Abstract
The aim of this study was to investigate the protective roles of H2S on acute renal damage in urinary-derived sepsis. Totally 40 male rabbits were randomly divided into the Control, Sham, Sepsis, NaHS and the dl-propargylglycine (PAG) groups. White blood cell counts (WBC), C-reactive protein (CRP), creatinine (Cr) and blood urea nitrogen were determined in peripheral blood samples. Hematoxylin and eosin staining was performed to detect the morphological changes. The mRNA and protein expression levels were detected by semi-quantitative reverse transcriptase PCR (sqRT-PCR) and Western blot analysis. The results showed that, 72 h after surgery, the WBC and CRP levels in the Sepsis group were significantly elevated, and the expression levels of inducible nitric oxide synthase (iNOS) and HO-1 were significantly up-regulated, compared with the Control and Sham groups, indicating renal damage induced by urinary-derived sepsis. Compared with the Sepsis group, the expression levels of iNOS in the renal tissues of the PAG group were significantly up-regulated, whereas the expression levels of HO-1 in the renal tissue were significantly down-regulated, indicating aggravated renal damage. On the other hand, compared with the Sepsis group, the expression levels of iNOS in the renal tissue of the NaHS group were declined, whereas the expression levels of HO-1 were elevated, together with the declined WBC and CRP levels, indicating alleviated renal functions. We could conclude that iNOS and HO-1 expression levels are elevated in the renal tissue of urinary-derived sepsis. H2S could exert protective effects on the urinary-derived sepsis-associated renal damages by down-regulating iNOS expression and up-regulating HO-1 expression.
Introduction
Urinary-derived sepsis is a type of sepsis caused by pathogenic bacteria and/or toxins entering the blood circulation through the urinary tract due to urinary tract infection, accounting for 9%–31% of all the sepsis cases [Citation1]. Under serious conditions, it can lead to septic shock and/or multiple organ dysfunction syndrome (MODS). The kidney is one of the most vulnerable organs in MODS [Citation2], often suffering from acute kidney injury (AKI). The prevalence of urinary-derived sepsis is increasing year by year, and the mortality rate for severe urinary-derived sepsis is as high as 30%–40% [Citation3]. As a serious disease in urology, the pathophysiological mechanisms of urinary-derived sepsis are rather complex, and the disease develops rapidly, with high mortality rate.
The studies of hydrogen sulfide (H2S) under physiological conditions have begun in the early twentieth century, and H2S is now considered the third gas signal molecule following nitric oxide (NO) and carbon monoxide (CO) [Citation4]. Studies have shown that H2S could be synthesized in almost all the organs, including the kidney, with a wide range of biological functions, such as neuroprotection [Citation5], cardioprotection [Citation6,Citation7] and kidney protection [Citation8,Citation9]. Both cystathionine-β-synthase and cystathionine-γ-lyase are expressed in the renal tissues, which participate in the production of endogenous H2S in the kidney, in turn regulating the organ function [Citation10,Citation11]. It has been widely believed that exogenous supplementation of H2S could induce important effects on the body. For instance, in a mouse model of kidney ischemia and reperfusion, treatment with NaHS (an exogenous H2S donor) could reduce the levels of creatinine (Cr) and blood urea nitrogen (BUN) at 24 h after reperfusion [Citation12]. Other studies have also found that H2S can effectively alleviate the renal fibrosis [Citation13] and exert protective effects on the renal epithelial cell injuries and ischemia-reperfusion injury (IRI) in vitro, in a dose-dependent manner [Citation14]. Meanwhile, H2S could also improve the conditions of diabetic and/or hypertensive kidney damage. Our previous studies have also shown that ulinastatin could reduce the urinary-derived sepsis-associated inflammation by up-regulating IL-10 levels and down-regulating TNF-α levels [Citation15]. Moreover, H2S could inhibit the expression levels of NF-κB, to decrease the TNF-α level and increase the IL-10 level, further reducing the renal damage caused by urinary-derived sepsis [Citation16]. On the other hand, reducing the formation of H2S can exacerbate the urinary-derived sepsis-induced inflammatory responses in the renal injuries [Citation17]. However, the underlying complicated mechanisms still need to be investigated in depth.
Nitric oxide synthase (NOS) is a key enzyme in the process of the production of NO from L-arginine. It has been shown that, in AKI animals, the level of NO is significantly increased, and the renal function could also be significantly improved after knocking out the inducable NOS (iNOS) gene [Citation18]. Heme oxygenase (HO) was first discovered in the process of heparin conversion into biliverdin in liver microsomes. HO is the initiating and rate-limiting enzyme in the heme metabolism. HO catalyzes the degradation of heme to produce free divalent iron ions, carbon monoxide (CO) and biliverdin/bilirubin, exerting anti-oxidant and anti-inflammatory effects in the body [Citation19]. In the AKI models caused by IRI, or by obstructive and/or nephrotoxic factors, the transcription and expression of HO-1 would be observed in the kidney [Citation20], exerting protective effects. H2S, NO and CO are important gas signal molecules in humans, and the complex interactions between them and the related biological effects have been hot spots of research in recent years. In the in vivo environment, iNOS and HO-1 participate in the regulation of the production of NO and CO, respectively. The HO-1/CO system is inextricably linked to the NOS/NO system, working as a complementary system [Citation21]. Moreover, there may also be a cross-relationship between H2S and the HO-1/CO system, as well as the iNOS/NO system. For the urinary-derived sepsis-induced AKI, H2S has no effects on the iNOS/NO and HO-1/CO systems in the renal tissue, with, however, relatively few reports.
