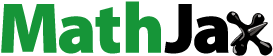
Abstract
Graptopetalum paraguayense E. Walther (GP) belongs to the Crassulaceae family and has several health benefits. In the present study we evaluated the cytotoxic, anti-Herpes Simplex virus (HSV) and antibacterial activities of a total methanol/water GP extract. The results from the cytotoxicity investigation of the tested extract showed a high cell tolerable concentration range. The GP extract demonstrated a significant inhibitory effect on the wild-type HSV-1 strain Victoria in a concentration of 0.0001 mg/mL equal to the maximal nontoxic concentration (with 97.5% protection of the cells). Regarding the acyclovir-resistant mutant HSV-2 strain PU and wild-type HSV-2 strain Bja, the antiviral activity and selectivity of the extract were very low, with EC50 values from 0.1 to 0.01 mg/mL, and the selectivity index ranging from 25 to 250, respectively. The GP extract exhibited selective inhibitory activity also on the growth of Gram-positive bacterial pathogens, with Staphylococcus aureus being most sensitive. It also prevented the formation of MRSA biofilm up to 50% at a concentration of 1.6 mg/mL and up to 90% at a concentration of 2.5 mg/mL. The anti-herpetic and antibacterial activities demonstrated in our investigation are probably related to the presence of phenols, and in particular gallic acid. The antimicrobial activity of the GP extract holds a promise for the future development of nutrition supplements based on G. paraguayense and therefore warrants further studies aimed at isolating biologically active compounds or mixtures thereof with subsequent in-depth investigation of their anti-HSV and antibacterial mechanisms of action.
Introduction
Viral and bacterial diseases are considered to be one of the major challenges in medicine due to their global spread, difficult epidemiological control, increased chemotherapeutical resistance and high mortality rate [Citation1]. Among the most common infections are those caused by human herpes viruses, including Herpes Simplex virus type 1 and type 2 (HSV-1 and HSV-2) spread worldwide [Citation2]. HSV infections range from inapparent [Citation3], through orofacial and genital lesions [Citation4] to severe life-threatening encephalitis [Citation5] and also are the probable cause of cervical cancer [Citation6]. Genital herpes increases the risk of acquisition and transmission of the human immunodeficiency virus [Citation7]. Persistent infections, including HSV, could suppress the host immune system and enhance the possibility of co-infection with some pathogenic bacteria, such as causative agents of food-borne, skin, urinary or respiratory tract diseases [Citation8]. The standard treatment of HSV infections is acyclovir (ACV, 2-amino-1,9-dihydro-9-((2-hydroxyethoxy)methyl)-6H-purin-6-one) and its nucleoside analogues [Citation4]. Systemic application of these agents is often limited by the development of drug-resistance or toxicity [Citation9], especially in immunosuppressed patients [Citation7, Citation10] which highlights the need for new therapeutic options. The same trend for accelerated development of antimicrobial resistance is observed among pathogenic bacteria where the widespread use of antibiotics has led to the emergence of resistant strains [Citation11, Citation12]. In this context, the development of alternative antibacterial and antiviral agents with significant effect, low toxicity and novel mechanisms of action is urgently required.
Medicinal plants are a source of chemical compounds with different pharmacological properties [Citation13]. Graptopetalum paraguayense E. Walther (GP) belongs to the Crassulaceae family and is a succulent plant species distributed in tropical and subtropical countries and grown mainly as an ornamental house plant in temperate regions. It was found that GP aqueous extracts show anti-inflammatory [Citation14], antioxidant [Citation15] and antineoplastic activities [Citation16]. Preliminary phytochemical studies on the effect of n-butanol extract from fresh watery juice of GP leaves demonstrate anti-conjunctivitis activity due to probable synergistic effect between alkylamines, hydroxycarboxylic, aliphatic and aromatic carboxylic acids [Citation17]. Published data [Citation18] suggest that the GP polyphenolic fraction inhibits hepatitis B virus (HBV) replication, gluconeogenesis and lipogenesis and could be used for treatment of chronic HBV patients. In vivo studies on the toxicological safety of aqueous GP extract prove that it does not possess mutagenicity on mammalian erythrocyte micronuclei, acute lethal effect at max dose of 5 g/kg body weight (bw) in rats or adverse effects up to a dose of 1 g/kg (bw) [Citation19].
