Abstract
Oral squamous cell carcinoma (OSCC) is an aggressive malignancy which is the most common type of head and neck cancer. The study aimed to investigate the role of CXCL14 in cell proliferation, invasion, migration, and epithelial-mesenchymal transition (EMT) of OSCC and its potential mechanisms. In the present study, overexpression of CXCL14 was employed in TCA-8113 cells. Cell proliferation was detected using Cell Counting Kit-8 assay and flow cytometry. Scratch wound healing and Transwell assays were applied for the analysis of cell migration and invasion, respectively. Quantitative real-time polymerase chain reaction analysis was used to explore the mRNA expression of CXCL14 and PD-L1. Moreover, the protein expression levels of CXCL14, E-cadherin, N-cadherin, vimentin, PD-L1, NF-κB p65, and phospho-IκB-α (p-IκB-α) were examined by Western blot. The results revealed that the overexpression of CXCL14 was associated with suppressed proliferation of TCA-8113 cells coupled with decreasing the percentages of cells in the S phase. Moreover, the levels of cell migration and invasion were attenuated as well. Additionally, the expression level of EMT relative protein E-cad was increased notably, whereas N-cad and Vimentin were decreased. Importantly, the mRNA and protein expression of PD-L1, a crucial factor for OSCC, were lowered after overexpression of CXCL14. At the same time, the expression of NF-κB p65 and p-IκB-α was down-regulated significantly. Taken together, we speculate that CXCL14 inhibited OSCC cell proliferation, invasion, migration and EMT via suppressing PD-L1 expression and NF-κB mediated EMT, which indicated that CXCL14 may be an underlying target for OSCC treatment.
Introduction
Oral squamous cell carcinoma (OSCC) is an aggressive malignancy which is the most common type of head and neck cancer [Citation1,Citation2]. It is noted for high morbidity accounting for over 90% of all oral malignancies and high mortality for which the 5-year survival rate is only at 50%–55% [Citation3,Citation4]. Although advances in its treatment are accompanied with multiple new treatment modalities, OSCC still has a poor prognosis which is attributed to the late diagnosis [Citation5]. To the best of our knowledge, understanding the molecular mechanisms for the incidence and development progression of OSCC has clinical implications for the OSCC therapy. It is urgent to ascertain effective therapeutic targets to improve the survival rate of OSCC patients.
CXC chemokine ligand 14 (CXCL14), a CXC-type chemokine known as breast and kidney-expressed chemokine (BRAK), has been implicated in activating B cells and monocytes, maintaining cancer progression [Citation6,Citation7]. It has been well documented that the expression of CXCL14 was relatively high in many normal tissues whereas down-regulated or absent in various types of malignant tumors, which indicated that CXCL14 may exhibit antiangiogenic properties [Citation8–10]. In addition, accumulating evidence shows that CXCL14 overexpression in cancer cells exerts anti-tumor effects [Citation11].
Epithelial-mesenchymal transition (EMT) is a process from which epithelial cells lose their polarity and are converted into a mesenchymal phenotype, which has been suggested as a pivotal step for cancer invasion and metastasis [Citation12,Citation13]. Activation of EMT is related to aberrant expression of a variety of genes. It is commonly characterized by down-regulation of E-cadherin (E-cad), which is a vital epithelial marker, accompanied with up-regulation of N-cadherin (N-cad) and Vimentin, which are crucial mesenchymal marker genes [Citation14,Citation15]. Programmed cell death‐1 ligand 1 (PD‐L1) is a cell surface protein of the B7 family. Mounting evidence suggested that the arbitrary high expression of PD-L1 has been associated with treatment response in OSCC, which might be attributed to the immunosuppressive microenvironment [Citation16–18]. It has been well documented that the inhibition of NF-κB signaling pathway contributes to the reverse EMT [Citation7,Citation19]. Therefore, whether CXCL14 regulates the EMT of OSCC via NF-κB signaling remains unknown and attracts our interest.
The present study aimed to investigate the role of CXCL14 in the proliferation, migration and invasion of OSCC cells. Furthermore overexpression of CXCL14 in TCA-8113 cells was employed to measure the relative variation of EMT in OSCC cells. The result suggested that CXCL14 inhibited OSCC by suppressing PD-L1 and NF-κB expressions which may act as a tumor suppressor and may represent an effective therapeutic strategy in OSCC.
