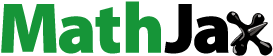
Abstract
Our previous study demonstrated that a new Bacillus subtilis AD35 was the first Bacillus sp. so far possessing the capability to produce the antibacterial compound di-(2-ethylhexyl) phthalate (DEHP) [Lotfy WA, Mostafa SW, Adel AA, et al. Production of DEHP by Bacillus subtilis AD35: isolation, purification, characterization and biological activities. Microb Pathog. 2018;124:89–100]. In this study, we investigate the process parameters influencing DEHP production by B. subtilis AD35. The best culture medium, carbon and nitrogen source that significantly affected DEHP synthesis were beef-yeast-peptone medium, starch and L-asparagine, respectively. A fractional factorial design (FFD) was applied to screen the fermentation conditions affecting DEHP formation. Agitation rate, inoculum size and sodium chloride concentration were found to be the major factors affecting the synthesis of DEHP. Sequentially, response surface methodology (RSM) was implemented and a polynomial model was developed to predict maximum DEHP activities against Escherichia coli, methicillin-resistant Staphylococcus aureus (MRSA), Salmonella typhimurium, Staphylococcus aureus, Staphylococcus epidermidis, Listeria monocytogenes, Pseudomonas aeruginosa and Aeromonas hydrophila. At optimized conditions, agitation rate, 220 rpm; inoculum size, 0.075% (v/v) and sodium chloride concentration, 0.75% (w/v), DEHP activity markedly increased to 24.7 AU, which represents an approximately 707% increase when compared to the pre-optimized conditions. The work reported here represents the first optimization of the process parameters influencing DEHP synthesis by B. subtilis AD35.
Introduction
The occurrence of antibiotic-resistant bacteria increases the need for exploring new sources of antimicrobial agents. Our previous study demonstrated that a new Bacillus subtilis AD35 was the first Bacillus sp. so far possessing the capability to produce the antibacterial compound di-(2-ethylhexyl) phthalate (DEHP) [Citation1]. This is of particular interest, as multidrug-resistant pathogens represent a severe worldwide problem [Citation2]. Bacteria are a permanent source of bioactive compounds. B. subtilis strains produce more than 70 diverse antimicrobials [Citation3] including ribosomally or non-ribosomally synthesized peptides [Citation4]. Bacterial synthesis of antibiotics depends on the producer strain, formulation of fermentation medium and the biosynthesis conditions [Citation5]. Slight variations in these factors can significantly affect the antibiotic yield. Moreover, the biosynthesis of antibiotics by Bacillus strains is considerably affected by alterations in the type and concentration of carbon or nitrogen sources [Citation6]. Hence, the maximal production of the synthesized antibiotics depends on the type of carbon and nitrogen sources utilized by the producer strain [Citation7, Citation8].
Following our previous work on DEHP production by a B. subtilis marine isolate [Citation1], here, we were interested in optimizing the process parameters shaping the production of DEHP by B. subtilis AD35 under submerged fermentation. Optimization through fractional factorial design (FFD) and response surface methodology (RSM) is a common practice for quick screening and estimating the levels of variables giving maximum response using a mathematical model [Citation9–11]. Here, we adopted FFD and RSM to boost the DEHP synthesis by B. subtilis AD35. To the best of our knowledge, this is the first report to describe the optimization of DEHP production by B. subtilis. This investigation will be useful for the development of the B. subtilis cultivation process for efficient bulk-scale production of DEHP.
Materials and methods
Producer strain
The producer strain used throughout this study was taxonomically characterized as B. subtilis [Citation1] and has been deposited in the Egyptian Microbial Culture Collection Network, Alexandria, Egypt (Accession Number: EMCCN 4033).
Indicator pathogens
Escherichia coli (ATCC 8739), methicillin-resistant Staphylococcus aureus (MRSA: ATCC 43300), Salmonella typhimurium (ATCC 14028), Staphylococcus aureus (ATCC 6538), Staphylococcus epidermidis (ATCC 12228), Listeria monocytogenes (ATCC 19111), Pseudomonas aeruginosa (ATCC 9027) and Aeromonas hydrophila (ATCC 35654) were used as indicator pathogens in this study.