Although many members of the Crassulaceae family exhibit anti-herpetic [Citation20, Citation21] and antibacterial effects against Staphylococcus aureus, Klebsiella pneumoniae, Salmonella typhi and Escherichia coli [Citation22], there is little information in the literature on the anti-HSV and antimicrobial activities of G. paraguayense E. Walther. Therefore, the aim of our study was to evaluate the effectiveness and selectivity of a total GP extract on HSV-1/HSV-2 and a panel of Gram-positive and Gram-negative bacterial pathogens.
Materials and methods
Plant material and GP extract preparation
G. paraguayense E. Walter was grown as an ornamental plant at the Institute of Organic Chemistry with Centre of Phytochemistry, Bulgaria. Botanical identification and authentication were performed by Asen Asenov PhD (Sofia University ‘St. Kliment Ohridski’) and Antoaneta Petrova PhD (Botanical Garden, Bulgarian Academy of Sciences), and a voucher specimen number SO 107 621 was deposited in the herbarium of Sofia University, Bulgaria. Leaf extract was prepared as follows: the leaves were cleaned, washed with distilled water, air-dried overnight, and then freeze-dried at –50 °C by a vacuum freeze-dryer (ALPHA 1-2 LD plus, Martin Christ Gefriertrocknungsanlagen GmbH). Ten-gram portions of the plant were mixed with 200 mL methanol/water (75:25,v/v) followed by heating at 70 °C for 1 h in a laboratory thermo mixer (Analytik Jena AG) and then cooling to room temperature. The solution was filtered and dried by a vacuum freeze-dryer. The extract was stored at –70 °C until use. Stock solutions for the experiments were prepared at initial concentration of 50 mg/mL in dimethyl sulfoxide (DMSO, Sigma, Germany) and distilled water, respectively. Before application, the stock solutions were diluted in DMEM (Dulbecco’s Modified Eagle Medium, Sigma, Germany) at different concentrations.
In vitro analyzes
Cell cultures and HSV propagation
Vero (Green monkey kidney) and RD (human rhabdomyosarcoma) cell lines (National Center of Infectious and Parasitic Diseases, Bulgaria) were cultured in DMEM supplemented with 10% heat-inactivated fetal bovine serum (FBS, Gibco, USA), while 2% FBS medium was employed for the antiviral experiments. The following HSV strains (collection) were used: two wild-type (wt) strains sensitive to ACV, Victoria (HSV-1) and Bja (HSV-2), and two clinical isolates resistant to ACV (ACVR), DD (HSV-1) and PU (HSV-2). Virus titers were determined by the Reed and Muench method [Citation23] and were expressed as 50% tissue culture infective dose/mL (TCID50/mL). The anti-herpes drug ACV (Sigma, USA) (1 mg/mL) was used as a standard.
Cytotoxicity assay
The cytotoxicity of the GP extract was determined by microscopic observation of monolayers [Citation24] and by a colorimetric MTT [3-(4,5-dimethylthiazol-2-yl)-2,5-diphenyltetrazolium bromide] assay [Citation25] with minor modifications [Citation26]. Vero and RD cells (3 × 105 cells/mL) were treated with different concentrations of the extract (0.00001–2.5 mg/mL) and the drug ACV (0.0005–0.1 mg/mL). The optical density (OD) was determined at λ = 540 nm by an ELISA reader (Bio-Tek Instruments, Germany). The percentage (%) of viable treated cells was calculated in relation to untreated controls (ODexp/ODcell control×100). CC50 was the concentration required to reduce the OD or to induce visible morphological changes in 50% of cells. The maximal nontoxic concentration (MNC) was defined as the highest concentration which did not cause injury or death of the treated cells.
Anti-HSV activity assay
The virus-inhibitory effect of the GP extract was determined by two assays.
Cytopathic effect reduction assay [Citation27]
Vero cells (104 cells/mL) were infected with the tested HSV strains (0.1 mL/well virus suspension) at a low multiplicity of infection MOI 100 TCID50/well. After virus adsorption, the infected cells were treated with non-cytotoxic (MNC and lower) concentrations of the GP extract. Plates were examined at 72 h by inverted light microscopy for cellular changes/cytopathic effect (CPE) on Vero cells. The reduction of virus multiplication was calculated as percentage of virus control (CPEexp/CPEvirus control×100). The half maximal effective concentration (EC50) was expressed as the concentration that achieved 50% protection of cells from the HSV-induced destruction. Selectivity index (SI) was determined as CC50/EC50.