Materials and methods
Cell culture and transfection
The TCA-8113 cell line was purchased from Boster Biological Technology (Wuhan, China) and cultured in Dulbecco's modified Eagle's medium (DMEM; Gibco, Gaithersburg, MD, USA) containing 10% fetal bovine serum (FBS, Gibco) at 37°C in a 5% CO2 humidified incubator. TCA-8113 cells were randomly allocated to three groups: control, vector negative control (vector-NC) and CXCL14 overexpression. Cells were seeded in six-well plates at the density of 1 × 106 cells/well. When grown to 40%–60% confluence, the CXCL14-pcDNA3.1 plasmid and empty vector pcDNA3.1 (BioVector, Beijing, China) were applied for transfection using Lipofectamine 2000 (Invitrogen, Carlsbad, CA) according to the manufacturer's instructions. Then successful transfections were determined by Quantitative real-time polymerase chain reaction (qRT-PCR) and Western blot assay after cells were cultured for 24 h.
Cell proliferation assay
Cell Counting Kit-8 assay (Dojindo Laboratories, Kumamoto, Japan) was employed to detect cell viability. TCA-8113 cells were seeded in 96-well plates (5 × 103 cells/well) and cultured at 37 °C in a 5% CO2 humidified incubator. At the indicated time points (24, 48, and 72 h) after transfection, 10 μL CCK-8 solution was added to each well. Following culture at 37 °C for 1 h, the absorbance was measured with a plate reader at 450 nm.
Cell invasion assay
The ability of invasion was detected by the transwell assay, which was performed in 24-well transwell plates (Corning, NY, USA) with 8-μm-pore inserts coated with Matrigel (BD Biosciences, San Jose, CA, USA). Cells were suspended in serum-free DMEM, and 200 µL of cell suspension (5 × 104) were seeded into the upper chamber. A total of 500 µL of medium supplemented with 15% FBS was added to the lower chamber as a chemoattractant. After 24 h of culture at 37 °C in a humidified 5% CO2 incubator, the Matrigel and the cells remaining in the upper chamber were removed by a cotton-tipped swab. Cells were stained with 1% crystal violet. Images were taken with a microscope (Zeiss, Oberkochen, Germany) and cells were counted under five randomly chosen fields.
Wound healing assay
The scratch wound healing assay was used to evaluate the migration ability of TCA-8113 cells. Before the experiment started, cells were seeded into six-well plates. By reaching a confluence of more than 75% for cultured cells, a 200 μL pipette tip was used to make a wound and then the floating cells were removed by washing with phosphate buffered saline. Then, the cells were cultured in serum-free DMEM and photographed with a camera. An inverted microscope (BX51; Olympus Corporation; 20× magnification) was used to monitor cells at the borders of the scratches. The degree of scratch healing was observed and images were captured in each group at 0 and 24 h.
Flow cytometry
The cell cycle analysis was performed using the Cell Cycle Analysis Kit (Beyotime Biotechnology, Shanghai, China). Following transfection for 48 h, 1 × 105 cells were treated with 70% cold ethanol at 4 °C overnight to increase cell membrane penetrability. Subsequently 100 μg/mL RNase (Keygentec, Nanjing, China) was used to treat cells at 37 °C for 20 min. Following cell staining with 30 μg/mL propidium iodide (Keygentec) for 30 min at room temperature in the dark, cell cycle was analyzed by Gallios Flow Cytometry (Beckman Coulter, Brea, CA, USA). The flow cytometry results were evaluated using a Cell Quest kit (BD Biosciences, San Jose, CA, USA).
Quantitative reverse transcription-PCR (qRT-PCR)
Total RNA was extracted using the TRIzol Reagent (Invitrogen, Carlsbad, CA, USA). RNA was reverse-transcribed to cDNA using the QuantiTect RT kit (Qiagen, Hilden, Germany) following the manufacturer’s instructions. Real-time PCR was performed using a System 7500 instrument (Applied Biosystems, Carlsbad, CA, USA). The mRNA expressiom level of GAPDH (glyceraldehyde 3-phosphate dehydrogenase) was the endogenous control and the expression of CXCL-14 and PD-L1 were normalized to the GAPDH levels. The primer sequences for qPCR were as follows: CXCL-14, forward 5′-CGCTACAGCGACGTGAAGAA-3′ and reverse 5′-GTTCCAGGCGTTGTACCAC-3′; PD-L1, forward 5′-TGGCATTTGCTGAACGCATTT-3′ and reverse 5′-TGCAGCCAGGTCTAATTGTTTT-3′; GAPDH, forward 5′-CTCACCGGATGCACCAATGTT-3′ and reverse 5′-CGCGTTGCTCACAATGTTCAT-3′. The results were analyzed following the 2‐ΔΔCq calculation method [Citation20].