Media
The media used throughout this work were supplied from LAB M, England and were prepared according to the manufacturer’s instructions. The following media (g/L) were used throughout the present work. Nutrient Agar (NA): Peptone, 5; yeast extract, 3; beef extract, 1; sodium chloride, 5; and agar, 20. NA medium was used to prepare the seed inoculum. Nutrient Broth (NB): yeast extract, 3; peptone, 5; beef extract, 1; and sodium chloride, 5. Müller-Hinton Agar (MHA): beef extract, 2; casein hydrolysate, 17.5; starch, 1.5; and agar, 17. Beef-Yeast-Peptone (BYP): yeast extract, 3; peptone, 5; beef extract, 3; and sodium chloride, 3. Peptone-Yeast (PY): yeast extract, 3; peptone, 5; and sodium chloride, 3. Luria-Bertani (LB): yeast extract, 10; peptone, 5; and sodium chloride, 10.
Chemicals
All chemicals used in the present work were of analytical grade. Agar, glucose, sucrose, dextrose, maltose, galactose, lactose, glycerol, starch, sodium nitrate and urea: products of El-Nasr Pharmaceutical and Chemicals Company, El Obour, Egypt. Ammonium sulfate and ammonium nitrate: products of SD fine-Chem limited, Mumbai, India. L-asparagine: a product of Loba-Chemie Company, Mumbai, India.
Antimicrobial activity of DEHP produced by B. subtilis AD35
The agar diffusion method was used to test the ability of DEHP to inhibit the growth of indicator bacteria as reported previously [Citation1].
Factors affecting the production of DEHP
Effect of fermentation medium
B. subtilis AD35 was grown on LB, PY and BYP media. The strain was incubated in liquid culture media at 30 °C under shaking at 120 rpm for 14 h, and the cell-free supernatant of each culture was tested against indicator test pathogens using the agar diffusion assay; NB medium was used as a control.
Effect of carbon sources
Various carbon sources viz. glucose, sucrose, dextrose, maltose, galactose, lactose, glycerol, starch, corn and bran were used to study their effect on the synthesis of DEHP. These substrates were added separately, at a concentration of 1% (w/v), to the fermentation medium [Citation12]. After incubation for 14 h at 30 °C under shaking at 120 rpm, the antimicrobial activities of the cell-free supernatants were tested and NB medium was used as a control.
Effect of nitrogen sources
Various nitrogen sources namely sodium nitrate, ammonium nitrate, ammonium sulfate, urea and L-asparagine were used to assess their effect on the production of DEHP. These substrates were added separately, at a concentration of 1% (w/v), to the fermentation medium [Citation12]. After incubation for 14 h at 30 °C under shaking at 120 rpm, the antimicrobial activities of the cell-free supernatants were tested and NB medium was used as a control.
Optimization of the fermentation conditions
Optimization was performed on two sequential phases; the first phase was designed to evaluate the significance of fermentation parameters and various medium constituents on DEHP production by applying a FFD. In the second phase, levels of variables, which had significant influence on the production process, were further studied using an RSM design.
An FFD, the Plackett-Burman design [Citation13, Citation14], was applied to reflect the relative impact of various cultural and process parameters for higher DEHP synthesis in liquid cultures. Seven independent variables were screened at two levels, a high (+) and low (−) level. Nine trials were performed in triplicate and the average of the observation results was considered as the response. Statistica 10 software was used to determine the main effect and the significance of each variable.
The Box-Behnken design, an RSM, was adopted to determine the optimal levels of the most significant variables with respect to DEHP production [Citation15]. This optimization process involved performing the designed experiments, estimating the coefficients in a mathematical model, predicting the response and checking the adequacy of the model. The low, basal and high levels of each variable were designated as −1, 0 and +1, respectively. A design matrix of 15 combinations was constructed, all trials were carried out in triplicate and mean values of DEHP synthesis were calculated. Statistica 10 software was used for the regression analysis of the experimental data obtained [Citation15, Citation16], estimation of t-values, p-values, optimum values and the effects of the independent factors on the response. Experiments were performed in triplicate and mean values were given.
Results and discussion
Antibiotic-resistant bacteria apparently increased the need for discovery of new antibacterials. Recently [Citation1], we demonstrated that a new B. subtilis isolate was a potent producer of an antibacterial compound which was structurally elucidated as DEHP. The present work aimed to optimize the bioprocess of DEHP synthesis by B. subtilis AD35.
