Abstract
Introducing resistance (R) genes into a susceptible rice cultivar is an effective strategy to achieve broad-spectrum and durable disease resistance. During the breeding process, marker-assisted selection (MAS) provides an efficient approach for direct screening of the progeny containing the R gene in a population. In this study, we designed an optimized polymerase chain reaction marker, Pi9/2, targeting the same polymorphic region of our previously developed Pi9-Pro and demonstrated that Pi9/2 is more specific than Pi9-Pro for the blast resistance genes Pi9 and Pi2 in MAS. With the assistance of Pi9/2, Pi9 and Pi2, from donor lines 75-1-127 and C101A51, respectively, were separately introgressed into the strong rice restorer line, Hui 316, by backcrossing. The two improved representative Hui 316 lines, Hui 316-Pi9 and Hui 316-Pi2, displayed similar agronomic traits to Hui 316. Inoculation tests for blast resistance revealed that Hui 316-Pi9 and Hui 316-Pi2 showed markedly enhanced resistance to blast fungi compared with Hui 316. Investigation of agronomic traits for yield and rice quality indicated that the hybrid combinations from Hui 316-Pi9 or Hui 316-Pi2 crossed with male sterility lines were similar to those derived from Hui 316. Therefore, introgression of Pi9 or Pi2 into with Hui 316 could improve its resistance to blast with little influence on its restoring ability, which will facilitate the application of Hui 316 in rice production. These results also indicate that the optimized marker, Pi9/2, is effective in introducing Pi9 or Pi2 by MAS to improve blast resistance of rice cultivars.
Introduction
Rice (Oryza sativa) is food for more than half of the world’s population. Rice blast disease, caused by the fungal pathogen Magnaporthe oryzae, results in a yearly loss of 10–30% rice production, which is enough to feed at least 60 million people [Citation1, Citation2]. Therefore, rice blast disease is regarded as one of the most serious threats to global food security. The most effective and environmentally friendly approach to prevent and control rice blast is planting rice cultivars resistant to blast isolates [Citation3]. However, new virulent blast isolates continually present in the field, causing rice lines carrying resistance (R) genes to become susceptible after a few years of cultivation [Citation4]. This situation is more severe in areas growing hybrid rice because of the narrow genetic base of hybrid cultivars and excessive application of nitrogen fertilizer. One strategy to achieve long-lasting disease resistance is broadening the spectrum of resistance by pyramiding multiple R genes into a single cultivar.
In general, the traditional phenotype-based rice breeding strategy is inefficient and inaccurate in screening the desired resistant progeny under field conditions because disease incidence is easily affected by many environmental factors. Molecular markers based on the polymorphisms of genomic sequences have been widely developed and used in resistance breeding [Citation5–11]. The utilization of marker-assisted selection (MAS) provides an efficient approach for direct identification of the R genes controlling the resistance phenotype in a population, which can overcome the limitations of conventional breeding [Citation12].
At present, 35 R genes have been cloned and characterized from approximately 100 blast resistance genes in O. sativa subspecies indica and japonica [Citation13]. With the exception of Pi-d2 and pi21, which encode a receptor-like protein kinase (RLK) protein and a proline-rich protein, respectively, the majority of these R genes encode nucleotide-binding site (NBS)-leucine rich repeat (LRR) proteins (NBS-LRR) [Citation14, Citation15]. The Pi2/9 locus is a cluster of tandemly repeated NBS-LRR genes on the short arm of chromosome 6, containing at least nine blast-resistance genes. The Pi2 gene was first introgressed from a highly resistant indica cultivar, 5173, into CO39 (a highly susceptible indica cultivar), and the derived isogenic line displayed broad-spectrum blast resistance [Citation16, Citation17]. The resistance gene, Pi9, was introduced into cultivated rice from a tetraploid wild rice, Oryza minuta, and its introgression line showed even broader spectrum blast resistance than the line with Pi2 in the Philippines [Citation18]. Recently, Pi9 and Pi2 have been widely utilized in the genetic improvement of rice cultivars for enhanced blast resistance [Citation19–21]. Our previously developed molecular marker, Pi9-Pro, could differentiate the different genotypes corresponding to the Pi9, Pi2 and non-Pi2/Pi9 alleles by polymerase chain reaction (PCR); this marker has also been validated to co-segregate with the blast resistance phenotype in populations [Citation22].