Western blotting
TCA-8113 cell proteins were collected and lysed with RIPA lysis buffer (Beyotime Biotechnology) and cultured for 30 min on ice subsequently. The protein concentration was detected by using an Enhanced BCA Protein Assay Kit (Beyotime Biotechnology). Forty micrograms total protein samples were isolated by sodium dodecyl sulfate-polyacrylamide gels and electroblotted onto a polyvinylidene difluoride membrane. All membranes were then blocked with 5% defatted milk, and then incubated with primary antibodies. Subsequently membranes were washed with TBST three times and incubated with horseradish peroxidase-labeled Goat Anti-Mouse IgG (H + L) antibody (A0216, Beyotime Biotechnology). All blots were then developed with an enhanced chemiluminescence reagent and analyzed by ImageJ software, which was normalized by GAPDH. Anti-CXCL14 (ab46010) was the product of Abcam Company (Cambridge, UK). Anti-E-cadherin (3195S), anti-N-cadherin (13116S), anti-Vimentin (3300 T), anti-PD-L1 (13684S), anti-NF-κB (8242S), anti-p-I-κB-α (2859S), and anti-GAPDH (5174S) antibodies were obtained from Cell Signaling Technology (Boston, MA, USA).
Statistical analysis
Statistical data analysis was performed with SPSS 22.0 and GraphPad Prism 5.0. All experimental results are expressed as mean values with standard deviation (±SD). Difference analysis was performed with the two-tailed Student’s t test and analysis of variance (ANOVA). P < 0.05 was deemed statistically significant.
Results and discussion
Metastasis is a symbolic feature of cancer, which is becoming a dominating obstacle in enhancing the survival rates of patients with OSCC because of the high rate of distant metastasis [Citation21]. The lymphatic system and blood vessels are invaded by a portion of tumor cells, which then attack tissues far from the tumor lesion. To the best of our knowledge, the process of cell proliferation, invasion and migration is essential for cancer metastasis. Therefore, interdiction of this progress is an efficient strategy to resist OSCC metastasis [Citation22,Citation23].
Previous studies showed that the expression of CXCL14 is remarkably decreased in OSCC cells, and its up-regulation suppressed tumor cell growth in vivo [Citation24,Citation25], but the underlying mechanism still needs to be understood. To explore whether CXCL14 serves a role as a tumor suppressor in OSCC, overexpression of CXCL14 was employed to detect the proliferation of OSCC cells. TCA-8113 cells were transfected with empty vector pcDNA3.1 (negative control, NC) or CXCL14-pcDNA3.1 plasmid (CXCL14 mimic), and the expression of CXCL14 was measured by Western blot or qRT-PCR. As shown in , the protein and mRNA expression levels of CXCL14 were increased significantly in the CXCL14 mimic group. The cell proliferation was assessed by Cell Counting Kit-8 assay and Flow cytometry. Analysis of the results indicated that CXCL14 overexpression was associated with suppressed TCA-8113 cell proliferation compared with the NC group (. In addition, the percentages of cells in the G1 phase was increased, while the percentages of cells in S and G2 phases were decreased, which demonstrated a cycle arrest (. The above data suggested that CXCL14 overexpression could impede the proliferation of TCA-8113 cells. Moreover, the invasion and migration of TCA-8113 cells were measured in our study by Transwell assay and wound healing assay respectively. From the results, we found that overexpression of CXCL14 was associated with suppressed TCA-8113 cell invasion and migration compared with the NC group (). The results of the present study suggested that overexpression of CXCL14 led to suppressed proliferation, invasion and migration significantly of OSCC cells.
Figure 1. Protein (A) and mRNA (B) expression levels of CXCL14 after overexpression of CXCL14 in TCA-8113 cells. (A) The protein expression level of CXCL14 was determined by Western blot. (B) The mRNA expression level of CXCL14 was measured by qRT-PCR. **P < 0.01, ***P < 0.001 versus NC. NC, negative control. All results were confirmed in at least three independent experiments.
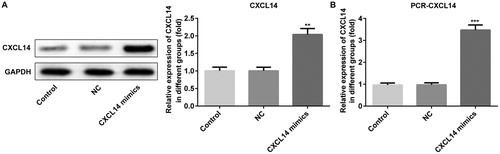
Figure 2. Effects of CXCL14 overexpression on proliferation of TCA-8113 cells. (A) Cell Counting Kit-8 assay was used to detect proliferation of TCA-8113 cells. Cell cycle analysis (B) and Cell cycle distribution (%) (C) of TCA-8113 cells after CXCL14 overexpression. **P < 0.01, ***P < 0.001 versus NC. NC, negative control. All results were confirmed in at least three independent experiments.