Time course of DEHP synthesis
Our previously published data [Citation1] showed that the stationary phase of B. subtilis AD35 (12–24 h) lasted for approximately 12 h and the formation of DEHP increased significantly between 10 and 18 h. The highest DEHP activity (3.5 AU) was recorded against S. typhymurium after 15 h. On the other hand, throughout the stationary phase the lowest antimicrobial activity (0.45 AU) was observed against A. hydrophila.
Factors affecting the production of DEHP by B. subtilis AD35
Effect of growth medium
Synthesis of DEHP by B. subtilis AD35 started at the early stages of the stationary phase. Therefore, it was important to limit the cellular growth by proper formulation of culture medium ingredients, so that it favors DEHP formation. Consequently, B. subtilis AD35 was grown on LB, PY and BYP media and was tested for its antagonistic effect against the test pathogens. illustrated that BYP was the most promising medium for higher activity of DEHP by a 1.2–3.71 fold increase followed by LB medium with 1.2–3.4 fold increase when compared to the control. On the other hand, PY medium demonstrated the lowest DEHP activity against the tested pathogens with about 0.63–0.9 fold decrease in DEHP activity compared to the control and no antibacterial activity against MRSA. According to these results, BYP was chosen as the experimental medium in this study on the basis of maximum DEHP activity [Citation17].
Figure 1. Effect of medium type on the activity of DEHP produced by B. subtilis AD35.
LB; Luria-Bertani medium, PY; Peptone-Yeast extract medium, BYP; Beef-Yeast-Peptone medium.
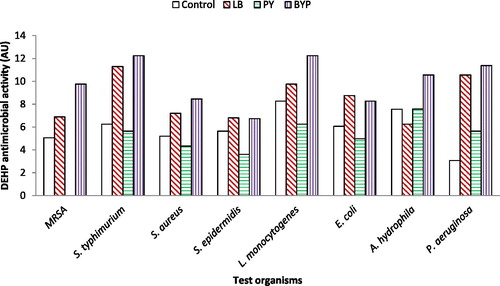
This is in accordance with the findings of Fang et al. [Citation18], who performed a comparison between different media for enhancing the antibiotic activity and found that tryptone soya broth medium was the superior. In this context, the formulation of both tryptone soya broth and BYP broth include complex organic nitrogen sources and sodium chloride. Our data also verified the previous reports by Maxwell et al. [Citation19] and Ji et al. [Citation20] regarding the high productivity of fermentation media that contain complex organic nitrogen sources. Chen [Citation21] suggested that the use of different media may change the levels of the expressed antibiotic activity.
Effect of carbon and nitrogen sources
The type and concentration of carbon or nitrogen sources have impact on the biosynthesis of antibiotic by Bacillus strains [Citation6]. Thus, the effect of various carbon and nitrogen sources on the activity of DEHP produced by B. subtilis AD35 was investigated. Various carbon sources viz. glucose, sucrose, dextrose, maltose, galactose, lactose, glycerol, starch, bran and corn were added by equivalent amounts at a concentration of 1% (w/v) to BYP medium, one at a time. illustrated that DEHP production was affected by the nature of the carbon source differently. Starch and dextrose were the most preferable carbon sources yielding a DEHP activity with 1.55–1.68 and 1.32–1.52 fold increase, respectively, when compared to the control. On the other hand, the lowest DEHP activity was obtained in cultures containing glycerol and bran as a sole carbon source with 0.14–0.35 and 0.12–0.38 fold decreases in antimicrobial activity, respectively compared to control. According to the obtained results, the best DEHP activity was obtained with culture containing starch as a sole carbon source. Consequently, starch was chosen for the following experiments. Similar results were obtained in other studies, a higher antibiotic yield was obtained with a slowly utilizable carbohydrate because of its availability in adequate amount during biomass growth and antibiotic production [Citation22–24].
On the other hand, various nitrogen sources, namely, sodium nitrate, ammonium sulfate, ammonium nitrate, urea and L-asparagine, were added by equivalent amounts at a concentration of 1% (w/v) to BYP medium, one at a time. illustrated that DEHP activity was affected by the type of nitrogen source differently. L-asparagine was the most preferable nitrogen source with 1.05–1.15 fold increase in DEHP activity compared to the control. On the other hand, the lowest antimicrobial activity was obtained in the cultures containing ammonium sulfate as a nitrogen source with 0.14–0.54 fold decrease compared to the control. No antimicrobial activity was detected by B. subtilis AD35 grown on BYP medium containing sodium nitrate or ammonium nitrate as a nitrogen source. Based on the previous results, the best antimicrobial activity was obtained with the culture broth containing L-asparagine as a nitrogen source. Therefore, L-asparagine was chosen as the best nitrogen source in our subsequent experiments.