In the three-line breeding system, a cytoplasmic male sterility line is crossed with a restorer line to produce F1 hybrid seeds, which are subsequently used for rice production. It is widely accepted that the restorer line is the key donor parent to further improve the resistance, quality and heterosis of hybrid rice [Citation23]. Hui 316 is a newly bred strong rice restorer for three-line hybrid rice production, which possesses many advantages, such as strong restoring and combining ability as well as high yield. However, Hui 316 lacks broad-spectrum blast resistance, which could limit its popularization in hybrid rice production.
In this study, using the optimized PCR marker Pi9/2, targeting the same polymorphic region of Pi9-Pro, we individually introgressed Pi9 and Pi2 into Hui 316 by MAS. The produced lines were subjected to inoculation with blast fungi under both greenhouse and field conditions to determine whether their resistance had been improved. With the investigation into yield traits and rice quality of the hybrid combinations, we concluded that the improved rice restorer lines conferred markedly enhanced resistance to blast with little influence on their restoring ability.
Materials and methods
Rice materials and blast isolates
The blast R-gene donors 75-1-127 (carrying Pi9) and C101A51 (carrying Pi2) were provided by Dr. Guo-Liang Wang’s lab at Ohio State University. Hui 316 was derived from the crossing of Shuhui 881 and Lianhui 9-9. The 21 M. oryzae isolates used in this study (JY15015, NH17028, SM17211, SH17004, JL15140, NH13093, NH17267, SM17192, JY14027, NH15039, SM17212, NH15040, NH17026, NH17268, SM17203, NH17029, NJ08022, SM17207, SM17191, NH17025 and NH15092) were collected in Fujian Province, China.
Evaluation of disease incidence in greenhouse and field conditions
Rice seedlings were grown in the greenhouse for approximately 3 weeks (until they had three to four leaves) at 28–30 °C and natural light. Spray-inoculation was performed with M. oryzae spores at the concentration of 5 × 105 spores/mL. After inoculation, the seedlings were maintained in the dark for 24 h at 28 °C and then kept under high humidity (80–90%) for approximately 5–7 days to evaluate symptoms. The standard 0-5 scale was used to score disease incidence [Citation24]. The plants with lesion scores of 0, 1, and 2 were considered as resistant (R); the plants with lesion scores of 3, 4 and 5 were considered as moderately resistant (MR), moderately susceptible (MS) and susceptible (S), respectively. For evaluation of blast in the field trial, 75-1-127, C101A51, Hui 316 and the improved lines were grown at the station for testing blast-resistance in Shanghang, Fujian Province, China, in both 2016 and 2017. Disease evaluation was carried out on leaves and spikes at seedling and mature stages, respectively.
DNA extraction and sequence comparison
Leaf tissue was collected for DNA extraction. First, leaf samples were frozen in liquid nitrogen, then shocked into fine powder using a TissueLyser (Qiagen, Haan, Germany). Genomic DNA was extracted from ground tissue using the CTAB method. PCR amplification using the primer pair Pi9/2 was performed with the following procedure: denaturation at 94 °C for 5 min; followed by 34 cycles of denaturation at 98 °C for 30 s, annealing at 62 °C for 30 s, and extension at 72 °C for 30 s; final extension at 72 °C for 7 min. Sequences were compared using ClustalW2 (https://www.ebi.ac.uk/Tools/msa/clustalw2/) software and further modified using GeneDoc (https://genedoc.software.informer.com/). PAGE gel electrophoresis (8%) was used to differentiate the PCR amplification patterns of Pi9, Pi2 and non-Pi9/Pi2 alleles with the DNA ladder, DL500 (Takara, 3590A).