MTT assay for screening of anti-HSV compounds [Citation28]
Confluent Vero monolayers were overlaid with virus suspensions at a low MOI 100 TCID50/well and non-cytotoxic (MNC and low) concentrations of GP extract (0.1 mL/well). The effect was measured at 72 h post infection (p.i.) as described in the cytotoxicity assay. The percentage of protection was calculated as follows: [(ODexp) – (ODvirus control)]/[(ODcell control) – (ODvirus control)] × 100 (%), where ODexp, ODvirus control and ODcell control indicate the absorbance of the test sample, the virus and the cell controls, respectively. The EC50 and SI were determined as described above.
Evaluation of antibacterial and anti-biofilm activity
Bacterial strain and growth conditions
The antibacterial activity was tested on Staphylococcus aureus ATCC (American Type Culture Collection, USA) 29213, Enterococcus faecalis ATCC 29212, Escherichia coli 25922, Pseudomonas aeruginosa ATCC 27853, methicillin resistant S. aureus (MRSA) NBIMCC 8327 (National Bank for Industrial Microorganisms, Bulgaria) and Streptococcus pyogenes SAIM 10535 (Collection of the St. Angeloff Institute of Microbiology, Bulgaria). The strains were maintained in Trypticase Soy Agar/Broth (TSA/TSB, HiMedia, India). The broth microdilution assay (BMD) was performed in Mueller Hinton broth (MHB, ThermoScientific, UK). The biofilm experiment was carried out in Brain Heart Infusion Broth (BHI, HiMedia, India) supplemented with 2% d-glucose (Sigma, Germany).
Broth microdilution test
The antibacterial activity of the tested extract was evaluated following ISO 20776/1-2006 [Citation29]. Twofold serial dilutions of the extract from 5 down to 0.3125 mg/mL were prepared in triplicate for all bacterial test-strains. The plates were incubated at 37 °C for 24 h. The lowest drug concentration which inhibited the visible bacterial growth was accepted as the minimal inhibitory concentration (MIC). Gentamycin (0.008–4 mg/L) and penicillin (0.008–4 mg/L) (Gibco, UK) were used as reference antibiotics (positive control). The recommendations of EUCAST (European Committee on Antimicrobial Susceptibility Testing) were followed for the analysis of the results [Citation30]. Phosphate buffered saline (PBS) with pH 7.4 (HiMedia, India) served as negative control and MHB, as a blank solution.
Biofilm formation assay
The biofilm formation assay was performed on MRSA according to the protocol of Stepanovic et al. [Citation31]. Briefly, twofold serial dilutions of the extract from 5 down to 0.3125 mg/mL were prepared in triplicate in 96-well polystyrene tissue culture plates. The biofilm formation was documented under an inverted light microscope (40× magnification) after staining with crystal violet. Thereafter, the attached bacteria were dissolved in 160 μL of 33% acetic acid and the OD was measured at λ = 550 nm (ELISA reader, Bio-Tek Instruments, Germany). The minimum biofilm inhibition concentration (MBIC50) was defined as the concentration of the GP extract that led to 50% inhibition of the biofilm formation. The biofilm-inhibitory effect was calculated based on the absorbance of crystal violet.
Calculation of median inhibitory concentrations and statistical analysis
Data were presented as mean values with standard deviation (±SD). Experiments were performed in triplicate, independent-sample Student’s t-test was used and p < 0.05 was considered significant. CC50 and EC50 were obtained from dose-effect curves. The correlation coefficient values (R2) were calculated. The median biofilm inhibitory concentration of the extract was calculated with the GraphPad Prism software version 8.00 for Windows, La Jolla California USA (www.graphpad.com) using the nonlinear regression model log(inhibitor) vs. normalized response, variable slope: Y = 100/(1 + 10^((LogIC50-X)*HillSlope)).
Results and discussion
Many members of the Crassulaceae family with therapeutic potential against infectious and inflammatory processes contain phenols, saponins, glycosides, quinolones, flavonoids, tanins, terpenoids, essential oils, alkaloids, polypeptides, etc. [Citation21, Citation22]. It is known that flavonoids such as quercetin and kaempferol show anti-herpetic activities [Citation32].