Figure 3. Influence of different nitrogen sources on the activity of DEHP produced by B. subtilis AD35.
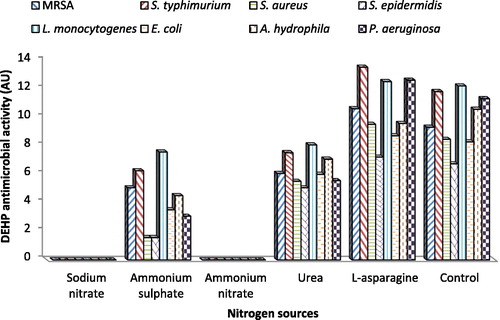
Our results are in accordance with the findings reported by Kadiri and Yarla [Citation25]. They stated that L-asparagine was the best nitrogen source for the production of antimicrobial metabolites by Streptomyces fradiae. However, a previous study found that DL-methionine was the optimal nitrogen source for antibiotic production by B. circulans [Citation22]. Pandey et al. [Citation26] examined a number of carbon and nitrogen sources for their effect on the production of an antibacterial antibiotic by Streptomyces kanamyceticus M 27. They demonstrated that the most suitable carbon and nitrogen sources were dextrose and yeast extract, respectively. Another study [Citation27] reported that glucose and ammonium nitrate in synthetic medium gave a high yield of polyketide antibiotic SBR-22 produced by a marine Streptomyces species. These disparities may be due to the use of different strains, culture conditions or fermentation media for nitrogen and carbon source selection.
Optimization of DEHP production by B. subtilis AD35
The nutritional and environmental conditions have a great influence on the activity of DEHP. To achieve maximum DEHP activity, the factors affecting the production process need to be well identified. Experimental designs are excellent techniques for optimization of fermentation conditions to obtain optimal levels of desired compounds [Citation9, Citation11, Citation28]. Optimization was performed by a two-phase experimental design. The first phase was to evaluate the significance of different factors on the antibacterial activity of DEHP by applying an FFD. In the second phase, the levels of the significant factors were further studied using a RSM.
Evaluating the significance of fermentation conditions using the Plackett-Burman design
The Plackett-Burman experimental design was used to evaluate the relative impact of various factors on the activity of DEHP in liquid BYP cultures. The chosen levels of 7 variables were presented in and the design was applied with 9 different fermentation conditions as shown in . The response, DEHP antimicrobial activity (AU), was calculated for each trial () and the main effect of each variable on the activity of DEHP was graphically presented in . The results indicated that the agitation rate, pH and temperature had a positive effect on the antimicrobial activity of DEHP against MRSA and S. typhimurium, whereas the other tested variables showed negative effects. Starch, L-asparagine and the inoculum size had a positive effect on the activity of DEHP against S. aureus, L. monocytogens and P. aeruginosa, while the other tested variables exhibited negative effects. Starch, agitation rate and pH had positively affected the antimicrobial activity of DEHP against S. epidermidis and A. hydrophila, whereas the other tested variables demonstrated negative effects. On the other hand, starch, L-asparagine and agitation rate have positive effect on the activity of DEHP against E. coli, whereas the other tested variables showed negative effects.
Table 1. Levels of the independent variables applied in the Plackett-Burman design.
Table 2. The Plackett-Burman experimental design matrix for 7 variables.
Table 3. Experimental results of the Plackett-Burman experimental design.
P-values, t-values and significance levels were estimated for each independent variable as shown in . In the range of the examined levels of variables, it is apparent that the agitation rate, inoculum size and sodium chloride concentration had the most significant effects on the antimicrobial activity of DEHP produced by B. subtilis AD35.
Table 4. Statistical analysis of the Plackett-Burman experimental results.
Decreasing the level of sodium chloride was advantageous for DEHP activity, which was in keeping with previous results reported by El-Sersy et al. [Citation28]. On the other hand, this finding contradicted with the outcomes of Ji et al. [Citation29], who found that soybean meal, corn powder and incubation temperature were the most significant factors identified by FFD to affect sublancin production by B. subtilis. It is conceivable that this may be due to the difference in the biosynthetic pathway of DEHP and sublancin. The latter is biosynthesized as a precursor peptide bearing an N-terminal leader peptide and a C-terminal core peptide [Citation30].