In this study, to investigate the protective role of H2S in the urinary-derived sepsis-induced renal injuries and the possible underlying mechanisms, we used a rabbit model of urinary-derived sepsis induced by acute upper urinary tract obstruction and infection. The effects of H2S on the expression levels of iNOs and HO-1 in the renal tissues of these model animals were analyzed.
Materials and methods
Study animals
A total of 40 healthy male rabbits, weighing 2.0–2.4 kg, were purchased from the Animal Experimental Center of the University of South China (Hengyang, Hunan, China). Before animal experiments, these animals were allowed for acclimatization for 1 week, with free access to food and water.
Ethics statement
All animal experiments in this study were approved by the Ethics Committee of the University of South China.
Bacterial strains
The Escherichia coli strain (ATCC 25922; the Second Affiliated Hospital of the University of South China, Hengyang, Hunan, China) was inoculated on blood plate medium (Antu Bioengineering, Zhengzhou, Henan, China) and cultured at room temperature (35–37 °C) for 18–24 h. A typical positive colony was picked up, and was used to prepare the bacterial suspension at a concentration of 105/mL.
Model establishment and animal grouping
To establish the urinary-derived sepsis model, the rabbits were anesthetized with 10% chloral hydrate (at a dose of 3.5 mL/kg body weight). After iodine sterilization, a 4-cm incision was made to the side of the rectus abdominis muscle at the left lower abdomen. The abdominal cavity was cut open, and the left ureter was found along the anterior edge of the left psoas muscle. The middle segment of the ureter was freed, and then ligated with a 1# silk thread. E. coli suspension (0.5 mL/kg body weight) was injected into the ureteral lumen at the ligation site near the renal pelvis. Then the incisions were sutured up layer by layer.
The 40 healthy male rabbits were randomly divided into the following 5 groups (n = 8 per group): (1) the Control group, in which the rabbits received no surgical treatments; (2) the Sepsis group, in which the rabbits were subjected to the urinary-derived sepsis model establishment as described above; (3) the Sham group, in which the rabbits received the same procedures as the Sepsis group, except for lacking the injection of the E. coli suspension; (4) the dl-propargylglycine (PAG) group, in which the rabbit models of urinary-derived sepsis were treated with propargylglycine (i.p.; 37.5 mg/kg in a single dose, immediately after closing the cut; Molbase, Shanghai, China) [Citation17]; and (5) the NaHS group, in which the rabbit models of urinary-derived sepsis were injected (in a single dose, immediately after the surgery) with the 50 mmol/L NaHS (Efirm Biochemistry, Binzhou, Shandong, China) solution through the ear vein (to obtain a final dosage of 8.4 μmol/kg).
Blood index determination
Peripheral blood samples of rabbits were obtained before surgery and at 24, 48, and 72 h after surgery, respectively. After centrifugation, the white blood cell (WBC) counts were determined with an automatic modular blood and body fluid analyzer (XN-10[B4]; Sysmex, Kobe, Japan). The levels of creatinine (Cr), BUN, and C-reactive protein (CRP) were detected with a biochemical analyzer (7180 with ions)).
Hematoxylin and eosin (H&E) staining
At 72 h after surgery, the rabbits were sacrificed, and the left kidney tissue was removed and fixed in 10% formalin solution for 48 h. After embedding in paraffin, the tissue was cut into 5-μm section series on a microtome. The sections were dewaxed with xylene and alcohol gradients, and then stained with H&E. After washing, the section was covered with a cover slip and sealed, and then observed under a microscope (CX31-12LO2; Olympus, Tokyo, Japan).
Western blot analysis
After the rabbits were sacrificed, the renal tissue was harvested and homogenized. Then the sample was lysed with the RIPA lysis buffer. After centrifugation, the total protein concentration was measured with the bicinchoninic acid method. Totally 50-μg protein samples were separated with 10% sodium dodecyl sulfate polyacrylamide gel electrophoresis (SDS-PAGE), and then electronically transferred onto a polyvinylidene fluoride membrane. After blocking with 5% non-fat milk, the membrane was incubated with rabbit anti-rabbit anti-iNOS (1:250 dilution; Abcam, Cambridge, UK), mouse anti-rabbit anti-HO-1 (1:1000 dilution; Novus, Littleton, CO, USA), and mouse anti-rabbit anti-β-actin (1:5000 dilution) primary antibodies, respectively, at 4 °C overnight. Then the membrane was incubated with horseradish peroxidase (HRP)-conjugated goat anti-rabbit or anti-mouse secondary antibodies (1:5000 dilution; Proteintech, Chicago, IL, USA) at room temperature for 90 min. Color development was observed with the enhanced chemiluminescence method, and the protein band images were scanned and analyzed with the Quantity One software.