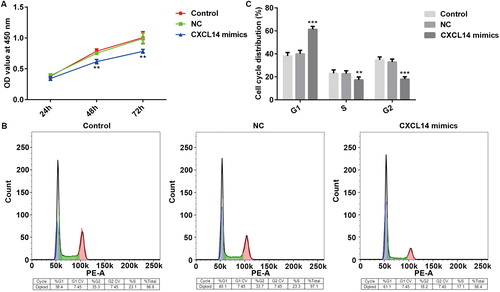
Figure 3. Changes of cell migration and invasion after overexpression of CXCL14 in TCA-8113 cells. (A) Cell migration was measured by wound healing assay (magnification, ×20). (B) Number of migrated TCA-8113 cells after 24 h culture. (C) Images of cell invasion of TCA-8113 cells (magnification, ×100). (D) Number of invading cells as determined with a counting chamber. **P < 0.01 versus NC. NC, negative control. All results were confirmed in at least three independent experiments.
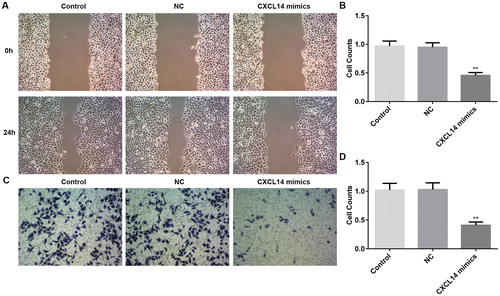
The EMT has been deemed as a vital stage in regulating the initial phase of OSCC metastasis, and the significance of EMT in mediating aggressiveness in the progression of OSCC has drawn more and more attention in recent years [Citation26]. Mounting evidence indicates that the cancer-associated EMT process accelerates cancer cell invasion and migration in vivo and in vitro [Citation26]. A previous study demonstrated that inhibition of EMT is an intriguing therapeutic strategy which can remarkably improve the disease development and the outcome of cancer patients attributing to its clinical importance [Citation27]. It is well known that there is downregulation of E-cad and upregulation of N-cad and Vimentin, which are all EMT marker proteins, in OSCC cells [Citation28]. In our study, as shown in , the expression of E-cad was up-regulated notably after CXCL14 overexpression, whereas the levels of N-cad and Vimentin were down-regulated, which was in accordance with the previous study [Citation28]. Our finding confirmed CXCL14 as a pivotal regulator of the EMT in OSCC cells.
Figure 4. Protein expression levels of E-cad, N-cad, and Vimentin detected by Western blot. *P < 0.05, **P < 0.01, ***P < 0.001 versus NC. NC, negative control. All results were confirmed in at least three independent experiments.
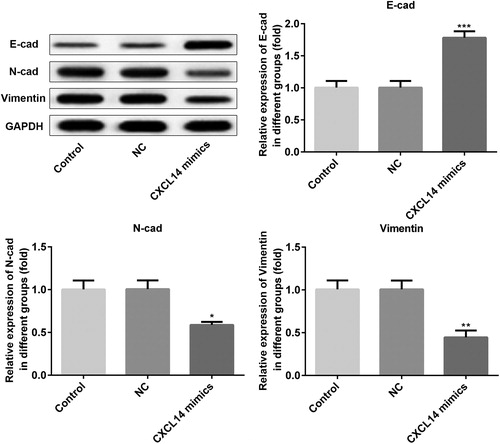
Previous studies have indicated that the expression of PD-L1 is inducible and essential in the cells of many malignancies [Citation29,Citation30]. It has been well documented that high PD-L1 expression in head and neck squamous cell carcinoma cells is associated with poor prognosis [Citation31]. Our present results suggested that overexpression of CXCL14 obviously attenuates the expression of PD-L1 (). Moreover, the NF-κB pathway plays an important role in EMT, and emerging evidence indicates that CXCL14 can inhibit the EMT of colorectal cancer by suppressing NF-κB signaling [Citation32]. And activation of CXCL14/NF-κB signaling contributes to osteosarcoma metastasis [Citation33]. In order to investigate whether EMT occurrence in OSCC cells was regulated via the NF-κB signaling pathway, the NF-κB signal was detected at the same time. As displayed in , the expression of NF-κB and p-IκB-α were down-regulated significantly after CXCL14 overexpression. Collectively, these data indicated that CXCL14 could serve as an OSCC inhibitor through suppressing PD-L1 and NF-κB signaling.