Little data exist for the GP chemical composition that could be associated with its bio-medical benefits. Seven flavonoid glucoside derivatives of quercetin and kaempferol were isolated from GP methanol extract by Liu et al. [Citation33]. To evaluate the anti-oxidative activities of water (GWE), 50% ethanol (GE50), and 95% ethanol (GE95) extracts of GP, the total phenol and anthocyanin contents were analyzed by Chung et al. [Citation15]. The highest total phenol and anthocyanin contents were obtained from GE50 of GP and could be attributed to the radical-scavenging activities of the extract, probably due to gallic acid [Citation34].
In the present study, we focused on the in vitro cytotoxicity, biofilm formation, antiviral and antibacterial activities of the total methanol extract obtained from GP leaves. The results of our study provide further evidence of the potential use of plants, which are a reservoir for pharmacologically active compounds.
Design of the study
We selected for our study a panel of HSV-1/HSV-2 strains sensitive to ACV and ACVR thymidine kinase (TK) mutants and pathogenic bacterial strains. For the investigation of the in vitro cytotoxicity and the antimicrobial potential of G. paraguayense methanol extract, we followed the procedure presented in .
Cytotoxic effect of total GP extract
Microscopic observation of the morphology of Vero and RD cells at 72 h after treatment with a concentration range of 0.00001–1 mg/mL did not show cytopathology typical of any toxic effect of the extract. In contrast, some changes in the morphology and density of cells, such as rounding, detachment and loss of membrane integrity, were observed after application of higher concentrations of GP extract about 2–2.5 mg/mL. Similar morphological characteristics have been demonstrated in cells exposed to ACV in the range of 0.1–1 mg/mL, in which apoptosis has been previously described [Citation35].
Cell survival analyzes by the MTT assay indicated that the GP extract caused growth inhibition and decreased cell viability in both cell lines in a dose-dependent manner (). Dynamics of viability of the cells treated with different concentrations of GP extract at 72 h were determined from the dose-response curves. The R2 values exceeded 0.95. The concentration range used to assess the cytotoxic activity of GP extract was well tolerated by both cell lines.
Figure 2. Cytotoxic activity of GP extract on the viability of Vero and RD cell lines at 72 h treatment. Data are presented as percentage (%) of cell control.
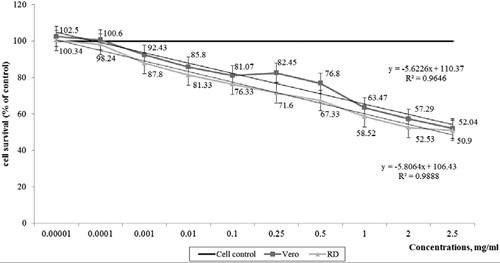
The results from the cytotoxicity assay showed that MNC values obtained by microscopic observation of cell morphology and MTT assay were different, depending on the cell line used (p < 0.05) ().
Table 1. Cytotoxic activity of GP extract and ACV on Vero and RD cell lines, expressed as MNC and CC50 obtained by different methods.
Based on the MNC data the GP extract expressed 10 times higher cytotoxicity against RD than Vero cell lines. The CC50 values obtained by the MTT assay correlated well with the observed morphological changes in the cells. The cytotoxic potential of the extract was well demonstrated by CC50 values according to which the GP extract was 6.25 times less cytotoxic in comparison with the anti-herpetic drug ACV.
Taken together our data on the in vitro cytotoxicity of the GP extract indicate that it is characterized with a high cell tolerable concentration range. The data from our experiments showed that the GP extract could be administrated in a concentration range (MNC and lower) that avoids significant cell damage. These results are in accordance with the fact that Graptopetalum paraguayense is a widely consumed vegetable in Taiwan [Citation36].
