was obtained from all patients or their families.
Consent for publication
Written informed consents for publication of any associated data and accompanying images were obtained from all patients or their parents, guardians or next of kin.
Acknowledgements
The authors wish to thank their department and research team for their help and dedication.
Disclosure statement
The authors declare that they have no competing interests.
Availability of data and materials
The datasets used and/or analysed during this study is available from the corresponding author upon reasonable request.
Additional information
Notes on contributors
Huiying Xu
The final version of the manuscript has been read and approved by all authors, and each author believes that the manuscript represents honest work. HX and YD collaborated to design the study. HX, YD, JH, LW and GS were responsible for performing experiments. HX and YD analyzed the data. All authors collaborated to interpret the results and develop the manuscript.
References
- Stavropoulos K, Sotiriadis A, Patoulias D, et al. Pseudohyperaldosteronism due to mumijo consumption during pregnancy: a licorice-like syndrome. Gynecol Endocrinol. 2018;34:1019–1021.
- Yin H, Zhao L, Lin Y, et al. Perinatal outcomes following labor induction with dinoprostone in pregnancies with borderline amniotic fluid index at term: A clinical observation study. J Obstet Gynaecol Res. 2018;44:1397–1403.
- Dominguez JE, Habib AS, Krystal AD. A review of the associations between obstructive sleep apnea and hypertensive disorders of pregnancy and possible mechanisms of disease. Sleep Med Rev. 2018;42:37–46.
- Guan J, Singh-Mallah G, Liu K, et al. The role for cyclic Glycine-Proline, a biological regulator of insulin-like growth factor-1 in pregnancy-related obesity and weight changes. J Biol Regul Homeost Agents. 2018;32:465–478.
- Hutcheon JA, Stephansson O, Cnattingius S, et al. Pregnancy weight gain before diagnosis and risk of Preeclampsia: A population-based cohort study in Nulliparous women. Hypertension. 2018;72:433–441.
- Arendt LH, Henriksen TB, Lindhard MS, et al. Hypertensive disorders of pregnancy and genital anomalies in boys: A Danish Nationwide Cohort Study. Epidemiology (Cambridge, Mass). 2018;29:739–748.
- Hobson SR, Gurusinghe S, Lim R, et al. Melatonin improves endothelial function in vitro and prolongs pregnancy in women with early-onset preeclampsia. J Pineal Res. 2018;65:e12508. [cited 2019 Apr 21].
- Han N, Li Y, Dong Y. Therapeutic effect of long-term epidural block in rats with pregnancy induced hypertension. Biomed Res Int. 2018;2018:1. [cited 2019 Apr 21]
- Wu C, Wang Y, Zhang W, et al. Inverse correlation between serum Adiponectin level and albuminuria in pregnancy-induced hypertension patients. Clin Exp Hypertens. 2019;41(3):263–267.
- Fossum S, Halvorsen S, Vikanes AV, et al. Cardiovascular risk profile at the age of 40–45 in women with previous hyperemesis gravidarum or hypertensive disorders in pregnancy: A population-based study. Pregnancy Hypertens. 2018;12:129–135.
- Zhang Q, Huang Y, Zhang K, et al. Progesterone attenuates hypertension and autoantibody levels to the angiotensin II type 1 receptor in response to elevated cadmium during pregnancy. Placenta. 2018;62:16–24.
- Tao J, Cong H, Wang H, et al. MiR-30a-5p inhibits osteosarcoma cell proliferation and migration by targeting FOXD1. Biochem Biophys Res Commun. 2018;503:1092–1097.
- Yao Q, Song Z, Wang B, et al. Emerging roles of microRNAs in the metabolic control of immune cells. Cancer Lett. 2018;433:10–17.
- Amaral AED, Rode MP, Cisilotto J, et al. MicroRNA profiles in serum samples from patients with stable cirrhosis and miRNA-21 as a predictor of transplant-free survival. Pharmacol Res. 2018;134:179–192.
- Shekhar S, Cunningham MW, Pabbidi MR, et al. Targeting vascular inflammation in ischemic stroke: Recent developments on novel immunomodulatory approaches. Eur J Pharmacol. 2018;833:531–544.
- Zhang H, Zheng J, Lin J, et al. miR-758 mediates oxLDL-dependent vascular endothelial cell damage by suppressing the succinate receptor SUCNR1. Gene. 2018;663:1–8.
- Yang J, Zeng P, Yang J, et al. MicroRNA-24 regulates vascular remodeling via inhibiting PDGF-BB pathway in diabetic rat model. Gene. 2018;659:67–76.
- Kim J, Hyun J, Wang S, et al. MicroRNA-378 is involved in hedgehog-driven epithelial-to-mesenchymal transition in hepatocytes of regenerating liver. Cell Death Dis. 2018;9:721.
- Yuan J, Liu H, Gao W, et al. MicroRNA-378 suppresses myocardial fibrosis through a paracrine mechanism at the early stage of cardiac hypertrophy following mechanical stress. Theranostics. 2018;8:2565–2582.