Semi-quantitative reverse transcription and polymerase chain reaction (SqRT-PCR)
The renal tissue sample was obtained, and the total RNA was extracted with Trizol. The cDNA template was obtained with RT-PCR. Then the SqRT-PCR was performed with SYBGREEN PCR Mix (Invitrogen, Carlsbad, CA, USA). The primer sequences were as follows: iNOS, forward 5′-AGCTCTTTGAAAGATCGACCCGCCCGACA-3′ and reverse 5′-CTACCATGAGCGCCTTGTGCCTCGTGA-3′; HO-1, forward 5′-GTTACCGGGTAAGGAACCATGGCCAGTCG-3′ and reverse 5′-CCACCACCCTCTAAGCTGTGACCCG-3′; and β-actin, forward 5′-AGCATTCCAGTTCACGATCTCCGTTCG-3′ and reverse 5′-GATCGGGTACTATGAACCGGTGCCA-3′. The 30-μL PCR system consisted of 1 μL template, 0.5 μL primer each (10 μmol/L), 16 μL 2× ES Master Mix and 12 μL ddH2O. The reaction conditions were set as follows: 95 °C for 8 min; 95 °C for 12 s, 60 °C for 1 min, for 45 cycles in total. The expression levels of the target genes were normalized with β-actin as the internal reference.
Statistical analysis
Data were expressed as mean values with standard deviation (±SD). The SPSS 20.0 software was used for statistical analysis. Variance analysis with repeated measure design was used for the comparison of data at different time points between groups. One-way analysis of variance (ANOVA) was used for comparison between groups, and the LSD t test was used for multiple comparison. P < 0.05 was considered statistically significant.
Results and discussion
Analysis of rabbit WBC
The WBC counts in the peripheral blood of the experimental animals were analyzed and compared. The results showed significant differences in the peripheral blood WBC counts between the experimental groups (F = 1630.099; P < 0.01). In each group, the peripheral blood WBC changed in a time-dependent manner (F = 2271.671; P < 0.01), indicating an interacting effect between grouping and time (F = 453.807; P < 0.01). Moreover, before surgery, there was no significant difference in the WBC counts between the groups (P = 0.361). At 24, 48, and 72 h after surgery, the WBC counts in the Sepsis group were significantly higher than those in the Control and Sham groups (P = 0.000), whereas there were no significant differences in the WBC counts between the Control and Sham groups at these time points (P = 0.931). Furthermore, at 24, 48, and 72 h after surgery, the WBC counts in the PAG group were significantly higher (P = 0.000), whereas the WBC counts in the NaHS group were significantly lower (P = 0.000) than the Sepsis group (). These results suggest that the white blood cells are elevated in the Sepsis group, and more increased in the PAG group. The leukocytes would be decreased after the NaHS intervention compared with the sepsis and PAG groups.
Table 1. WBC counts at indicated time points in the experimental groups.
Analysis of peripheral blood CRP
The peripheral blood levels of CRP were next analyzed and compared between the experimental groups. Our results showed significant differences in the peripheral blood CRP levels between these groups (F = 2146.009; P < 0.01), and in each group, the peripheral blood CRP levels followed a time-dependent trend (F = 5909.168; P < 0.01), indicating an interacting effect between grouping and time (F = 1111.882; P < 0.01). Moreover, before surgery, there was no significant difference in the peripheral CRP levels between these groups (P = 0.999). At 24, 48, and 72 h after surgery, the peripheral blood CRP levels in the Sepsis group were significantly higher than those in the Control and Sham groups (P = 0.000), whereas there were no significant differences in the CRP levels between the Control and Sham groups at these time points (P = 0.632). Furthermore, at 24, 48, and 72 h after surgery, the peripheral blood CRP levels in the PAG group were significantly higher (P = 0.000), whereas the peripheral blood CRP levels in the NaHS group were significantly lower (P = 0.000) than in the Sepsis group (). These results suggest that the CRP level in the PAG group was significantly higher than that in the Sepsis group. After the NaHS intervention, the blood CRP level was decreased compared with the sepsis and PAG groups.
Table 2. CRP levels at indicated time points in the experimental groups.
Analysis of renal function indices (Cr and BUN)
The peripheral blood levels of Cr and BUN (as the renal function indices) were next analyzed and compared between these groups. Our results showed significant differences in the peripheral blood Cr levels between these groups (F = 921.089; P<0.01). At each time point after surgery, the peripheral levels of Cr in the Sepsis group were significantly higher than those in the Control and Sham groups (both P < 0.05), whereas there were no significant differences in the peripheral blood Cr levels between the Control and Sham groups (both P > 0.05). In each group, the peripheral blood levels of Cr changed along with the advancement time (F = 1065.006; P < 0.001). In specific, the peripheral blood Cr levels in the Sepsis group showed a time-dependent increase. There was a significant interacting effect between the grouping and time (F = 221.971; P < 0.001). Moreover, before surgery, there was no significant difference in the peripheral blood levels of Cr between these groups (P = 0.993). At 24, 48, and 72 h after surgery, the peripheral blood Cr levels in the Sepsis group were significantly higher than those in the Control and Sham groups (P = 0.000), whereas there were no significant differences in the Cr levels between the Control and Sham groups at these time points (P = 0.989). Furthermore, at 24, 48, and 72 h after surgery, the peripheral blood Cr levels in the PAG group were significantly higher (P = 0.000), whereas the peripheral blood Cr levels in the NaHS group were significantly lower (P = 0.000) than the Sepsis group ().
Table 3. Peripheral blood Cr levels at indicated time points in the experimental groups.