Breeding of Pi2 and Pi9 near-isogenic lines by MAS
The F1 plants with the blast resistance genes Pi9 or Pi2 were obtained by crossing Hui 316 with 75-1-127 or C101A51, respectively. The F1 plants were backcrossed with Hui 316 for five generations to BC5F1. At each generation, a pollen mixture collected from the selected plants with the Pi9 or Pi2 allele determined by MAS was used to pollinate Hui 316. The seeds resulting from the selfing were harvested at every generation in case of hybrid loss. Furthermore, the BC5F2 plants that were homozygous at the resistance loci were selected and their selfed seeds were retained. The homozygous introgression lines from BC5F2 were further selected based on DNA fingerprinting markers and agronomic traits compared with Hui 316 throughout the entire growth period.
Evaluation of agronomic traits
Eighty-four individuals of Hui 316, Hui 316-Pi9, Hui 316-Pi2, and their hybrids with Huifeng A and Guang 8A were divided into three plots and planted in the field in Fuzhou, Fujian Province and Tengqiao, Sanya, Hainan, Province during the summer 2016 season and the winter 2017 season, respectively. Each plot comprised four rows with seven plants per row, and the planting density was 21 cm × 21 cm. Five individual plants in the middle of the second row in each plot were collected to measure the following agronomic traits related to yield: plant height, panicles per plant, panicle length, spikelet fertility (%), 1000-grain weight, yield per plant and rice quality traits.
Statistical analysis
One-way analysis of variance (ANOVA) was performed to detect statistical differences using SPSS (Statistical Package for Social Sciences) (SPSS Inc. Released 2009; PASW Statistics for Windows, Version 18.0. Chicago: SPSS Inc.). A P-value of less than 0.05 was considered to be statistically significant.
Results and discussion
Polymorphism analysis of the marker Pi9/2 targeting Pi9, Pi2 and non-Pi2/Pi9 alleles
The restorer line, Hui 316, derived from the hybrid of Shuhui 881 × Lianhui 9-9 backcrossed with Shuhui 881, lacks broad-spectrum resistance against rice blast fungi, which was also verified in the following inoculation tests of this study. This disadvantage limits its popularization in rice production. Pi2 and Pi9 have been reported to confer broad-spectrum resistance against various rice blast fungal isolates [Citation25, Citation26]. Therefore, introgressing Pi9 or Pi2 into Hui 316 could be beneficial for enhancing the blast resistance of its hybrid combinations.
The molecular marker, Pi9-Pro, was developed previously for differentiating the different genotypes corresponding to the Pi9, Pi2, and non-Pi2/Pi9 alleles by PCR [Citation22]. However, when it was subjected to hybrid rice-breeding lines, like Hui 316 and Minfeng B (maintainer line), Pi9-Pro produced weak nonspecific amplification bands with the same size of Pi9 and Pi2 alleles (, left), which could result in inaccurate selection of the introgressed progeny in populations. To improve the specificity of the marker for MAS, a new pair of primers, Pi9/2-F (5′-TTTGTTACTAGAATCGCTCCAT-3′) and Pi9/2-R (5′-GATTAGTGAGATCCATTGTTCC-3′), was designed targeting the same polymorphic region of Pi9-Pro (). Fragments of 164 bp and 147 bp were amplified for Pi9 (75-1-127 as donor) and Pi2 (C101A51 as donor) alleles, respectively, and a longer fragment of 174 bp was detected in Hui 316 and Minfeng B (non-Pi2/Pi9 alleles) (, right). Pi9/2 showed higher specificity compared with Pi9-Pro. Therefore, the Pi9/2 marker could be ideal for MAS during the introgression process.
Figure 1. Development of the optimized InDel marker Pi9/2. (A) Pi9/2 primers are designed for targeting the genomic variation of the Pi9 (75-1-127), Pi2 (C101A51) and non-Pi9/Pi2 (Hui 316) alleles in different cultivars. (B) PCR amplification patterns of the InDel marker Pi9/2 differentiating the Pi9, Pi2 and non-Pi9/Pi2 alleles. M: DNA ladder.