- Tong H, Jiang R, Liu T, et al. bta-miR-378 promote the differentiation of bovine skeletal muscle-derived satellite cells. Gene. 2018;668:246–251.
- Jiang L, Tang C, Gong Y, et al. PD-1/PD-L1 regulates Treg differentiation in pregnancy-induced hypertension. Braz J Med Biol Res. 2018;51:e7334.
- Zhao X, Wang X. Candesartan targeting of angiotensin II type 1 receptor demonstrates benefits for hypertension in pregnancy via the NF-κB signaling pathway. Mol Med Rep. 2018;18:705–714.
- Wang ZH, Sun SY, Chen QN, et al. The first-trimester maternal serum cyclophilin A concentrations in women with complicated pregnancy as preeeclampsia. Clin Chim Acta. 2018;484:105–110.
- Ventura NM, Li TY, Tse MY, et al. Developmental origins of pregnancy-induced cardiac changes: establishment of a novel model using the atrial natriuretic peptide gene-disrupted mice. Mol Cell Biochem. 2018;449:227–236.
- Dayan N, Schlosser K, Stewart DJ, et al. Circulating MicroRNAs implicate multiple atherogenic abnormalities in the long-term cardiovascular sequelae of preeclampsia. Am J Hypertens. 2018;31:1093–1097.
- Biro O, Alasztics B, Molvarec A, et al. Various levels of circulating exosomal total-miRNA and miR-210 hypoxamiR in different forms of pregnancy hypertension. Pregnancy Hypertens. 2017;10:207–212.
- Mei Z, Huang B, Mo Y, et al. An exploratory study into the role of miR-204-5p in pregnancy-induced hypertension. Exp Ther Med. 2017;13:1711–1718.
- Ding J, Huang F, Wu G, et al. MiR-519d-3p suppresses invasion and migration of trophoblast cells via targeting MMP-2. PLoS One. 2015;10:e0120321. [cited 2019 Apr 21]
- Gray C, Li M, Patel R, et al. Let-7 miRNA profiles are associated with the reversal of left ventricular hypertrophy and hypertension in adult male offspring from mothers undernourished during pregnancy after preweaning growth hormone treatment. Endocrinology. 2014;155:4808–4817.
- Miller ES, Grobman WA, Culhane J, et al. Antenatal depression, psychotropic medication use, and inflammation among pregnant women. Arch Womens Ment Health. 2018;21:785–790.
- Favero JF, Da Silva AS, Bottari NB, et al. Physiological changes in the adenosine deaminase activity, antioxidant and inflammatory parameters in pregnant cows and at post-partum. J Anim Physiol Anim Nutr (Berl). 2018;102:910–916.
- Yang SL, Niu TT, Li XL, et al. Bu-Shen-Yi-Qi formula impairs cytotoxicity of NK cells by up-regulating IDO expression in trophoblasts. Gynecol Endocrinol. 2018;34:675–679.
- Jung IH, Kim DH, Yoo DK, et al. In Vivo Study of Natural Killer (NK) cell cytotoxicity against Cholangiocarcinoma in a nude mouse model. In Vivo. 2018;32:771–781.
- Yougbare I, Tai WS, Zdravic D, et al. Activated NK cells cause placental dysfunction and miscarriages in fetal alloimmune thrombocytopenia. Nat Commun. 2017;8:224.
- Zhang Y, Liu T, Chen Y, et al. CD226 reduces endothelial cell glucose uptake under hyperglycemic conditions with inflammation in type 2 diabetes mellitus. Oncotarget. 2016;7:12010–12023.
- Xu Z, Zhang T, Zhuang R, et al. Increased levels of soluble CD226 in sera accompanied by decreased membrane CD226 expression on peripheral blood mononuclear cells from cancer patients. BMC Immunol. 2009;10:34. [cited 2019 Apr 21].
- Han S, Tan C, Ding J, et al. Endothelial cells instruct liver specification of embryonic stem cell-derived endoderm through endothelial VEGFR2 signaling and endoderm epigenetic modifications. Stem Cell Res. 2018;30:163–170.
- Xu C, Gao X, Wei Q, et al. Stem cell factor is selectively secreted by arterial endothelial cells in bone marrow. Nat Commun. 2018; [cited 2019 Apr 21]9:2449.
- Cazzaniga A, Locatelli L, Castiglioni S, et al. The contribution of EDF1 to PPARgamma transcriptional activation in VEGF-treated human endothelial cells. Int J Mol Sci. 2018;19:1830. [cited 2019 Apr 21].
- Gao H, Zhang Q, Chen J, et al. Porcine IL-6, IL-1beta, and TNF-alpha regulate the expression of pro-inflammatory-related genes and tissue factor in human umbilical vein endothelial cells. Xenotransplantation. 2018;25:e12408. [cited 2019 Apr 21]