On the other hand, there were significant differences in the peripheral BUN levels between the groups (F = 1223.684; P<0.01). At each time point after surgery, the peripheral BUN levels in the Sepsis group were significantly higher than those in the Control and Sham groups (both P < 0.05), whereas no significant differences were observed in the peripheral BUN levels between the Control and Sham groups (both P > 0.05). In each group, the peripheral BUN levels changed with time (F = 2886.252; P < 0.001). More specifically, the peripheral BUN levels in the Sepsis group increased with time. There was a significant interacting effect between the grouping and time (F = 568.217; P < 0.001). What is more, before surgery, there was no significant difference in the peripheral BUN levels between these experimental groups (P = 0.996). At 24, 48, and 72 h after surgery, the peripheral BUN levels in the Sepsis group were significantly higher than those in the Control and Sham groups (P = 0.000), whereas there were no significant differences in the peripheral BUN levels between the Control and Sham groups at these time points (P = 0.660). Furthermore, at 24, 48, and 72 h after surgery, the peripheral BUN levels in the PAG group were significantly higher (P = 0.000), whereas the peripheral BUN levels in the NaHS group were significantly lower (P = 0.000) than in the Sepsis group (). Taken together, these results suggest that the renal dysfunction was significant in the Sepsis group, and the Cr and BUN levels were further elevated in the PAG group. The NaHS intervention significantly alleviated the renal function damage in the sepsis and PAG groups.
Table 4. Peripheral blood BUN levels at indicated time points in the experimental groups.
Analysis of rabbit renal tissue morphology
To investigate the morphological changes in the renal tissues, we performed H&E staining. At 72 h after surgery, in the Control and Sham groups, there were no significant abnormal changes in the cellular morphology in the glomeruli and renal tubules, with no significant differences in the morphological features between these two groups. However, in the Sepsis group, we observed significant pathological changes in the morphology of renal cells, and the following features were detected: glomerular deformation, edema, and hyperemia, with cystic enlargement; renal tubular epithelial cells showing edema and necrosis, with luminal dilatation; and renal interstitial edema, with neutrophil infiltration. Furthermore, compared with the Sepsis group, in the PAG group, the pathological changes in the renal tissue structure and cellular morphology were significantly aggravated. On the other hand, compared with the Sepsis group, in the NaHS group, the pathological changes in the renal tissue structure and cellular morphology were significantly alleviated (). These results suggest that pathological and morphological changes are observed in the renal tissue in the Sepsis group, which are aggravated in the PAG group. The changes could be alleviated by NaHS treatment.
Expression levels of iNOS and HO-1 in rabbit renal tissues
To investigate the mRNA and protein expression levels of iNOS and HO-1 in the renal tissues, we used SqRT-PCR and Western blot analysis. At 72 h after surgery, there were no significant differences in the mRNA/protein expression levels of iNOS or HO-1 in the renal tissues between the Control and Sham groups (P > 0.05). However, at 72 h after surgery, the mRNA and protein expression levels of iNOS and HO-1 in the Sepsis group were significantly higher than those in the Control and Sham groups (P < 0.05). Moreover, at 72 h after surgery, compared with the Sepsis group, the mRNA and protein expression levels of iNOS were significantly elevated in the PAG group (P < 0.05), but significantly decreased in the NaHS group (P < 0.05). Furthermore, at 72 h after surgery, compared with the Sepsis group, the mRNA and protein expression levels of iNOS were significantly decreased in the PAG group (P < 0.05), but significantly elevated in the NaHS group (P < 0.05; and ). Taken together, these results suggest that the expression levels of iNOS and HO-1 were elevated in the Sepsis group. The iNOS expression was elevated, whereas the HO-1 expression was decreased in the PAG group. The changes could be alleviated by treatment with NaHS.
Figure 2. Expression levels of iNOS in renal tissues. The mRNA (A) and protein (B) expression levels of iNOS were detected with SqRT-PCR and Western blot analysis, respectively. Compared with the Control group, *P < 0.05; compared with the Sepsis group, #P < 0.05.
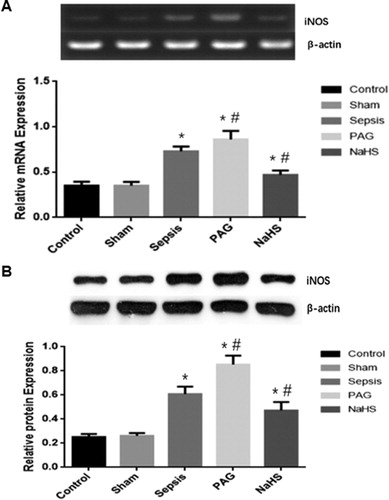
Figure 3. Expression levels of HO-1 in renal tissues. The mRNA (A) and protein (B) expression levels of HO-1 were detected with SqRT-PCR and Western blot analysis, respectively. Compared with the Control group, *P < 0.05; compared with the Sepsis group, #P < 0.05.