Based on the results of the Plackett-Burman experiment, the following fermentation conditions were expected to be close to optimal levels: temperature, 28.75 °C; pH, 7.75; agitation rate, 170 rpm; inoculum size, 0.1%; starch, 2%; L-asparagine, 1% and sodium chloride, 1%.
Optimization of fermentation conditions by applying Box-Behnken design
The Box-Behnken design, an RSM, was applied to determine the optimal level of each key factor, as identified by the Plackett-Burman experiment. The factors included in the model were; agitation rate (X1), inoculum size (X2) and sodium chloride (X3). As shown in , the design matrix comprised 15 trials, each factor being examined at three different levels (−, 0 and +), and the observed antimicrobial activities (AU) were calculated.
Table 5. Box-Behnken design for the antimicrobial activity of DEHP produced by B. subtilis AD35.
Based on the value of R2 (0.99769), the performance of the experimental data was fitting. The statistical analysis of the design showed a high model precision, which reflects a high degree of proximity between predicted and experimental data. Verifying the accuracy of model prediction revealed low residual values as shown in , the predicted and observed optimized antimicrobial activities (AU) were closely related to each other.
Figure 5. Residual diagnostics of quadratic model surface. (A) Residuals versus predicted response, (B) The predicted response versus observed antimicrobial activity of DEHP produced by B. subtilis AD35, and (C) Residuals versus observed antimicrobial activity of DEHP produced by B. subtilis AD35.
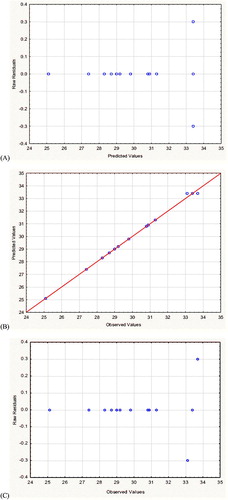
The experimental response results were fitted mathematically with the help of Statistica 10 software and the following second order polynomial function was generated.
Where, Y is the response, DEHP antimicrobial activity (AU), and X1, X2 and X3 are the coded values of the independent variables, viz. NaCl concentration (%), agitation rate (rpm) and inoculum size (%), respectively.
An optimum response was determined by the help of Statistica 10 software () at the following levels: agitation rate, 220 rpm; inoculum size, 0.075% (v/v) and sodium chloride 0.75% (w/v) with a predicted DEHP antimicrobial activity of 33.4 AU. These optimum conditions raised the average antimicrobial activity of DEHP by an 1.98-fold increase when compared to the basal conditions before applying the Box-Behnken experiment.
A three-dimensional plot of the polynomial equation was generated with the help of Statistica 10 software as illustrated in . described the effects of agitation rate and sodium chloride concentration on the antimicrobial activity of DEHP (AU) and the maximum activity was clearly located at 220 rpm and 0.75%, respectively. demonstrated the response surface plot for the activity of DEHP (AU) as a function of inoculum size with sodium chloride concentration and with agitation rate, respectively. A positive effect of sodium chloride concentration and agitation rate can be observed with a maximum DEHP activity (AU) obtained at inoculum size concentration of 0.075%.
Figure 7. The response surface plot of B. subtilis AD35 DEHP antimicrobial activity (AU) as a function of (A) NaCl concentration (%) and agitation rate (rpm), (B) NaCl concentration (%) and inoculum size (%), and (C) agitation rate (rpm) and inoculum size (%).
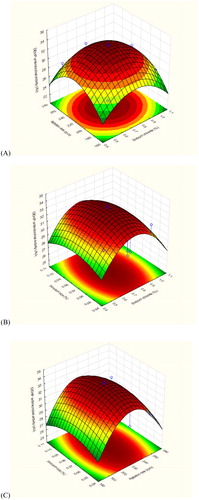
An experiment was performed under the predicted optimal conditions for model validation, where the activity of DEHP (AU) was measured. The basal culture condition, before applying the Box-Behnken experiment, was used as a control. Furthermore, a reverse state to the optimized fermentation condition was applied for comparison. The results from the validation experiment revealed that the average activity of DEHP produced by B. subtilis AD35 rose up to 24.7 AU, which represents a 1.98, 2.6 and 7.07 fold increase when compared to the basal, anti-optimized and pre-optimized condition (3.5 AU), respectively ().