Assessment of anti-HSV-1/HSV-2 activities of total GP extract
In our study, the antivirus inhibitory effect of the total GP extract was evaluated against the reproduction of HSV-1 and HSV-2 by p.i. treatment of Vero cells and by a number of different assays. EC50 was indicative of the antiviral activity, while SI of the selectivity. All antiviral experiments were performed with the aim to overcome ACV resistance; wt strains sensitive to ACV (Victoria, HSV-1) and (Bja, HSV-2) as well as clinical ACVR strains (DD, HSV-1) and (PU, HSV-2) with different TK gene mutations were employed. The results suggested that the inhibitory effect of the GP extract was selective, strain-specific and dose-dependent. The GP extract effectively inhibited the replication of wt HSV-1 strain (Victoria) in a concentration 0.0001 mg/mL equal to MNC (with 97.5% protection of the cells) ( and ). In the present study, ACV, which we used as a positive control in a concentration range 0.0005–0.05 mg/mL, suppressed the virus replication of wt HSV-1 strain Victoria (with total protection 100%), whereas wt HSV-2 strain Bja was 10 times less sensitive.
Table 2. Antiviral activity of leaf extract of G. paraguayense against wt HSV strains sensitive to ACV (Victoria, HSV-1) and (Bja, HSV-2).
Table 3. Antiviral activity of leaf extract of G. paraguayense against HSV-1/HSV-2 ACVR mutants (DD, HSV-1) and (PU, HSV-2).
ACV demonstrated 10 times lower selectivity for the Bja strain (HSV-2) than for the other wt HSV-1 strain. The tested GP extract was 20 times more effective and selective as an inhibitor of the growth of the ACVR mutant DD (HSV-1) compared to ACV and gave cell protection of 65.5%. On the other hand, the antiviral activity and selectivity of the extract against ACVR HSV-2 mutant (PU) and wt HSV-2 strain Bja were very low with EC50 values from 0.1 to 0.01 mg/mL and SI ranging from 25 to 250, respectively. In comparison, ACV was also not effective and selective as an inhibitor of the replication of TK mutants. The resistance of HSV mutants to ACV was confirmed by EC50 equal to MNC, and the SI values were very low.
Briefly, according to EC50, the virus-specific response ranked as follows:
The selectivity, expressed as SI ( and ), was also virus-specific. The virus-specific response ranked in the following order:
Thus, the HSV-inhibitory effect of GP extract seems to be selective and specific. At this stage of our investigations it is not possible to discuss in details the mechanism of the inhibitory effect of the total methanol extract of G paraguayense. The HSV-1 inhibitory effect against the ACV sensitive strain Victoria could not be explained by the direct virucidal activity alone (data not shown). According to this study, our results indicated significant anti-herpetic effect at the stage of the replication cycle of HSV-1 after adsorption and entry into the host-cell. The selectivity of the antiviral action to GP extract was confirmed by the variation in sensitivity of different HSV-1 and HSV-2 strains sensitive or resistant to ACV. The different results for the inhibitory effects against HSV strains may be due to some differences in replication, regulatory proteins (ICP group) and presence of mutations in the genes coding for some viral enzymes (especially thymidine kinase (TK)). The antiviral efficacy of ACV is related to the specific inhibition of the viral DNA polymerase during the replication cycle when new viral DNA is synthesized [Citation37]. Compared to ACV (with total protection of the cells 100%), the anti-HSV-1 effect of the GP extract was less pronounced (97.5% cell protection) but due to its different nature, viral mechanism of action and low cytotoxicity, it has the potential to be used as an additive in the treatment of HSV-1 infections after detailed evaluation of its pharmacological interactions with clinically applied anti-herpetic drugs.
Antibacterial activity of total GP extract
The leaf extract obtained from G. paraguayense was tested on six pathogenic bacterial strains, including four Gram-positive and two Gram-negative bacterial species. The parameters for determination of antibacterial and anti-biofilm activity (growth inhibition and mass of the biofilm) investigated in the present study describe the effect of GP extract on a population level. This lays the groundwork for future pharmacological studies of the exact mechanism of action. The results from the BMD test are presented in .
Table 4. Antibacterial activity of total extract obtained from G. paraguayense.