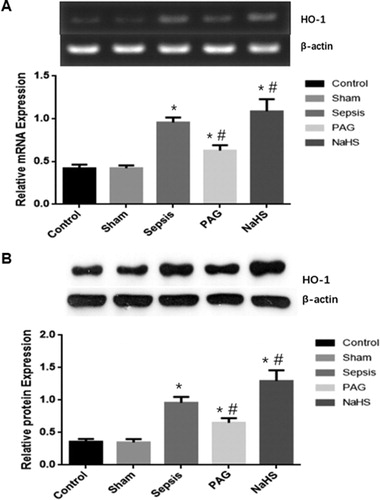
According to the International Consensus on the Definition of Sepsis and Septic Shock (2016), sepsis is defined as a life-threatening organ dysfunction caused by the host’s specific responses to infection [Citation22]. Sepsis is the most common cause of AKI. AKI caused by sepsis accounts for 26%–50% of all the AKI cases in the developed countries, whereas the incidence of primary nephropathy-associated AKI is only 7%–10% [Citation23]. Clinical and basic evidence suggests that, unlike AKI cases induced by other causes, sepsis-associated AKI is driven by a variety of characteristic pathophysiological mechanisms. Studies have reported that 9%–40% of patients with sepsis eventually develop AKI, and the mortality rate for patients with sepsis complicated with AKI is as high as 70% [Citation24,Citation25], significantly higher than the cases only with sepsis. Early aggressive treatment of AKI can reduce the mortality of patients with sepsis [Citation26]. In septic shock patients with severe acute kidney injuries, there was no significant difference in the 90-day overall mortality between patients receiving early or late complete renal replacement therapy [Citation27]. Therefore, it is of great significance to develop effective new therapeutic strategies that could effectively prevent and alleviate the sepsis-induced AKI.
In our previous study, the urinary-derived sepsis model was established by the pelvis high-pressure method (i.e. ligating the left ureter and injecting E. coli) [Citation16], which simulates the main pathogenic characteristics of clinical cases of sepsis, contributing to investigating the disease development and pathogenic features. In this study, based on the established rabbit models and the experimental procedures, a related investigation was performed. In the early stage of sepsis, AKI would be induced, in which the renal function is the direct reflection of the disease AKI severity. In clinical and basic research, the indicators of Cr and BUN are commonly used to evaluate the renal function. CRP is an acute phase protein associated with inflammatory responses, which is a sensitive indicator for bacterial infection. In addition, CRP is a risk factor for sepsis [Citation28], and has value in predicting the disease severity and prognosis of urinary-derived sepsis. In this study, our results showed that, at 72 h after surgery, the WBC and CRP levels in the Sepsis group were significantly elevated, indicating significantly decreased renal function and pathological alterations, as typical symptoms of sepsis-associated acute kidney injuries.
NOS is a key enzyme in the NO synthesis in vivo. iNOS is a non-calcium-dependent NOS widely distributed in the endothelial cells and macrophages. Under physiological conditions, iNOS is always inactive, and could be activated under pathological conditions, inducing the production of a large amount of endogenous NO. NO is involved in the waterfall of inflammatory mediators, and the excessive release of NO usually leads to organ failure. High expression of iNOS could produce excessive NO, which is closely related to systemic infection with circulatory failure [Citation29]. In sepsis, iNOS is activated and expressed in large amounts [Citation30]. In the biochemical parameters associated with sepsis, excessive NO production leads to the decreased vascular tone and consequent hypotension [Citation31]. Quoilin et al. [Citation32] have used the lipopolysaccharide (LPS) treatment to induce the elevated expression of iNOS and NADPH oxidase 4 (NOX-4), which further increases the NO and superoxide anion. These changes would interrupt the mitochondrial oxidative phosphorylation by reducing the cytochrome C oxidase activity, to induce mitochondrial dysfunction. These findings demonstrate that treatment with iNOS inhibitors or antioxidants could maintain the cytochrome C oxidase activity, and prevent the mitochondrial membrane potential dissipation, thus protecting against acute sepsis. It has been shown that the iNOS levels in the Sepsis group are significantly increased, suggesting that iNOS aggravates the disease progression in sepsis [Citation30]. Therefore, inhibiting the expression of iNOS in septic rats could improve the oxygen supply to the tissues and reduce the tissue damage. In this study, our results showed that, at 72 h after surgery, no significant differences in the mRNA and protein expression levels of iNOS were observed between the Control and Sham groups. Moreover, the mRNA and protein expression levels of iNOS in the Sepsis group were significantly higher than the Control and Sham groups. Therefore, the up-regulated expression of iNOS might be one of the most important mechanisms underlying the acute renal injuries caused by urinary-derived sepsis.