Figure 8. Antimicrobial activity of DEHP produced by B. subtilis AD35 grown under basal, anti-optimized and optimized fermentation conditions in comparison to predicted optimized conditions against S. aureus (A), E. coli (B), P. aeruginosa (C), L. monocytogenes (D), S. epidermidis (E), MRSA (F), S. typhimurium (G) and A. hydrophila (H).
BC, basal conditions; AOC, anti-optimized conditions; POC, predicted optimized conditions; OC, optimized conditions
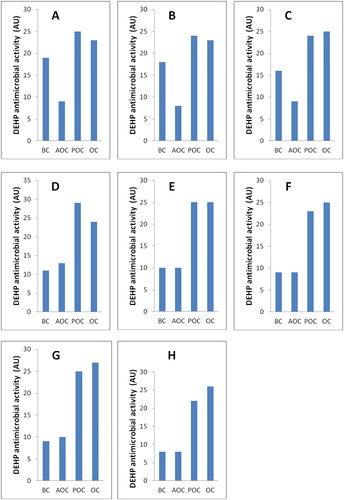
Our results clearly demonstrated an adequate approximation of the fitted model generated by the Box-Behnken design based on the validation experiment in addition to the value of the regression coefficient and the parity plots. Our results also strongly support the use of RSM for optimization of DEHP production by B. subtilis AD35. The adopted optimization approach not only resulted in a 707% increase of antibiotic activity, but also was accurate and less time consuming.
Conclusions
B. subtilis strain AD35 was the first Bacillus sp. possessing the capability to synthesize DEHP. Therefore, this study was conducted to demonstrate some factors affecting the production of DEHP. To the best of our knowledge, there are no reports on optimization of DEHP synthesis by B. subtilis. This work also demonstrates the importance of knowledge for the development of efficient industrial scale production of DEHP by B. subtilis.
Disclosure statement
No potential conflict of interest was reported by the authors.
Funding
This research did not receive any specific grant from funding agencies in the public, commercial, or not-for-profit sectors.
References
- Lotfy WA, Mostafa SW, Adel AA, et al. Production of di-(2-ethylhexyl) phthalate by Bacillus subtilis AD35: Isolation, purification, characterization and biological activities. Microb Pathog. 2018;124:89–100.
- WHO. Antibacterial agents in clinical development: an analysis of the antibacterial clinical development pipeline, including tuberculosis. Geneva: WHO; 2017. Available from: https://apps.who.int/iris/bitstream/handle/10665/258965/WHO-EMP-IAU-2017.11-eng.pdf;jsessionid=705615951D7018500631F482E1447740?sequence=1.
- Todorova S, Kozhuharova L. Characteristics and antimicrobial activity of Bacillus subtilis strains isolated from soil. World J Microbiol Biotechnol. 2010;26:1207–1216.
- Stein T. Bacillus subtilis antibiotics: structures, syntheses and specific functions. Mol Microbiol. 2005;56:845–857.
- Moita C, Feio SS, Nunes L, et al. Optimisation of physical factors on the production of active metabolites by Bacillus subtilis 355 against wood surface contaminant fungi. Int Biodeter Biodeg. 2005;55:261–269.
- Grahovac J, Rončević Z, Tadijan I, et al. Optimization of media for antimicrobial compounds production by Bacillus subtilis. Acta Aliment. 2015;44:427–435.
- El-Banna NM. Effect of carbon source on the antimicrobial activity of the air flora. World J Microbiol Biotechnol. 2005;21:1451–1454.
- El-Banna NM, Quddoumi SS. Effect of nitrogen source on the antimicrobial activity of the bacilli air flora. Ann Microbiol. 2007;57:669–671.
- Lotfy WA, Abd-El-Karim NM, El-Sharouny EE, et al. Isolation and characterization of a haloalkaliphilic protease producer bacterium from Wadi Natrun in Egypt. Afri J Biotechnol. 2017;16:1210–1220.
- Lotfy WA, Atalla RG, Sabra WA, et al. Expression of extracellular polysaccharides and proteins by clinical isolates of Pseudomonas aeruginosa in response to environmental conditions. Int Microbiol. 2018;21:129–142.