The MIC values of the tested extract ranged between 2.5 and 5 mg/mL for the Gram-positive strains, wherein the Staphylococcus and Streptococcus strains were more sensitive than E. faecalis. The Gram-negative species were not susceptible to exposure to the extract. The respective values of the referent antibiotics penicillin and gentamicin are given for comparison. According to our results, the GP extract showed moderate antibacterial activity on Gram-positive bacterial strains at MICs of 2.5 and 5 mg/mL depending on the strain. The pathogenic bacterial species S. aureus was more susceptible to the extract than E. faecalis and S. pyogenes. This result corresponds to the published data on the anti-staphylococcal effect of methanol extracts obtained from other plants belonging to the Crassulaceae family, such as Bryophyllum pinnatum (BP) Kurz [Citation22]. The GP extract tested in our study showed a three-fold lower MIC (2.5 mg/mL) on S. aureus than the BP methanol extract (6.25 mg/mL), suggesting that methanol is a better solvent for extraction of constituents with antibacterial activity than water. In addition, the GP extract exerted a four- to six-fold stronger inhibitory potential on S. aureus than the BP ethanol extract (MIC = 10 mg/mL) obtained from B. pinnatum Kurz [Citation38]. Considering the estimated maximal dose without adverse effects (1 mg/kg bw) in rats for aqueous GP extracts [Citation19], our result (MIC = 2.5 mg/mL) suggests that systematic application of the GP extract for treatment of Gram-positive bacterial infections could be problematic. Therefore, the GP extract could be developed as a topical antibacterial natural product. To explore this possibility further, we determined the anti-biofilm potential of the GP extract, considering the fact that biofilm formation is an important mechanism of bacteria to overcome treatment with chemotherapeutics and survive in adverse environmental conditions [Citation39].
Anti-biofilm activity of GP extract
The anti-biofilm effect of the extract was evaluated on MRSA. After 24 h of exposure, the extract inhibited the formation of MRSA biofilm by 90% at a concentration of 2.5 mg/mL (). The biofilm inhibitory effect increased in a concentration-dependent manner and was strongest at the highest concentration applied in this study. The MBIC50 was 1.6 mg/mL, which is lower than the MIC value determined for this strain.
Figure 3. Biofilm formation of MRSA after exposure to leaf extract obtained from Graptopetalum paraguayense. Qualitative microscopic (40× microscopic magnification) and quantitative spectrophotometric (MBIC50) evaluation. Scale bar = 50 μm; 40× magnification.
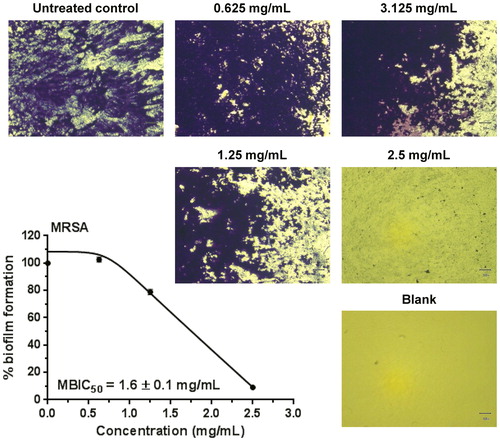
According to our data, GP inhibited by 50% the formation of MRSA biofilm at a concentration three-fold lower (1.6 mg/mL) than the estimated lethal dose in rats (5 g/kg bw), whereas the MIC estimated in liquid culture (2.5 mg/mL) was only two-fold lower. However, the median effective anti-biofilm concentration was still higher than the maximal dose without adverse effects (1 g/kg bw) which directs to the necessity for fractionation of the GP extract in order to search for chemical constituents with stronger anti-biofilm activity. Concerning the possible mechanism of action of the GP extract, based on our data we could hypothesize that most probably it does not target the same cellular structures as β-lactam antibiotics [Citation40] because there was no difference in the activity of the GP extract against the methicillin sensitive and methicillin resistant S. aureus tested in the present study. The in vitro antibacterial and anti-biofilm activities found in our experiments as well as the proven antioxidant effect [Citation15] of G. paraguayense may be attributed to the presence of secondary metabolites belonging to various chemical classes present in the GP extract [Citation33].
Conclusions
This pilot investigation brings attention to the biological activity of Graptopetalum paraguayense E. Walter and presents for the first time its anti-Herpes Simplex virus, antibacterial and MRSA anti-biofilm effect. The total GP methanol extract demonstrated a significant inhibitory effect on HSV-1, moderate activity against Gram-positive bacterial strains and holds a promise for future development of nutritional supplements. However, further in-depth studies on the GP extract and its chemical composition are needed in order to identify the biologically active compounds which are responsible for the observed antiviral, anti-bacterial and/or anti-biofilm activities as well as to clarify the possible mechanism of their action.
Disclosure statement
No potential conflict of interest was reported by the authors.