HO-1 is a stress response protein and key enzyme in the process of heme metabolism. HO-1 and its products play a wide range of roles in the cellular protection, with anti-inflammatory [Citation33] and antioxidant [Citation34] effects. Recent studies have shown that HO-1 is a representative cytoprotective enzyme preventing oxidative stress and inflammation [Citation35]. It has been shown that under oxidative stress conditions, HO-1 would be activated and expressed, thereby enhancing the body’s antioxidant and anti-inflammatory effects [Citation36,Citation37]. Konrad et al. [Citation38] have shown that HO-1 exerts anti-inflammatory effects in the LPS-induced pneumonia model. Previous studies have shown that HO-1-deficient children have significant endothelial damage and sensitivity to oxidative and inflammatory damage, which might eventually lead to death in childhood [Citation39,Citation40]. Similarly, HO-1 knockout mice have increased sensitivity to oxidative stress and increased susceptibility to injuries [Citation41]. A large number of studies have found that the expression levels of HO-1 are increased in sepsis, which may protect important organs and tissues. Studies have shown that the enhancement of HO-1 induction in the renal tubular cells and lung macrophages can block the acute renal failure and acute respiratory distress syndrome in sepsis in mice with sepsis, whereas inhibition of HO-1 expression can reduce the protective effects of hepatocyte growth factor on the organ damage induced by LPS [Citation42]. Nath et al. [Citation43] have established the rhabdomyolysis-induced AKI rat model, and for the first time have shown that HO-1 could inhibit apoptosis and decrease AKI, significantly reducing the mortality of AKI rats. HO-1 plays a key role in maintaining the renal function and protecting kidney structure, and is also used as a biological marker for human AKI [Citation43]. The in vitro experiments have revealed that the HO-1 over-expression achieved by gene modification could improve the proliferation of bone marrow mesenchymal stem cells under AKI microenvironment, reduce the apoptotic processes and increase the transdifferentiation into renal tubular epithelial cells, facilitating the AKI repair. Up-regulation of HO-1 protein followed by CO increase could protect AKI caused by septic shock in rats [Citation44]. The glycerol models of AKI show strong induction of HO-1 mRNA and increase the HO activity [Citation45]. HO-1/CO exerts cytoprotective effects on the end organ damages during endotoxin shock [Citation46]. HO-1 is a therapeutic target for AKI, and the beneficial effects induced by HO-1 during AKI are partially mediated by by-products of the HO reaction [Citation47]. Increasing evidence has shown that HO-1 has strong organ protecting effects. In this study, our results showed that the mRNA and protein levels of HO-1 in the Sepsis group were significantly higher than in the Control and Sham groups, at 72 h after surgery, suggesting that HO-1 may play an adaptive protecting role in the acute renal injuries of sepsis.
H2S has protective effects on kidney and diaphragm of rats with type I diabetes, and the underlying mechanism is related to down-regulation of iNOS expression and reduction of NO production in the tissues [Citation48]. Some in vitro studies have shown that the HO-1 expression is significantly increased after treatment with H2S donors in the mesangial cells and podocytes, indicating that H2S plays a role in regulating the HO-1 expression, and the underlying mechanisms might be related to the up-regulated antioxidant enzymes [Citation49]. H2S can affect the expression levels of both iNOS and HO-1 in the tissues and thus exert the anti-inflammatory effects. It has been shown that, in a LPS-induced mouse macrophage inflammatory responses, H2S can activate the HO-1/CO system in macrophages, which mediates the inhibitory effects of H2S on iNOS/NO [Citation50]. H2S can protect the heart of mouse models with myocarditis induced by Coxsachie virus B3 (CVB3) by inhibiting the up-regulated mRNA and protein expression levels of iNOS, and inducing the mRNA and protein expression levels of HO-1 [Citation51]. In this study, our results showed that at 72 h after surgery, the WBC and CRP levels in the Sepsis group were significantly higher than in the Control and Sham groups, indicating renal function impairment, and the mRNA and protein expression levels of iNOS and HO-1 were also upregulated in the Sepsis group. Moreover, our results showed that, in the PAG group, the mRNA and protein expression levels of iNOS were up-regulated, while the mRNA and protein expression levels of HO-1 were decreased, indicating the aggravated pathological changes of the renal tissue. In addition, the elevation of WBC and CRP was more significant, suggesting that PAG can be applied to inhibit the production of endogenous H2S, and PAG aggravates the renal damages caused by urinary-derived sepsis. In the NaHS treatment, the exogenous NaHS supplementation significantly decreased the mRNA and protein expression levels of iNOS in the renal tissue, and increased the mRNA and protein expression levels of HO-1, together with declined WBC and CRP levels, indicating alleviated pathological changes of renal tissues. These results suggest that exogenous H2S supplementation could improve the renal damage caused by urinary-derived sepsis. As the H2S donor, NaHS promoted the mRNA and protein expression levels of HO-1 in the renal tissue of urinary-derived sepsis, and inhibited the mRNA and protein expression levels of iNOS, thus protecting the kidney, while the PAG treatment significantly decreased the mRNA and protein expression levels of HO-1 and elevated the mRNA and protein expression levels of iNOS, aggravating the renal damages.
Conclusions
The results from this study showed that the expression levels of iNOS and HO-1 were up-regulated in the renal tissue of urinary-derived sepsis. H2S could alleviate the urinary-derived sepsis-induced acute renal injuries, and the possible mechanism may be related to the down-regulation of iNOS and up-regulation of HO-1.
Disclosure statement
All authors declare no financial/non-financial competing interests.
Additional information
Funding
References
- Levy MM, Artigas A, Phillips GS, et al. Outcomes of the Surviving Sepsis Campaign in intensive care units in the USA and Europe: a prospective cohort study. Lancet Infect Dis. 2012;12(12):919–924.
- Poukkanen M, Vaara ST, Pettila V, et al. Acute kidney injury in patients with severe sepsis in Finnish Intensive Care Units. Acta Anaesthesiol Scand. 2013;57(7):863–872.
- Zhou J, Qian C, Zhao M, et al. Epidemiology and outcome of severe sepsis and septic shock in intensive care units in mainland China. PLoS One. 2014;9(9):e107181.