Figure 5. Expression levels of PD-L1, NF-κB, and p-IκB after overexpression of CXCL14 in TCA-8113 cells. The protein expression of PD-L1 (A) was measured by Western blot and the mRNA expression of PD-L1 (B) was assessed by qRT-PCR. (C) The protein expression of NF-κB and p-IκB were measured by Western blot. *P < 0.05, **P < 0.01, ***P < 0.001 versus NC. NC, negative control. All results were confirmed in at least three independent experiments.
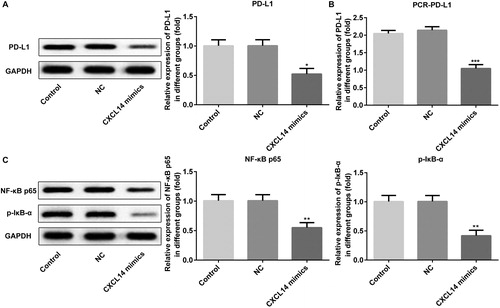
Conclusions
Based on our experimental results, we can draw the conclusion that CXCL14 overexpression was associated with significantly inhibited proliferation, invasion, migration and EMT in OSCC cells, potentially by regulating PD-L1 expression and NF-κB signaling. These findings indirectly indicated a novel tumor suppressive role of CXCL14 in the development of OSCC. If confirmed by direct evidence in future studies, CXCL14 may become an underlying therapeutic target in the treatment of OSCC.
Disclosure statement
The authors declare that they have no competing interests.
Funding
This study was supported by Jinling Hospital Management Project of Nanjing in China (2015018).
References
- Kulkarni P, Saxena U. Head and neck cancers, the neglected malignancies: present and future treatment strategies. Expert Opin Ther Targets. 2014;18(4):351–354.
- Hunter KD, Parkinson EK, Harrison PR. Profiling early head and neck cancer. Nat Rev Cancer. 2005;5(2):127–135.
- Leemans CR, Braakhuis BJ, Brakenhoff RH. The molecular biology of head and neck cancer. Nat Rev Cancer. 2011;11(1):9–22.
- Mucke T, Mitchell DA, Ritschl LM. Influence of tumor volume on survival in patients with oral squamous cell carcinoma. J Cancer Res Clin Oncol. 2015;141(6):1007–1011.
- Li Z, Liu J, Li L, et al. Epithelial mesenchymal transition induced by the CXCL9/CXCR3 axis through AKT activation promotes invasion and metastasis in tongue squamous cell carcinoma. Oncol Rep. 2018;39(3):1356–1368.
- Sleeman MA, Fraser JK, Murison JG, et al. B cell- and monocyte-activating chemokine (BMAC), a novel non-ELR alpha-chemokine. Int Immunol. 2000;12(5):677–689.
- Kiefer F, Siekmann AF. The role of chemokines and their receptors in angiogenesis. Cell Mol Life Sci. 2011;68(17):2811–2830.
- Mehrad B, Keane MP, Strieter RM. Chemokines as mediators of angiogenesis. Thromb Haemost. 2007;97(5):755–762.
- Maehata Y, Ozawa S, Kobayashi K, et al. Reactive oxygen species (ROS) reduce the expression of BRAK/CXCL14 in human head and neck squamous cell carcinoma cells. Free Radic Res. 2010;44(8):913–924.
- Gu XL, Ou ZL, Lin FJ, et al. Expression of CXCL14 and its anticancer role in breast cancer. Breast Cancer Res Treat. 2012;135(3):725–735.
- Shellenberger TD, Wang M, Gujrati M, et al. BRAK/CXCL14 is a potent inhibitor of angiogenesis and a chemotactic factor for immature dendritic cells. Cancer Res. 2004;64(22):8262–8270.
- Ghasri P, Admani S, Petelin A, et al. Treatment of actinic cheilitis using a 1,927-nm thulium fractional laser. Dermatol Surg. 2012;38(3):504–507.
- Quan J, Elhousiny M, Johnson NW, et al. Transforming growth factor-beta1 treatment of oral cancer induces epithelial-mesenchymal transition and promotes bone invasion via enhanced activity of osteoclasts. Clin Exp Metastasis. 2013;30(5):659–670.