- Lotfy WA, Ghanem KM, Ehab R. Citric acid production by a novel Aspergillus niger isolate: II. Optimization of process parameters through statistical experimental designs. Bioresour Technol. 2006;11:32–40.
- Sharon SFB, Daniel RR, Shenbagarathai R. Optimization of antibiotic production by marine actinomycetes Streptomyces sp. KOD10 S. Int J Pharm Sci. 2014;6:506–510.
- Plackett RL, Burman JP. The design of optimum multifactorial experiments. Biometrika 1946;33:305–325.
- Yu X, Hallett S, Sheppard J, et al. Application of the Plackett-Burman experimental design to evaluate nutritional requirements for the production of Colletotrichum coccodes spores. Appl Microbiol Biotechnol. 1997;47:301–305.
- Box GE, Behnken DW. Some new three level designs for the study of quantitative variables. Technometrics 1960;2:455–475.
- Ashengroph M, Saedi D. Optimization of selenite removal by Lactobacillus sp. Tra cheese 6 using Box-Behnken design. J Kerman Univ Med Sci. 2016;23:466–482.
- Khan MN, Lin H, Li M, et al. Identification and growth optimization of a marine Bacillus DK1-SA11 having potential of producing broad spectrum antimicrobial compounds. Pak J Pharm Sci. 2017;30:839–853.
- Fang X, Feng J, Zhang W, et al. Optimization of growth medium and fermentation conditions for improved antibiotic activity of Xenorhabdus nematophila TB using a statistical approach. Afri J Biotechnol. 2010;9:8068–8077.
- Maxwell PW, Chen G, Webster JM, et al. Stability and activities of antibiotics produced during infection of the insect Galleria mellonella by two isolates of Xenorhabdus nematophilus. Appl Environ Microbiol. 1994;60:715–721.
- Ji D, Yi Y, Kang GH, et al. Identification of an antibacterial compound, benzylideneacetone, from Xenorhabdus nematophila against major plant-pathogenic bacteria. FEMS Microbiol Lett. 2004;239:241–248.
- Chen G. Antimicrobial activity of the nematode symbionts, Xenorhabdus and Photorhabdus (Enterobacteriaceae), and the discovery of two groups of antimicrobial substances, nematophin and xenorxides (PhD Thesis). Department of Biological Sciences, Simon Fraser University; Burnaby, 1996.
- Abada EA, El-Hendawy HH, Osman ME, et al. Antimicrobial activity of Bacillus circulans isolated from rhizosphere of Medicago sativa. Life Sci J. 2014;11:711–719.
- El Batal A, Mona S. Al-Tamie M. Biosynthesis of gold nanoparticles using marine Streptomyces cyaneus and their antimicrobial, antioxidant and antitumor (in vitro) activities. J Chem Pharm Res. 2015;7:1020–1036.
- Majumdar MK, Majumdar S. Utilization of carbon and nitrogen-containing compounds for neomycin production by Streptomyces fradiae. Appl Microbiol. 1967;15:744–749.
- Kadiri SK, Yarla NS. Optimization of antimicrobial metabolites production by Streptomyces fradiae. Int J Pharm Pharm Sci. 2016;7:223–225.
- Pandey A, Shukla A, Majumdar S. Utilization of carbon and nitrogen sources by Streptomyces kanamyceticus M 27 for the production of an antibacterial antibiotic. Afri J Biotechnol. 2005;4:909–910.
- Sujatha P, Raju KB, Ramana T. Studies on a new marine streptomycete BT-408 producing polyketide antibiotic SBR-22 effective against methicillin resistant Staphylococcus aureus. Microbiol Res. 2005;160:119–126.
- El-Sersy NA, Ebrahim HA, Abou-Elela GM. Response surface methodology as a tool for optimizing the production of antimicrobial agents from Bacillus licheniformis SN 2. Curr Res Bacteriol. 2010;3:1–14.
- Ji S, Li W, Xin H, et al. Improved production of sublancin 168 biosynthesized by Bacillus subtilis 168 using chemometric methodology and statistical experimental designs. BioMed Res Int. 2015;2015:1–9.
- Oman TJ, Boettcher JM, Wang H, et al. Sublancin is not a lantibiotic but an S-linked glycopeptide. Nat Chem Biol. 2011;7:78–80.