- Wang R. Hydrogen sulfide: the third gasotransmitter in biology and medicine. Antioxidants Redox Signal. 2010;12(9):1061.
- Wei X, Zhang B, Cheng L, et al. Hydrogen sulfide induces neuroprotection against experimental stroke in rats by down-regulation of AQP4 via activating PKC. Brain Res. 2015;1622:292–299.
- Pan TT, Chen YQ, Bian JS. All in the timing: a comparison between the cardioprotection induced by HS preconditioning and post-infarction treatment. Eur J Pharmacol. 2009;616(1–3):160–165.
- Li L, Li M, Li Y, et al. Exogenous H2S contributes to recovery of ischemic post-conditioning-induced cardioprotection by decrease of ROS level via down-regulation of NF-κB and JAK2-STAT3 pathways in the aging cardiomyocytes. Cell Biosci. 2016;6(1):26.
- Kasinath BS, Feliers D, Lee HJ. Hydrogen sulfide as a regulatory factor in kidney health and disease. Biochem Pharmacol. 2018;149:29–41.
- Koning AM, Frenay AR, Leuvenink HG, et al. Hydrogen sulfide in renal physiology, disease and transplantation–the smell of renal protection. Nitric Oxide Biol Chem. 2015;46:37–49.
- Xia M, Chen L, Muh RW, et al. Production and actions of hydrogen sulfide, a novel gaseous bioactive substance, in the kidneys. J Pharmacol Exp Ther.. 2009;329(3):1056–1062.
- Beltowski J. Hypoxia in the renal medulla: implications for hydrogen sulfide signaling. J Pharmacol Exp Ther. 2010;334(2):358–363.
- Tripatara P, Patel NS, Brancaleone V, et al. Characterisation of cystathionine gamma-lyase/hydrogen sulphide pathway in ischaemia/reperfusion injury of the mouse kidney: an in vivo study. Eur J Pharmacol. 2009;606(1–3):205–209.
- Lin S, Visram F, Liu W, et al. GYY4137, a slow-releasing hydrogen sulfide donor, ameliorates renal damage associated with chronic obstructive uropathy. J Urol. 2016;196(6):1778–1787.
- Ahmad A, Olah G, Szczesny B, et al. AP39, a mitochondrially targeted hydrogen sulfide donor, exerts protective effects in renal epithelial cells subjected to oxidative stress in vitro and in acute renal injury in vivo. Shock (Augusta, GA). 2016;45(1):88–97.
- Chen X, Wang Y, Luo H, et al. Ulinastatin reduces urinary sepsis related inflammation by upregulating IL10 and downregulating TNFalpha levels. Mol Med Rep. 2013;8(1):29–34.
- Chen X, Xu W, Wang Y, et al. Hydrogen sulfide reduces kidney injury due to urinary-derived sepsis by inhibiting NF-kappaB expression, decreasing TNF-alpha levels and increasing IL-10 levels. Exp Ther Med. 2014;8(2):464–470.
- Qiu H, Chen X, Luo Z, et al. Inhibition of endogenous hydrogen sulfide production exacerbates the inflammatory response during urine-derived sepsis-induced kidney injury. Exp Ther Med. 2018;16(4):2851–2858.
- Pathak E, MacMillan-Crow LA, Mayeux PR. Role of mitochondrial oxidants in an in vitro model of sepsis-induced renal injury. J Pharmacol Exp Ther. 2012;340(1):192–201.
- Sacerdoti D, Pesce P, Di Pascoli M, et al. EETs and HO-1 cross-talk. Prostaglandins Other Lipid Mediat. 2016;125:65–79.
- Zager RA, Johnson AC, Becker K. Plasma and urinary heme oxygenase-1 in AKI. JASN. 2012;23(6):1048–1057.
- Foresti R, Clark JE, Green CJ, et al. Thiol compounds interact with nitric oxide in regulating heme oxygenase-1 induction in endothelial cells. Involvement of superoxide and peroxynitrite anions. J Biol Chem. 1997;272(29):18411.
- Singer M, Deutschman CS, Seymour CW, et al. The third international consensus definitions for sepsis and septic shock (Sepsis-3). Jama. 2016;315(8):801–810.
- Bagshaw SM, George C, Bellomo R. Early acute kidney injury and sepsis: a multicentre evaluation. Critical Care (London, England). 2008;12(2):R47.
- Oppert M, Engel C, Brunkhorst FM, et al. Acute renal failure in patients with severe sepsis and septic shock–a significant independent risk factor for mortality: results from the German Prevalence Study. Nephrol Dial Transplant. 2007;23(3):904–909.
- Mehta RL, Bouchard J, Soroko SB, et al. Sepsis as a cause and consequence of acute kidney injury: program to improve care in acute renal disease. Intensive Care Med. 2011;37(2):241–248.
- Rajapakse S, Rodrigo C, Rajapakse A, et al. Renal replacement therapy in sepsis-induced acute renal failure. Saudi Journal of Kidney Diseases & Transplantation. 2009;20(4):553–559.
- Barbar SD, Clere-Jehl R, Bourredjem A, et al. Timing of renal-replacement therapy in patients with acute kidney injury and sepsis. N Engl J Med. 2018;379(15):1431–1442.