Additional information
Funding
References
- Fauci AS. Infectious diseases: considerations for the 21st century. Clin Infect Dis. 2001;32:675–685.
- Wilson SS, Fakioglu E, Herold BC. Novel approaches in fighting herpes simplex virus infections. Expert Rev Anti Infect Ther. 2009;7:559–568.
- Whitley RJ, Kimberlin DW, Roizman B. Herpes simplex viruses. Clin Infect Dis. 1998;26:541–555.
- De Clercq E, Field HJ. Antiviral prodrugs - the development of successful prodrug strategies for antiviral chemotherapy. Br J Pharm. 2006;147:1–11.
- Kennedy PGE, Chaudhuri A. Herpes simplex encephalitis. J Neurol Neurosurg Psychiatry. 2002;73:237–238.
- Lehtinen M, Koskela P, Jellum E, et al. Herpes simplex virus and risk of cervical cancer: a longitudinal, nested case-control study in the Nordic countries. Am J Epidemiol. 2002;156:687–692.
- Celum CL. The interaction between herpes simplex virus and human immunodeficiency virus. Herpes: J IHMF. 2004;11:36a–45a.
- Almand EA, Moore MD, Jaykus L-A. Virus-bacteria interactions: an emerging topic in human infection. Viruses 2017;9:58.
- Piret J, Boivin G. Resistance of herpes simplex viruses to nucleoside analogues: mechanisms, prevalence, and management. Antimicrob Agents Chemother. 2011;55:459–472.
- Frobert E, Cortay JC, Ooka T, et al. Genotypic detection of acyclovir-resistant HSV-1: characterization of 67 ACV-sensitive and 14 ACV-resistant viruses. Antiviral Res. 2008;79:28–36.
- WHO. Antimicrobial resistance: global report on surveillance. France: World Health Organization; 2014.
- European Centre for Disease Prevention and Control. Surveillance of antimicrobial resistance in Europe – Annual Report of the European Antimicrobial Resistance Surveillance Network (EARS-Net) 2017 Antimicrobial resistance surveillance in Europe. Stockholm: ECDC; 2018.
- Raskin I, Ribnicky DM, Komarnytsky S, et al. Plants and human health in the twenty-first century. Trends Biotechnol. 2002;20:522–531. doi:10.1016/S0167-7799(02)02080-2.
- Chen S-J, Yen C-H, Liu J-T, et al. Anti-inflammatory effect of water extracts of Graptopetalum paraguayense supplementation in subjects with metabolic syndrome: a preliminary study. J Sci Food Agric. 2016;96:1772–1776.
- Chung Y-C, Chen S-J, Hsu C-K, et al. Studies on the antioxidative activity of Graptopetalum paraguayense E. Walther. Food Chem. 2005;91:419–424.
- Hsu WH, Chang CC, Huang KW, et al. Evaluation of the medicinal herb Graptopetalum paraguayense as a treatment for liver cancer. PLoS ONE. 2015;10:e0121298.
- Markova NV, Batovska DI, Kozuharova EK, et al. Anti-conjunctivitis effect of fresh juice of xGraptoveria (Crassulaceae): a phytochemical and ethnobotanical study. J Intercult Ethnopharmacol. 2015;4:24–28.
- Jhuang HJ, Hsu WH, Lin KT, et al. Gluconeogenesis, lipogenesis, and HBV replication are commonly regulated by PGC-1alpha-dependent pathway. Oncotarget 2015;6:7788–7803.
- Chung Y-C, Chou S-T, Jhan J-K, et al. In vitro and in vivo safety of aqueous extracts of Graptopetalum paraguayense E. Walther. J Ethnopharmacol. 2012;140:91–97.
- Orhan I, Deliorman-Orhan D, Özçelik B. Antiviral activity and cytotoxicity of the lipophilic extracts of various edible plants and their fatty acids. Food Chem. 2009;115:701–705. doi:10.1016/j.foodchem.2009.01.024.
- Ürményi FGG, Saraiva GN, Casanova LM, et al. Anti-HSV-1 and HSV-2 flavonoids and a new kaempferol triglycoside from the medicinal plant Kalanchoe daigremontiana. Chem Biodivers. 2016;13:1707–1714.