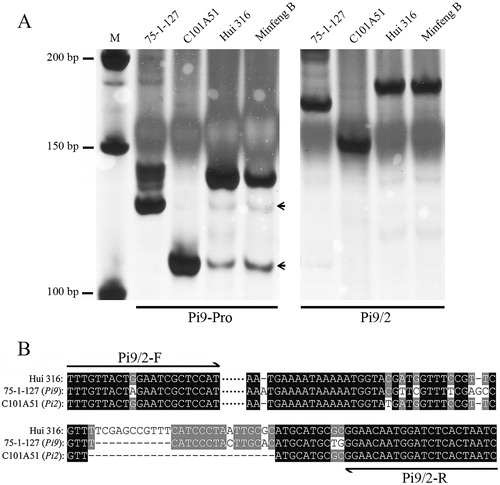
Introgressing Pi9 and Pi2 individually into Hui 316
To introduce Pi9 and Pi2 genes into Hui 316, we conducted conventional introgression breeding by backcrossing. As shown in , we crossed Hui 316 (female parent) with 75-1-127 or C101A51 (male parents) to produce the hybrid F1 lines, which were then backcrossed with Hui 316 to obtain the BC1F1 generation. Foreground selection among the BC1F1 progeny was carried out by phenotype selection and MAS. Finally, a total of 15 individuals were obtained with the Pi9 allele and 17 plants with the Pi2 allele were identified. The same strategy of selection and backcrossing was used until the BC5F1 generation was obtained (). Selecting the backcrossing progeny with similar agronomic traits to the recurrent parent could increase the recovery rate of the desired genetic background. As all, the receptor parent, Hui 316, and the donor parents, 75-1-127 and C101A51, in this study are indica cultivars, it is relatively easier for the comprehensive traits of introgressed lines to be recovered to Hui 316. The BC5F1 plants were then self-pollinated to obtain the BC5F2 lines. Twelve representative plants with a homozygous Pi9 or Pi2 allele from the BC5F2 lines were selected by MAS with the Pi9/2 marker and phenotype ( and (A)). Among them, two individuals carrying a homozygous Pi9 or Pi2 allele (named Hui 316-Pi9 or Hui316-Pi2) were verified as the alternative varieties of Hui 316 by 48 standardized fingerprinting markers covering the whole genome [Citation27] (Supplemental Figure S1) and subjected to the following analysis of agronomic performance. As shown in and , both Hui 316-Pi9 and Hui 316-Pi2 displayed high similarity with Hui 316 in the measured agronomic traits, except that Hui 316-Pi2 showed slightly higher 1,000-grain weight than Hui 316. Overall, Pi9 and Pi2 were successfully introgressed into Hui 316 through backcrossing, and the introduction of these R genes did not markedly change the agronomic traits of Hui 316.
Figure 2. Schematic diagram of introgressing Pi9 or Pi2 into Hui 316 with MAS and phenotype selection (PS).
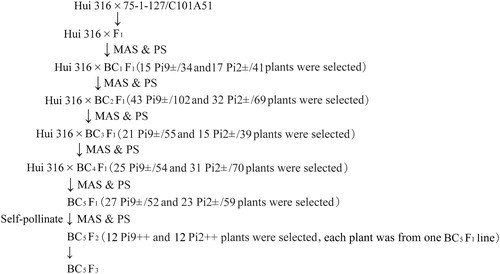
Figure 3. Identification of the homozygous Pi9 or Pi2-introgressed Hui 316 lines by MAS and characterization of Hui 316-Pi9 and Hui 316-Pi2. (A) PCR amplification patterns of marker Pi9/2 identifying 20 homozygous Pi9 or Pi2-introgressed Hui 316 lines. (B) Comparison of the plant and seed morphology of Hui 316, Hui 316-Pi9 and Hui 316-Pi2. Hui 316-Pi9 and Hui 316-Pi2 displayed similar plant and seed traits with Hui 316. Bars, 5 mm; M: DNA ladder.
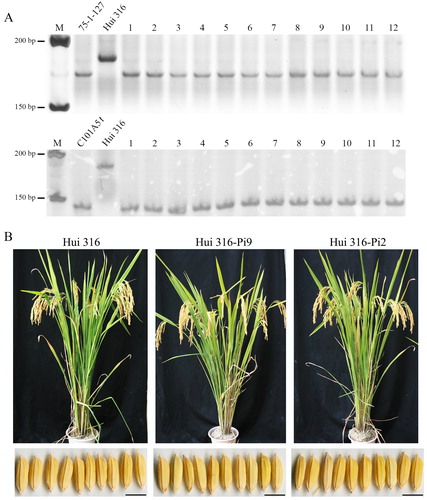
Table 1. Agronomic traits of Hui 316, Hui 316-Pi9 and Hui 316-Pi2.