- Albanopoulos K, Alevizos L, Natoudi M, et al. C-reactive protein, white blood cells, and neutrophils as early predictors of postoperative complications in patients undergoing laparoscopic sleeve gastrectomy. Surg Endosc. 2013;27(3):864–871.
- Kirkeboen KA, Strand OA. The role of nitric oxide in sepsis–an overview. Acta Anaesthesiol Scand. 1999;43(3):275–288.
- Li WC, Zou ZJ, Zhou MG, et al. Effects of simvastatin on the expression of inducible NOS in acute lung injury in septic rats. Int J Clin Exp Pathol. 2015;8(11):15106–15111.
- Prauchner CA. Oxidative stress in sepsis: pathophysiological implications justifying antioxidant co-therapy. Burns: J Int Soc Burn Injuries. 2017;43(3):471–485. PubMed PMID: 28034666; eng.
- Quoilin C, Mouithys-Mickalad A, Lecart S, et al. Evidence of oxidative stress and mitochondrial respiratory chain dysfunction in an in vitro model of sepsis-induced kidney injury. Biochim Biophys Acta. 2014;1837(10):1790–1800.
- Kobayashi H, Takeno M, Saito T, et al. Regulatory role of heme oxygenase 1 in inflammation of rheumatoid arthritis. Arthritis Rheum. 2006;54(4):1132–1142.
- Wu CC, Lu KC, Chen JS, et al. HO-1 induction ameliorates experimental murine membranous nephropathy: anti-oxidative, anti-apoptotic and immunomodulatory effects. Nephrol Dial Transplant. 2008;23(10):3082–3090. PubMed PMID: 18477570; eng.
- Marcinkiewicz J, Walczewska M, Olszanecki R, et al. Taurine haloamines and heme oxygenase-1 cooperate in the regulation of inflammation and attenuation of oxidative stress. Adv Exp Med Biol. 2009;643:439–450. [https://doi.org/19239176]
- Ryter SW, Choi AM. Targeting heme oxygenase-1 and carbon monoxide for therapeutic modulation of inflammation. Transl Res J Lab Clin Med. 2016;167(1):7–34.
- Ayer A, Zarjou A, Agarwal A, et al. Heme oxygenases in cardiovascular health and disease. Physiol Rev. 2016;96(4):1449–1508.
- Konrad FM, Knausberg U, Hone R, et al. Tissue heme oxygenase-1 exerts anti-inflammatory effects on LPS-induced pulmonary inflammation. Mucosal Immunol. 2016;9(1):98–111.
- Kawashima A, Oda Y, Yachie A, et al. Heme oxygenase-1 deficiency: the first autopsy case. Human Pathol. 2002;33(1):125–130.
- Yachie A, Niida Y, Wada T, et al. Oxidative stress causes enhanced endothelial cell injury in human heme oxygenase-1 deficiency. J Clin Invest. 1999;103(1):129–135.
- Poss KD, Tonegawa S. Reduced stress defense in heme oxygenase 1-deficient cells. Proc Nat Acad Sci USA. 1997;94(20):10925.
- Kamimoto M, Mizuno S, Matsumoto K, et al. Hepatocyte growth factor prevents multiple organ injuries in endotoxemic mice through a heme oxygenase-1-dependent mechanism. Biochem Biophys Res Commun. 2009;380(2):333–337.
- Yokoyama T, Shimizu M, Ohta K, et al. Urinary heme oxygenase-1 as a sensitive indicator of tubulointerstitial inflammatory damage in various renal diseases. Am J Nephrol. 2011;33(5):414–420.
- Yu JB, Zhou F, Yao SL, et al. Effect of heme oxygenase-1 on the kidney during septic shock in rats. Transl Res J Lab Clin Med. 2009;153(6):283–287.
- Nath KA. The role of renal research in demonstrating the protective properties of heme oxygenase-1. Kidney Int. 2013;84(1):3–6.
- Yu J, Shi J, Wang D, et al. Heme oxygenase-1/carbon monoxide-regulated mitochondrial dynamic equilibrium contributes to the attenuation of endotoxin-induced acute lung injury in rats and in lipopolysaccharide-activated macrophages. Anesthesiology. 2016;125(6):1190–1201.
- Bolisetty S, Zarjou A, Agarwal A. Heme oxygenase 1 as a therapeutic target in acute kidney injury. Am J Kidney Dis Off J Nat Kidney Found. 2017;69(4):531–545.
- Yang R, Jia Q, Liu XF, et al. Effects of hydrogen sulfide on inducible nitric oxide synthase activity and expression of cardiomyocytes in diabetic rats. Mol Med Rep. 2017;16(4):5277–5284.
- D'Araio E, Shaw N, Millward A, et al. Hydrogen sulfide induces heme oxygenase-1 in human kidney cells. Acta Diabetol. 2014;51(1):155–157.
- Oh GS, Pae HO, Lee BS, et al. Hydrogen sulfide inhibits nitric oxide production and nuclear factor-kappaB via heme oxygenase-1 expression in RAW264.7 macrophages stimulated with lipopolysaccharide. Free Rad Biol Med. 2006;41(1):106–119.
- Hua W, Chen Q, Gong F, et al. Cardioprotection of H2S by downregulating iNOS and upregulating HO-1 expression in mice with CVB3-induced myocarditis. Life Sci. 2013;93(24):949–954.