- Creighton CJ, Chang JC, Rosen JM. Epithelial-mesenchymal transition (EMT) in tumor-initiating cells and its clinical implications in breast cancer. J Mammary Gland Biol Neoplasia. 2010;15(2):253–260.
- Kang Y, Massague J. Epithelial-mesenchymal transitions: twist in development and metastasis. Cell. 2004;118(3):277–279.
- Straub M, Drecoll E, Pfarr N, et al. CD274/PD-L1 gene amplification and PD-L1 protein expression are common events in squamous cell carcinoma of the oral cavity. Oncotarget. 2016;7(11):12024–12034.
- Weber M, Wehrhan F, Baran C, et al. PD-L1 expression in tumor tissue and peripheral blood of patients with oral squamous cell carcinoma. Oncotarget. 2017;8(68):112584–112597.
- Stasikowska-Kanicka O, Wągrowska-Danilewicz M, Danilewicz M. Immunohistochemical analysis of Foxp3+, CD4+, CD8+ cell infiltrates and PD-L1 in oral squamous cell carcinoma. Pathol Oncol Res. 2018;24(3):497–505.
- Chang P-H, Hwang-Verslues WW, Chang Y-C, et al. Activation of Robo1 signaling of breast cancer cells by Slit2 from stromal fibroblast restrains tumorigenesis via blocking PI3K/Akt/β-catenin pathway. Cancer Res. 2012;72(18):4652–4661.
- Livak KJ, Schmittgen TD. Analysis of relative gene expression data using real-time quantitative PCR and the 2(-Delta Delta C(T)) Method. Methods. 2001;25(4):402–408.
- Pu Y, Wang L, Wu H, et al. High MMP-21 expression in metastatic lymph nodes predicts unfavorable overall survival for oral squamous cell carcinoma patients with lymphatic metastasis. Oncol Rep. 2014;31(6):2644–2650.
- Faisal M, Abu Bakar M, Sarwar A, et al. Depth of invasion (DOI) as a predictor of cervical nodal metastasis and local recurrence in early stage squamous cell carcinoma of oral tongue (ESSCOT). PLos ONE. 2018;13(8):e0202632. [cited 2019 Jun 30].
- Ong HS, Gokavarapu S, Tian Z, et al. PDGFRA mRNA overexpression is associated with regional metastasis and reduced survival in oral squamous cell carcinoma. J Oral Pathol Med. 2018;47(7):652–659.
- Hata R. A new strategy to find targets for anticancer therapy: chemokine CXCL14/BRAK is a multifunctional tumor suppressor for head and neck squamous cell carcinoma. ISRN Otolaryngol. 2012;2012:1. [cited 2019 May 25];
- Ozawa S, Kato Y, Komori R, et al. BRAK/CXCL14 expression suppresses tumor growth in vivo in human oral carcinoma cells. Biochem Biophys Res Commun. 2006;348(2):406–412.
- Yang H, Pan L, Xu C, et al. Overexpression of tumor suppressor gene ZNF750 inhibits oral squamous cell carcinoma metastasis. Oncol Lett. 2017;14(5):5591–5596.
- Kim SE, Shin SH, Lee JY, et al. Resveratrol induces mitochondrial apoptosis and inhibits epithelial-mesenchymal transition in oral squamous cell carcinoma cells. Nutr Cancer. 2018;70(1):125–135.
- Krisanaprakornkit S, Iamaroon A. Epithelial-mesenchymal transition in oral squamous cell carcinoma. ISRN Oncol. 2012;2012:681469. [cited 2019 May 25];
- Velcheti V, Schalper KA, Carvajal DE, et al. Programmed death ligand-1 expression in non-small cell lung cancer. Lab Invest. 2014;94(1):107–116.
- Shi SJ, Wang LJ, Wang GD, et al. B7-H1 expression is associated with poor prognosis in colorectal carcinoma and regulates the proliferation and invasion of HCT116 colorectal cancer cells. PLoS ONE. 2013;8(10):e76012.
- Zhang P, Liu JZ, Li WL, et al. Lactoferricin B reverses cisplatin resistance in head and neck squamous cell carcinoma cells through targeting PD-L1. Cancer Med. 2018;7(7):3178–3187.
- Cao B, Yang Y, Pan Y, et al. Epigenetic silencing of CXCL14 induced colorectal cancer migration and invasion. Discov Med. 2013;16(88):137–147.
- Lu JC, Song GH, Tang QL, et al. IRX1 hypomethylation promotes osteosarcoma metastasis via induction of CXCL14/NF-kappa B signaling. J Clin Invest. 2015;125(5):1839–1856.