Introgression of Pi9 or Pi2 enhanced blast resistance of Hui 316
To determine whether the introgression of Pi9 or Pi2 enhances blast resistance of Hui 316, the disease incidence was investigated by inoculation with blast fungi under both greenhouse and field conditions. Seedlings were inoculated with 21 blast isolates collected in Fujian Province and a mixture of their spores by spraying in a greenhouse. The results showed that both the R gene donor lines, 75-1-127 and C101A51, displayed resistance to all the blast isolates, whereas Hui 316 showed susceptibility to the isolates SM17211, SH17004, SM17192, NH15039, SM17212, SM17207 and SM17191, moderate susceptibility to the isolates JL15140, NH17026 and SM17203 and resistance to the remaining 11 isolates and their combinations (). Hui 316-Pi9 and Hui 316-Pi2 were resistant to all of the blast isolates and their combinations, which is similar to their donor parents, 75-1-127 and C101A51, respectively (). Based on these results, we concluded that the introgression of Pi9 or Pi2 can enhance the resistance of Hui 316 to the virulent blast isolates under greenhouse conditions.
Table 2. Blast disease incidence of 75-1-127, C101A51, Hui 316, Hui 316-Pi9 and Hui 316-Pi2 seedlings inoculated with 21 Fujian blast fungal isolates and their mixture in greenhouse.
In addition, we further tested the resistance of Hui 316-Pi9 and Hui 316-Pi2 under field conditions. As shown in , the integrated investigation of seedling, leaf, and neck blast disease incidence indicated that 75-1-127 displayed resistance and moderate resistance in 2016 and 2017, respectively. C101A51 showed moderate resistance in both years. Hui 316 was susceptible and highly susceptible in the tests in 2016 and 2017, respectively. However, Hui 316-Pi9 showed moderate resistance in both years, and Hui 316-Pi2 was moderately resistant and resistant in 2016 and 2017, respectively. These results indicated that Hui 316-Pi9 and Hui 316-Pi2 displayed similar blast resistance levels in field to their donor parents, 75-1-127 and C101A51, respectively. Overall, introgressing Pi9 or Pi2 into Hui 316 could markedly enhance its resistance against blast in both greenhouse and field conditions.
Table 3. Blast disease incidence of 75-1-127, C101A51, Hui 316, Hui 316-Pi9 and Hui 316-Pi2 grown in the field of Shanghang, Fujian during 2016 and 2017.
Analysis of agronomic traits of hybrid progeny derived from Hui 316-Pi9 and Hui 316-Pi2
To determine whether introgression of Pi9 or Pi2 into Hui 316 affects the agronomic characteristics of its hybrid progeny, we crossed the male sterility lines, Huifeng A and Guang 8A, with Hui 316, Hui 316-Pi9 and Hui 316-Pi2. The agronomic traits related to yield and rice quality of the hybrid plants, including plant height, number of panicles per plant, panicle length, seed setting rate, 1000-grain weight, yield per plant, brown rice rate, milled rice rate, head rice rate, transparence and grain length/breadth ratio, were investigated. As shown in , the hybrid progeny derived from Guang 8A × Hui 316-Pi9 and Guang 8A × Hui 316-Pi2 displayed similar yield traits and rice quality with those from Guang 8A × Hui 316. The hybrid plants from Huifeng A × Hui 316-Pi2 also showed similar agronomic traits and rice quality as the Huifeng A × Hui 316 progeny. However, compared with the progeny from Huifeng A × Hui 316, the hybrids from Huifeng A × Hui 316-Pi9 displayed significantly longer panicle length but lower seed setting rate (), whereas their yield per plant was not significantly different from that of the progeny from Huifeng A × Hui 316. Therefore, introgression of Pi2 into Hui 316 did not affect the traits related to the yield of its hybrids. Although introducing Pi9 into Hui 316 could change the panicle length and seed setting rate of its hybrids, these differences did not significantly influence their yield per plant and rice quality.
Table 4. Agronomic traits of the hybrid progenies derived from Hui 316, Hui 316-Pi9 and Hui 316-Pi2 crossing with various male sterility lines.