- Nwadinigwe AO. Antimicrobial activities of methanol and aqueous extracts of the stem of Bryophyllum pinnatum Kurz (Crassulaceae.). Afr J Biotechnol. 2011;10:16342–16346.
- Reed LJ, Muench H. A simple method of estimating fifty per cent endpoints. Am J Epidemiol. 1938;27:493–497.
- Serkedjieva J, Hay AJ. In vitro anti-influenza virus activity of a plant preparation from Geranium sanguineum L. Antivir Res. 1998;37:121–130.
- Mosmann TR. Rapid colorimetric assay for cellular growth and survival: application to proliferation and cytotoxicity assays. J Immunol Methods. 1983;65:55–63.
- Sieuwerts AM, Klijn JG, Peters HA, et al. The MTT tetrazolium salt assay scrutinized: how to use this assay reliably to measure metabolie activity of cell cultures in vitro for the assessment of growth characteristics, IC50-values and cell survival. Eur J Clin Chem Clin Biochem. 1995;33:813–824.
- El-Toumy SA, Salib JY, El-Kashak WA, et al. Antiviral effect of polyphenol rich plant extracts on herpes simplex virus type 1. Food Sci Hum Wellness. 2018;7:91–101. doi:10.1016/j.fshw.2018.01.001.
- Takeuchi H, Baba M, Shigeta S. An application of tetrazolium (MTT) colorimetric assay for the screening of anti-herpes simplex virus compounds. J Virol Methods. 1991;33:61–71. doi:10.1016/0166-0934(91)90008-N.
- ISO20776/1-2006, Clinical laboratory testing and in vitro diagnostic test systems—susceptibility testing of infectious agents and evaluation of performance of antimicrobial susceptibility test devices - Part 1: reference method for testing the in vitro activity of antimicrobial agents against rapidly growing aerobic bacteria involved in infectious diseases, 2006, p. 19. European Committee for Standardization (CEN), Technical Committee CEN/TC 140, Technical Committee ISO/TC 212.
- EUCAST [Internet]. Clinical breakpoints. Breakpoint tables for bacteria. European Society of Clinical Microbiology and Infectious Diseases; 2018. [updated 1 January 2019]. Available from: http://www.eucast.org/clinical_breakpoints/
- Stepanovic S, Vukovic D, Dakic I, et al. A modified microtiter-plate test for quantification of staphylococcal biofilm formation. J Microbiol Methods. 2000;40:175–179.
- Lyu SY, Rhim JY, Park WB. Antiherpetic activities of flavonoids against herpes simplex virus type 1 (HSV-1) and type 2 (HSV-2) in vitro. Arch Pharm Res. 2005;28:1293–1301.
- Liu HY, Peng HY, Hsu SL, et al. Chemical characterization and antioxidative activity of four 3-hydroxyl-3-methylglutaroyl (HMG)-substituted flavonoid glycosides from Graptopetalum Paraguayense E. Walther. Bot Stud. 2015;56:8.
- Wu S-C, Su Y-S, Cheng H-Y. Antioxidant properties of Lactobacillus-fermented and non-fermented Graptopetalum paraguayense E. Walther at different stages of maturity. Food Chem. 2011;129:804–809. doi:10.1016/j.foodchem.2011.05.025.
- Varadinova T, Vilhelmova N, Badenas F, et al. Effect of metal complexes of acyclovir and its acetylated derivative on Herpes simplex virus 1 and Herpes simplex virus 2 replication. Acta Virol. 2005;49:251–260.
- Duh P-D, Lin S-L, Wu S-C. Hepatoprotection of Graptopetalum paraguayense E. Walther on CCl4-induced liver damage and inflammation . J Ethnopharmacol. 2011;134:379–385.
- Elion GB. Mechanism of action and selectivity of acyclovir. Am J Med. 1982;73:7–13.
- Mudi SY, Ibrahim H. Activity of Bryophyllum pinnatum S. Kurz extracts on respiratory tract pathogenic bacteria. Bayero J Pure Appl Sci. 2008;1:43–48.
- Sonderholm M, Bjarnsholt T. The consequences of being in an infectious biofilm: microenvironmental conditions governing antibiotic tolerance. Int J Mol Sci. 2017;18:2688.
- Bush K, Bradford PA. Beta-lactams and beta-lactamase inhibitors: an overview. Cold Spring Harb Perspect Med. 2016;6:a025247.