It has been reported that planting diversified rice cultivars with different R genes in the same area could enhance their integral resistance to blast fungi [Citation28, Citation29]. In addition, the development of multiline varieties consisting of blast resistant isogenic lines can also provide enhanced resistance [Citation30]. This is likely because multiple R genes in a rice population increase the scale of interaction with avirulence genes, which limits the coevolution of pathogenic blast fungi [Citation31]. Gallet et al. has also characterized this synergistic blast resistance in various rice cultivars and blast isolates [Citation32]. Therefore, as the agronomic traits of Hui 316-Pi9 and Hui 316-Pi2 are similar, the planting of their hybrids derived from the same male sterility line together may provide long-lasting blast resistance in the field.
Conclusions
In this study, we designed an optimized PCR marker, Pi9/2, which targets the same polymorphic region of Pi9-Pro but displays higher specificity in identifying the non-Pi2/Pi9 allele in the newly bred restorer line, Hui 316. With the assistance of Pi9/2, we introduced Pi9 and Pi2, the two broad-spectrum blast resistance genes, individually, into the newly bred restorer line, Hui 316. Our investigation indicated that introgression of Pi9 or Pi2 into Hui 316 could markedly improve its resistance to blast under both greenhouse and field conditions with little influence on its restoring ability. Thus, Pi9/2 is an efficient marker for introducing Pi9 or Pi2 to improve the blast resistance of rice cultivars by MAS.
Supplemental Material
Download PDF (131 KB)Acknowledgements
We are grateful to Dr. Guo-Liang Wang from Ohio State University for kindly providing the rice lines C101A51 and 75-1-127.
Disclosure statement
No potential conflict of interest was reported by the authors.
Funding
This work was supported by the Projection of Public Welfare of Fujian Province, China under Grant (2017R1019-10) and Natural Science Foundation of Fujian Province, China under Grant (2014J01102).
References
- Pennisi E. Armed and dangerous. Science. 2010;327:804–805.
- Skamnioti P, Gurr SJ. Against the grain: safeguarding rice from rice blast disease. Trends Biotechnol. 2009;27:141–150.
- Dodds PN, Rathjen JP. Plant immunity: towards an integrated view of plant-pathogen interactions. Nat Rev Genet. 2010;11:539–548.
- Chen H, Wang S, Xing Y, et al. Comparative analyses of genomic locations and race specificities of loci for quantitative resistance to Pyricularia grisea in rice and barley. Proc Natl Acad Sci USA. 2003;100:2544–2549.
- Chen HQ, Chen ZX, Shen NI, et al. Pyramiding three genes with resistance to blast by marker-assisted selection to improve rice blast resistance of Jin 23B. Chin J Rice Sci. 2008;22:23–27.
- Gouda PK, Saikumar S, Varma CMK, et al. Marker-assisted breeding of Pi-1 and Piz-5 genes imparting resistance to rice blast in PRR78, restorer line of Pusa RH-10 Basmati rice hybrid. Plant Breed. 2013;132:61–69.
- Hittalmani S, Parco A, Mew TV, et al. Fine mapping and DNA marker-assisted pyramiding of the three major genes for blast resistance in rice. Theor Appl Genet. 2000;100:1121–1128.
- Khan GH, Shikari AB, Vaishnavi R, et al. Marker-assisted introgression of three dominant blast resistance genes into an aromatic rice cultivar Mushk Budji. Sci Rep. 2018;8:4091.
- Singh VK, Singh A, Singh SP, et al. Incorporation of blast resistance into “PRR78”, an elite Basmati rice restorer line, through marker assisted backcross breeding. Field Crops Res. 2012;128:8–16.
- Tanweer FA, Rafii MY, Sijam K, et al. Introgression of blast resistance genes (putative Pi-b and Pi-kh) into elite rice cultivar MR219 through marker-assisted selection. Front Plant Sci. 2015;6:1002.
- Wang Z, Jia Y, Rutger JN, et al. Rapid survey for presence of a blast resistance gene Pi‐ta in rice cultivars using the dominant DNA markers derived from portions of the Pi‐ta gene. Plant Breed. 2007;126:36–42.
- Ashkani S, Rafii MY, Shabanimofrad M, et al. Molecular breeding strategy and challenges towards improvement of blast disease resistance in rice crop. Front Plant Sci. 2015;6:886.
- Wang BH, Ebbole DJ, Wang ZH. The arms race between Magnaporthe oryzae and rice: diversity and interaction of Avr and R genes. J Integr Agric. 2017;16:2746–2760.
- Chen X, Shang J, Chen D, et al. A B-lectin receptor kinase gene conferring rice blast resistance. Plant J. 2006;46:794–804.
- Fukuoka S, Saka N, Koga H, et al. Loss of function of a proline-containing protein confers durable disease resistance in rice. Science. 2009;325:998–1001.
- Chen DH, Zeigler RS, Ahn SW, et al. Phenotypic characterization of the rice blast resistance gene Pi-2(t). Plant Dis. 1996;80:52–56.
- Mackill D,MB. Inheritance of blast resistance in near-isogenic lines of rice. Phytopathology. 1992;82:746–749.
- Liu G, Lu G, Zeng L, et al. Two broad-spectrum blast resistance genes, Pi9(t) and Pi2(t), are physically linked on rice chromosome 6. Mol Genet Genom. 2002;267:472–480.
- Ellur RK, Apurva K, Ashutosh Y, et al. Improvement of Basmati rice varieties for resistance to blast and bacterial blight diseases using marker assisted backcross breeding. Plant Sci. 2016;242:330–341.
- Luo Y, Yin Z. Marker-assisted breeding of Thai fragrance rice for semi-dwarf phenotype, submergence tolerance and disease resistance to rice blast and bacterial blight. Mol Breed. 2013;32:709–721.
- Balakrishnan D, Robin S, Rabindran R, et al. Marker assisted backcross breeding approach to improve blast resistance in Indian rice (Oryza sativa) variety ADT43. Euphytica. 2014;200:61–77.
- Tian D, Chen Z, Chen Z, et al. Allele-specific marker-based assessment revealed that the rice blast resistance genes Pi2 and Pi9 have not been widely deployed in Chinese indica rice cultivars. Rice. 2016;9:19.
- Cheng S. Current status and prospect in the development of breeding materials and breeding methodology of hybrid rice. Chin J Riceence. 2000;14:165–169.
- Campbell MA, Chen D, Ronald PC. Development of co-dominant amplified polymorphic sequence markers in rice that flank the Magnaporthe grisea resistance gene Pi7(t) in recombinant inbred line 29. Phytopathology. 2004;94:302–307.
- Qu S, Liu G, Zhou B, et al. The broad-spectrum blast resistance gene Pi9 encodes a nucleotide-binding site-leucine-rich repeat protein and is a member of a multigene family in rice. Genetics. 2006;172:1901–1914.
- Zhou B, Qu S, Liu G, et al. The eight amino-acid differences within three leucine-rich repeats between Pi2 and Piz-t resistance proteins determine the resistance specificity to Magnaporthe grisea. Mol Plant Microbe Interact. 2006;19:1216–1228.
- X-Z L, Cong X-h, Liu H-Z, et al. Establishment of DNA fingerprinting database for parental lines of hybrid rice. J Nucl Agric Sci. 2012;26:853–861.
- Shinzo A. Breeding of a blast resistant multiline variety of rice, Sasanishiki BL. Jpn Agric Res Q. 2004;38:149–154.
- Zhu Y, Chen H, Fan J, et al. Genetic diversity and disease control in rice. Nature. 2000;406:718–722.
- Ishizaki K, Hoshi T, Abe S, et al. Breeding of blast resistant isogenic lines in rice variety “Koshihikari” and evaluation of their characters. Breed Sci. 2005;55:371–377.
- Tack AJ, Thrall PH, Barrett LG, et al. Variation in infectivity and aggressiveness in space and time in wild host-pathogen systems – causes and consequences. J Evol Biol. 2012;25:1918–1936.
- Gallet R, Bonnot F, Milazzo J, et al. The variety mixture strategy assessed in a G × G experiment with rice and the blast fungus Magnaporthe oryzae. Front Genet. 2013;4:312.